1 Introduction
Contemporary polymeric materials should be characterized by appropriate functional properties. The stability of a polymeric product is very important as it corresponds to the polymer's degradation capability in a given environment. Most commercial polymers are synthetic products characterized by a long decomposition time; some of these polymers are manufactured from the products of crude oil, the deposits of which will run out within a few dozen years. Therefore, products made of renewable raw materials arouse greater and greater interest. However, the biodegradation capability and non-toxicity are not the sole arguments for choosing these types of polymer; their specific properties are equally important [1–15].
Over the past decade, interest in polycaprolactone (PCL), a biodegradable polyester, has increased. The polymerization of ɛ-caprolactone proceeds according to a coordination mechanism with monomer ring opening. It is characterized by excellent biocompatibility with the human body and has therefore been widely used in medical products. The greatest interest in PCL was observed during the 1970–1980 decade, when it was primarily used as a drug carrier. Due to its good rheological and viscoelastic properties and therefore its ease of processing, PCL has an advantage over other polyesters [16–23].
In our study, we propose polymeric composites of epoxidized natural rubber (ENR) and PCL. Using the elasticity of rubber and strength of PCL, we created biodegradable composites with specific functional properties. However, both ENR and PCL are polymers that are relatively rapidly degraded in vivo. To improve the stability of these materials without loss of their ecological value, we used natural anti-aging substances, specifically flavonoids. The ENR/PCL composite, which exclusively contained natural antioxidants, can be widely applied to both commercial and more specific products [24–31].
2 Experimental
2.1 Reagents
The above compounds were supplied by Sigma–Aldrich GmbH. Their solutions were prepared with the following compounds.
The main subject of the study was polycaprolactone – Mn 70,000–90,000, < 1.0% water, 1.145 g/mL at 25 °C (Aldrich). Epoxidized natural rubber (Epoxyprene 50) was obtained from Kumpulan Guthrie Berhad, Malaysia. Dodecanoic acid obtained from Sigma–Aldrich was used as a cross-linking agent.
The anti-aging substances used included the following derivatives: morin hydrate (Fluka, 98%), rutin hydrate (Sigma, 95%), 4,5,7-trihydroxyflavone (Aldrich 98%), silymarin (Sigma), fiboflavin (Sigma ≥ 98%), curcumin from Curcuma longa (Sigma–Aldrich) and l-asparagine (Sigma–Aldrich ≥ 98%). Their efficiencies were compared against a commonly used HALS stabilizer Chimassorb81 (Ciba). Composite blends were prepared with the compositions shown in Table 1.
Polymer blend composition (PCL-polycaprolactone, ENR(50)-epoxidized natural rubber).
Composition | (phr) |
PCL | 40 |
ENR (50) | 60 |
Cross-linking agent (dodecanoic acid) | 1.0 |
Anti-aging substance | 1.25 |
2.1.1 Biological material
Hyphal fungi are the most effective organisms used in the biodegradation of plastics; therefore, they were used as test organisms in this study. The selection of mildew fungi is consistent with Standard ISO 846 (Dec. 2002 “Plastics. Assessment of the effects of microorganisms”). The following mildew fungi were used in the experiments: Aspergillus niger, Penicillium ochrochloron, Aspergillus terreus, Scopulariopsis brevicaulis, Aureobasidium pullulans, Trichoderma viride, Paecilomyces variotii and Chaetomium globosum. The strains were derived from the Collection of Pure Industrial Microbe Cultures of the Institute of Fermentation Technology and Microbiology (Lodz University of Technology). The mildew fungi strains were stored in a 5 °C Blg sweet-wort solution.
2.1.2 Nutrient media
The low-value nutrient medium consisted of 2.0 g of NaNO3, 0.7 g of KH2PO4, 0.3 g of K2HPO4, 0.5 g of KCl, 0.01 g of MgSO4·7H2O, 20 g of agar, and 1000 mL of water; its pH was between 6.0 and 6.5. The full-value nutrient medium had the same composition as above, with an additional 30 g of glucose.
2.2 Measurement methods
Rubber blends were prepared using a laboratory Brabender mixer with a front-roll rotation speed of Vp = 60 rpm and a friction of 1.1. The average temperature in the Brabender mixer was approximately 120 °C, and the mixing time was 30 min.
The rubber blends were vulcanized using steel vulcanization molds placed between the shelves of an electrically heated hydraulic press. Teflon films were used as spacers, preventing the adherence of blends to the press plates. Samples were vulcanized at 180 °C under 15 MPa of pressure for 60 min.
The density of cross-links in the vulcanizate network was determined by the method of equilibrium swelling, according to standard ISO 188. The vulcanizates were subjected to equilibrium swelling in toluene for 48 h at room temperature. The swollen samples were then weighed on a torsion balance and dried in a dryer at 60 °C until a constant weight was reached, and after 48 h, they were reweighed. The cross-linking density was determined on the basis of Flory–Rehner's equation as follows:
(1) |
The tensile strength of the vulcanizates was tested according to standard ISO 37 with a Zwick tester, model 1435, dumbbell w-3.
Aging characteristics were determined according to standard ISO 2578. Samples were subjected to the action of air at an elevated temperature (383 K) for 10 days in a dryer with thermo-circulation. UV aging was performed by means of a UV 2000 apparatus from Atlas. The tests lasted for 288 h and consisted of two alternately repeating segments: a day segment (radiation intensity 0.7 W/m2, temperature 60 °C, duration 8 h) and a night segment (no UV radiation, temperature 50 °C, duration 4 h). Climatic aging was performed using a Weather-Ometer (Atlas; Ci4000). The test was based on two variable segments simulating day and night conditions, and the samples were subjected to two different cycles. The daily cycle was characterized by a radiation intensity of 0.4 W/m2, 60 °C, 240-min duration and 80% humidity under rainwater; the night cycle was characterized by no radiation, 50 °C, 120-min duration and 60% humidity.
The aging coefficient was calculated as S = [TS′ × EB′]/[TS × EB], where TS = tensile strength, EB = elongation at break, and TS′, EB′ = the corresponding values after aging.
The dispersion of the co-agent in the elastomer matrix was estimated using scanning electron microscopy with a ZEISS SEM. The vulcanizates were broken apart in liquid nitrogen, and the surfaces of their fractures were examined. Prior to measuring, the samples were coated with carbon.
The color of the composites obtained was measured using a CM-3600d spectrophotometer (Konica Minolta). The radiation source consisted of four impulse xenon tubes. The spectral range of the apparatus was 360–740 nm, where the change in color dE × ab was calculated as follows:
(2) |
The CIE-LAB color scale is an approximately uniform color scale. In a uniform color scale, the differences between points plotted in the color space correspond to visual differences between the colors plotted. The CIE-LAB color space is organized in a cube form. The L* axis runs from top to bottom. The maximum for L* is 100, which represents a perfect reflecting diffuser. The minimum for L* is zero, which represents black. The a* and b* axes have no specific numerical limits. Positive a* is red. Negative a* is green. Positive b* is yellow. Negative b* is blue.
Oxygen induction time (OIT) tests were performed with a Mettler Toledo DSC instrument. Four-milligram samples were heated from room temperature to the test temperature, 220 °C, at a rate of 20 °C/min under a nitrogen atmosphere. After 5 min at 220 °C, the gas was changed from nitrogen to air at a flow rate of 60 mL/min. When all antioxidants were consumed, the sample began to oxidize, producing a deviation in the baseline. The OIT was measured as the time between the gas switch and an intersection with a tangent from the maximum derivate after oxidation had started. Two analyses of each sample were performed to ensure the accuracy of the results.
2.3 Investigating the effect of mildew fungi on rubber vulcanizates using the static method
2.3.1 The effect of mildew fungi on the material tested was assessed by two methods
Method A was used to test the natural resistance of the material when no other nutrient substance was present to determine whether the material tested was a source of food for the microorganisms. The material was disinfected with 75% ethanol and rinsed in sterile water. It was then placed on a nutrient medium without carbon, and a suspension of microorganisms was added (batch I). Simultaneously, batch S was prepared without microorganisms. The samples were incubated at 28 °C and RH 80% for five weeks. During incubation, the growth of microorganisms on the material surface was assessed.
Method B (+ variant B′) was used to determine the fungi static effect and the influence of surface soiling on the resistance of the material. Material samples were placed on a full-value nutrient medium followed by a uniform deposition of microorganism suspension. Samples were then incubated at 28 °C and RH 80% for five weeks. Afterward, the growth of microorganisms on both the nutrient medium and the sample was assessed by observing the appearance of the growth retardation zone around the samples.
2.4 Interpretation of the results (visual evaluation of fungi growth)
The samples were exposed to biodegradation, according to methods A and B, and were evaluated by means of a standard microscope and a stereoscopic microscope (magnification 60×), using an assessment scale in accordance with ISO 846. The final evaluations of the material studied were interpreted according to norm ISO 846 (Table 2). The test results were interpreted according to ISO 846 (Table 3).
Evaluation of the growth of microorganisms according to PN-EN ISO 846.
Method | Growth intensity | Evaluation of the test material |
A | 0 | The material is not a nutrient for microorganisms |
1 | The material contains substances that constitute a nutrient for microorganisms, or it is contaminated to a small extent that causes slight growth | |
2–5 | The material is not resistant to the action of microorganisms and contains substances that are nutrients for their growth | |
B + B′ | 0 | A strong fungistatic effect |
0 + inhibition zone | A strong fungistatic effect comprising the zone around the sample | |
1–5 | No fungistatic effect |
Evaluation of the growth of microorganisms according to PN-EN ISO 846.
Growth intensity | Rating |
0 | No visible growth under the microscope |
1 | A growth invisible to the naked eye, visible under the microscope |
2 | A growth visible to the naked eye, covering up to 25% of sample surface |
3 | A growth visible to the naked eye, covering up to 50% of sample surface |
4 | A considerable growth, covering more than 50% of sample surface |
5 | An intensive growth covering the total sample surface |
3 Results and discussion
The tensile strength (TS) of the ENR/PCL composites ranged from 14 to 18.4 MPa, and their elongation at break (EB) was from 206% to 309%. A reference sample of the composite had a TS = 15.5 MPa and an EB = 304%. The addition of flavonoids and other natural antioxidants to the ENR/PCL composites made no significant changes in their TS. Significant changes in the TS and EB of the ENR/PCL composites were only observed with the addition of Morin hydrate (TS = 18.4 MP and EB = 206%) and asparagine (TS = 15.0 MPa and EB = 206%). The increase in TS and the decrease in EB caused by the addition of these two compounds may be due to their activity during the cross-linking process. Asparagine has amine groups, and morin hydrate contains hydroxyl groups, both of which can react with the epoxy group in the rubber.
The commercial stabilizer, chemisorbed, decreased the TS of the ENR/PCL composite by 3.2 MPa when compared to the reference sample (Figs. 1 and 2).
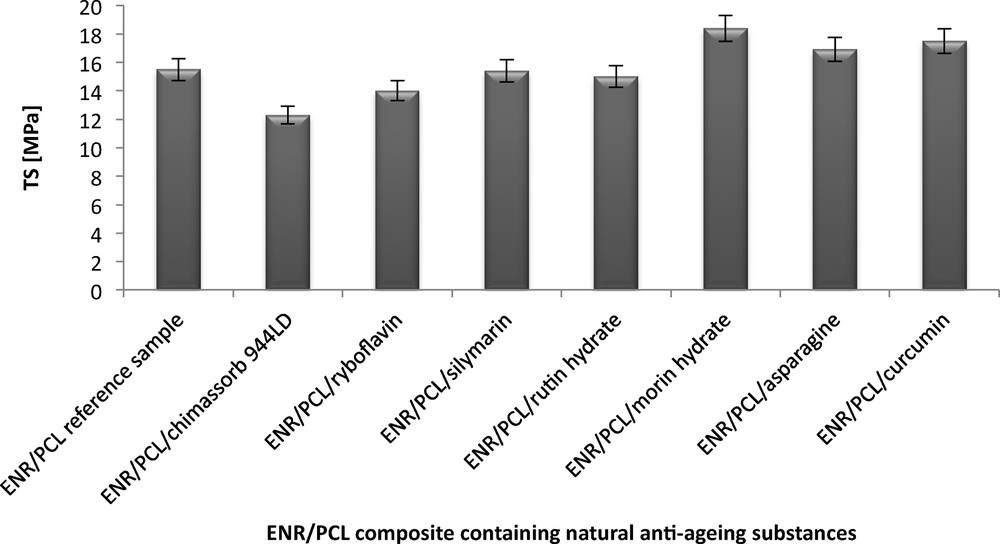
Tensile strength (TS) of the ENR/PCL (epoxidized natural rubber/polycaprolactone) composites containing natural anti-aging substances.
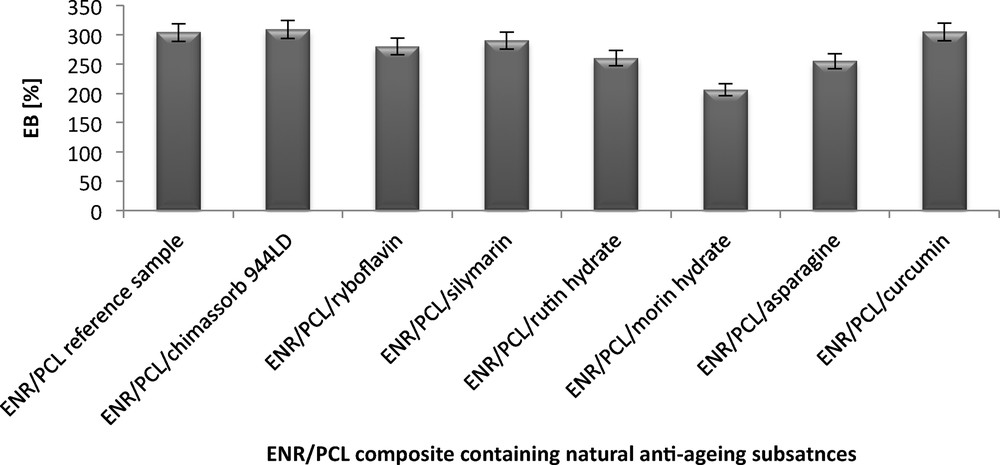
Elongation at the break (EB) of the ENR/PCL (epoxidized natural rubber/polycaprolactone) composites containing natural anti-aging substances.
The effect of morin hydrate and asparagine on the cross-linking of ENR rubber was also confirmed by the cross-link density of the ENR/PCL composites (Fig. 3). A reference sample of ENR/PCL showed a cross-link density of 14.6 × 10−5 mol·cm−3; the addition of hydrate increased νe by 11.1 × 10−5 mol·cm−3, and the addition of asparagine increased by 2.97 × 10−5 mol·cm−3. Both climatic and UV aging had no significant effect on the concentration of the network nodes of the ENR/PCL composites. After thermo-oxidative aging, most samples showed a slight increase in νe. The greatest increases, 9.57 × 10−5 mol·cm−3 and 6.92 × 10−5 mol·cm−3, were observed in the sample containing riboflavin and in the reference sample, respectively. The νe value for the silimaryn-containing composite increased by 5.15 × 10−5 mol·cm−3.

Density of the cross-links (ν) of the ENR/PCL (epoxidized natural rubber/polycaprolactone) composites containing natural anti-aging substances.
By analyzing the aging coefficients, which were calculated based on deformation energy before and after aging, we assessed the effect of the natural antioxidants on the stability of the ENR/PCL composites (Fig. 4). The coefficient of climatic, UV and thermo-oxidative aging of all the composites containing natural anti-aging substances were higher than those of the reference sample, confirming that the addition of polyphenols or amino acids results in increased aging resistance. Undoubtedly, the best anti-aging substances were morin hydrate, rutin hydrate and silymarin from the flavonoid group. The addition of curcumin and asparagine considerably improved the stability of the composites. It is worth noting that these substances increased the resistance of the ENR/PCL composites to climatic aging even at a high atmospheric humidity of almost 90%. This fact is important due to the high susceptibility of polycaprolactone to hydrolysis, which causes low stability in high humidity.
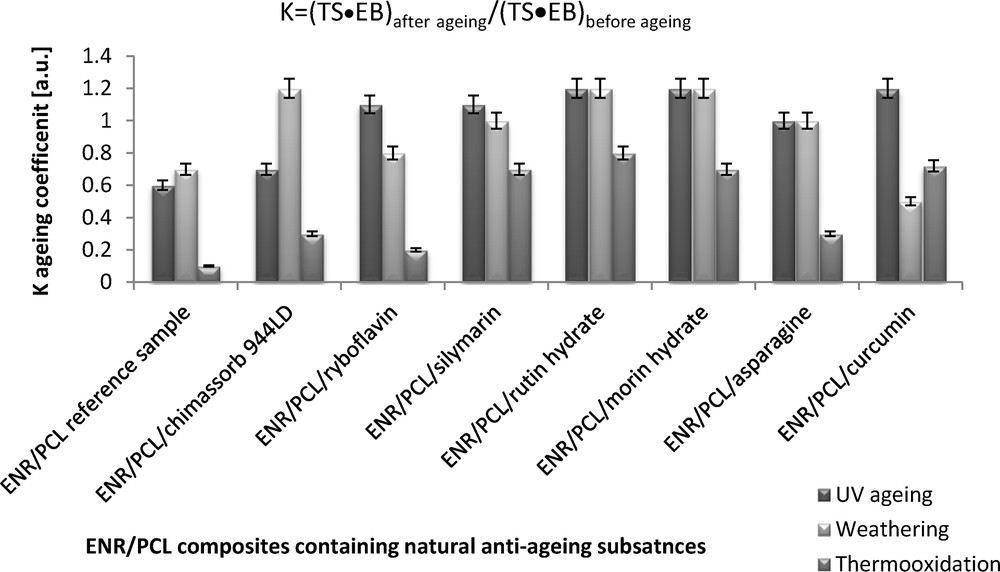
Aging coefficients (K) of the ENR/PCL (epoxidized natural rubber/polycaprolactone) composites containing natural anti-aging substances.
Because of the high pro-ecological value of the investigated ENR/PCL composites, we decided to examine their biodegradation capability. Using two methods as instructed in the standard, method A (with disinfection) and method B (without disinfection), we found that the composites were more biodegradable (a rating of 4 by method A and a rating of 5 by method B) than polycaprolactone alone (a rating of 0.1 by method A and a rating of 2 by method B). The addition of natural antioxidants, especially morin hydrate, only slightly influenced the susceptibility of the ENR/PCL composites to decomposition by mildew fungi, especially according to method A (Fig. 5 and Table 4).
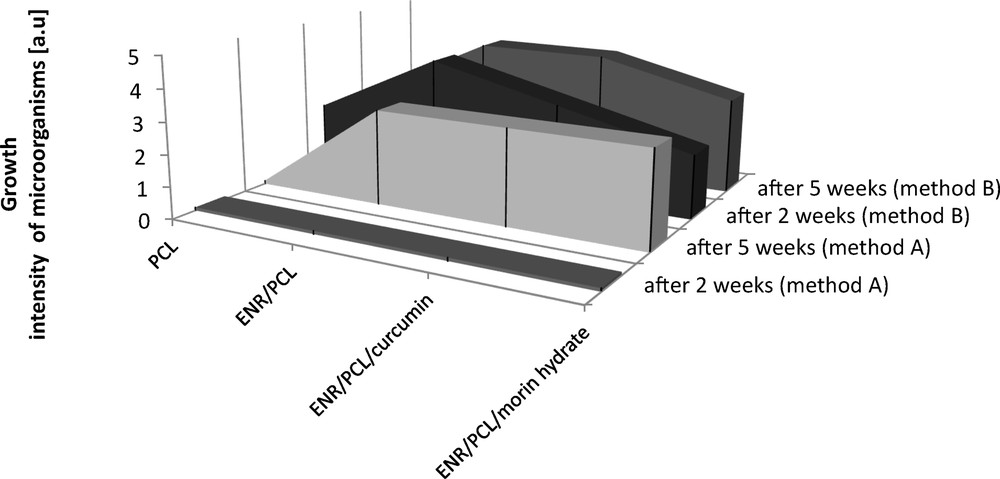
Assessment of the ENR/PCL (epoxidized natural rubber/polycaprolactone) composites containing natural anti-aging substances.
Photographs of ENR/PCL composites after biodegradation according to methods A and B. Method A: the material was disinfected with 75% ethanol and rinsed in sterile water. Method B: the sample was placed in chamber before the growth of the microorganisms.
Composite | Method A | Method B | ||
After 2 weeks | After 5 weeks | After 2 weeks | After 5 weeks | |
PCL | ||||
ENR/PCL | ||||
ENR/PCL/morin hydrate | ||||
ENR/PCL/curcumin |
The next stage in assessing the effect of natural antioxidants on the stability of ENR/PCL composites included a calorimetric test (Fig. 6). We used the stability of color as an indicator of sample susceptibility to degradation. The greatest changes in composite color were observed under the influence of climatic aging with high atmospheric humidity from rainfalls and solar radiation. As polycaprolactone itself shows a high capability to hydrolyze, it is of interest that the natural antioxidants increased the resistance of the composites to aging under extreme climatic conditions. This resistance was confirmed by the smaller color change estimated by means of the product differentia of parameters a and b (dE × ab) compared to those of the reference sample. For example, the ENR/PCL composite containing silymarin was characterized by a color change of dE × ab = 6.66, while the dE × ab of the reference sample was 18.39. The smallest color change was observed for composites containing antioxidants after UV aging; the ENR/PCL composite containing silymarin showed dE × ab = 5.41 compared to dE × ab = 19.65 for the reference sample. This fact indicates that the developed ENR/PCL composites containing anti-aging substances have good resistance to UV radiation; most anti-aging substances that are used have UV-absorbing properties. The greatest color changes were observed in both the ENR/PCL composites with antioxidants and the ENR/PCL reference sample.
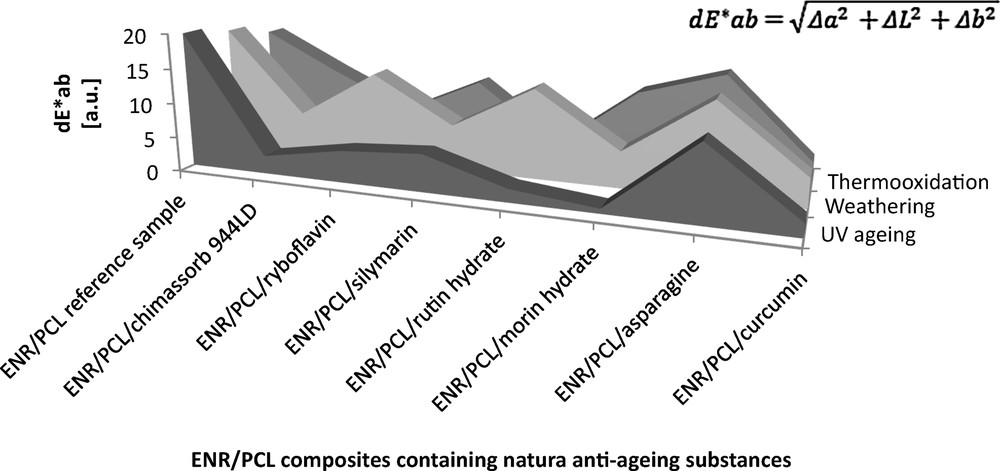
Color change due to aging (according to CIE-Lab system) of the ENR/PCL (epoxidized natural rubber/polycaprolactone) composites containing natural anti-aging substances.
Based on a DSC examination, we assessed the resistance of the ENR/PCL composites to oxidation. The oxidation induction time (OIT) test demonstrated that the best resistance to thermo-oxidation was shown by the ENR/PCL sample containing morin hydrate (OIT = 1.62 min) (Fig. 7). The reference sample of ENR vulcanizate showed a similar OIT (OIT = 1.36 min); however, in the case of this material, oxidation occurs naturally at a temperature of 210 °C. For PCL and ENR/PCL composites, oxidation was not recorded until 410 °C. Thus, the ENR/PCL composites are characterized by a higher thermal resistance to oxidation than ENR itself.

Oxidation induction time (OIT) of the ENR/PCL (epoxidized natural rubber/polycaprolactone) composites.
4 Conclusion
Polymers made of renewable raw materials are the most sought after pro-ecological materials, as opposed to the so-called petro-polymers. Unfortunately, their major drawback is that they are quite susceptible to degradation. It seems that the use of biodegradable polycaprolactone blends with natural rubber allows obtaining materials with special properties, such as an increased resistance to aging. Prolonging the use of such composites by adding natural anti-aging substances is an ecologically interesting solution for materials with a controlled exploitation time.
Certainly, the anti-aging substances used enhance the pro-ecological profile of the composites, making it possible to use them in products that must conform to restrictive standards. In this study, all substances that were used to develop the composites and polymers themselves are pro-ecological on account of their biodegradation capabilities and biocompatibilities. The addition of anti-aging substances derived from flavonoids and amino acids considerably improved the resistance of ENR/PCL composites to aging, especially under climatic conditions with variable air humidity and solar radiation. At the same time, our investigation results have confirmed that ENR/PCL composites are more biodegradable by selecting microorganism species than using polycaprolactone alone.
Acknowledgements
This study was supported by the Ministry of Science of Higher Education (0341/IP2/2011/71).