1 Introduction
Polymeric materials are widely used in various industrial branches, and the demand for them is continually growing; they are second after steel on the list of the most important material groups. It is assumed that in the 21st century, the amount of polymer used in industries may account for 50% of all materials [1–4]. Therefore, the long-term stability of polymer materials is of great importance. During their prolonged use, polymers continually interact with the environment. Their structure and properties change in time, and the range of these changes depends on the original polymer structure, as well as on the environment [5–7]. In other words, polymeric materials undergo ageing. There are two types of ageing that result in changes in the polymer properties: chemical and physical ageing. The process of polymer oxidation is responsible for the deterioration in their properties; hence, most stabilisers are antioxidants [6]. The industry currently uses stabilisers, such as amine derivatives with steric hindrance, synthetic polyphenols and phosphate derivatives that are not pro-ecological. Some portion of these additives migrates into the environment; therefore, it is important that the additives are both environment- and human life-friendly. According to the available literature, it seems that oxygen stabilisers of natural origin that show a high anti-oxidising activity may be worth investigating. A potential candidate may be one of the natural compounds, such as a derivative of gallic acid, that are well-known as perfect antioxidants. In this study, we investigate the use of lauryl gallate as a pro-ecological anti-ageing substance for common polymers with a wide range of use in everyday life. Undoubtedly, a positive influence of the additives used in polymers can considerably change the ecological profile of polymeric materials.
Lauryl gallate (dodecyl gallate) contains hydroxyl groups and a carboxyl group in its structure. It is one of the components of green tea. The healthful effects of green tea have been mainly ascribed to the capability of flavonol to capture free radicals by donating the phenolic hydrogen atom in the aromatic rings [7].
Gallic acid and its alkyl esters are natural compounds [8], used as additives to food products because of their anti-oxidising activity [9,10].
Alkyl gallates, which have a molecular structure composed of a hydrophilic head (phenolic ring) and a hydrophobic alkyl chain, have also been reported to have antifungal and antibacterial activity [11,12]. Though different studies have investigated the relation between the hydrophobic chain lengths and alkyl gallate properties, there is no clear consensus regarding the effect of the chain length on the antioxidant and antibacterial properties of gallates [13–17]. Several studies have reported the anti-carcinogenic effects of gallic acid and some of its derivatives in studies using animal models or human cell lines [18–21]. Kubo et al. [15] and Hossain et al. [11] showed that dodecyl gallate exhibits both potent chain breaking and preventive antioxidant activity. Dodecyl gallate prevents the generation of superoxide radicals by xanthine oxidase, inhibiting the enzyme. The hydrophobic dodecyl group is largely associated with the preventive antioxidant activity [10].
The electrochemical properties, anti-oxidising capability and the oxidation mechanism of organic compounds, such as flavonoids have been determined and assessed by electrochemical and spectrophotochemical methods [22–26]. Electroanalytical examinations by cyclic voltammetry and differential pulse voltammetry allow the precise determination of the electrochemical properties of the investigated compounds [27]. Electroanalytical measurements are used to determine and calculate some parameters of the compounds, such as half-wave potential, number of exchanged electrons, transition coefficient and rate constant of electrode reactions, which are important for the assessment of the anti-oxidising characteristics as well as for the recognition and understanding of the reaction mechanism of the compounds under investigation. The half-wave potential (E1/2) is an especially useful parameter providing information about the anti-oxidising activity of the compounds tested. It has been shown that flavonoids with less positive oxidation potential, i.e., higher susceptibility to electrochemical oxidation, possess higher radical scavenging activity [28,29].
2 Experimental
2.1 Reagents
The ethylene–octene elastomer (Engage) used in this study was from Mentedison Ferrara (Italy). Dicumyl peroxide (DCP, from Fluka) was used as the cross-linking agent, 1,3,5-triallyl-1,3,5-triazine-2,4,6(1H,3H,5H)-trione (Sigma–Aldrich Chemie GmbH) was the cross-linking co-agent and hexadecyltrimethylammonium bromide (CTAB, Sigma–Aldrich Chemie GmbH) was the dispersing agent. Areosil 380 silica (from Degussa) was used as a filler.
A 98% solution of lauryl gallate (Sigma–Aldrich) was the flavonoid derivative used as the anti-ageing substance.
Elastomer blends were prepared with the compositions shown in Table 1. Engage rubber was mixed with 2 phr CTAB. The time required for plasticising the rubber was 4 min. Then, 30 phr silica A380 and 1.5 phr lauryl gallate (antioxidant) were added directly to the rubber. Finally, DCP and the cross-linking co-agent were added. The total mixing time was 10 min at 40 °C.
Composition of Engage elastomer blends containing lauryll gallate.
Composition | M1 (phr) | M2 (phr) |
Engage | 100 | 100 |
DCP | 3.00 | 3.00 |
CTAB | 2.00 | 2.00 |
1,3,5-Triallyl-1,3,5-triazine-2,4,6(1H,3H,5H)-trione | 0.5 | 0.5 |
A380 | 30 | 30 |
Lauryll gallate | 1.5 |
The above compounds were supplied by Sigma-Aldrich GmbH. Solutions were also prepared with the following compounds:
- • acetonitrile (CH3CN) pure p.a. from POCh Gliwice, Poland;
- • tetrabutylammonium perchlorate (C4H9)4NClO4 from Fluka, used as the supporting electrolyte.
All the chemicals used in the experiments were analytical grade, and all the experiments were performed at room temperature.
2.2 Biological material
Hyphal fungi are the most effective organisms in the biodegradation of plastics and therefore were used as the test organisms. The selection of mildew fungi is consistent with Standard PN-EN ISO 846 (Dec. 2002 “Plastics. Assessment of the effects of microorganisms.”). The following mildew fungi were used in the experiments: Aspergillus niger, Penicillium ochrochloron, Aspergillus terreus, Scopulariopsis brevicaulis, Aureobasidium pullulans, Trichoderma viride, Paecilomyces variotii, and Chaetomium globosum. The strains were derived from the Collection of Pure Industrial Microbe Cultures of the Institute of Fermentation Technology and Microbiology (Lodz University of Technology, Poland). The mildew fungi strains were stored in a 5oBlg sweet-wort solution.
2.3 Nutrient media
The low-value nutrient medium consisted of 2.0 g of NaNO3, 0.7 g of KH2PO4, 0.3 g of K2HPO4, 0.5 g of KCl, 0.01 g of MgSO4·7H2O, 20 g of agar, and 1000 mL of water. The pH was 6.0–6.5. The full-value nutrient medium had the same composition as above, plus 30 g of glucose.
2.4 Measurement methods
To assess the mechanism and kinetics of the electrochemical oxidation of the compounds under investigation, cyclic voltammetry (CV) and differential pulse (DPV) methods were used, employing an Autolab analytical unit (Ecochemie, Holland). A three-electrode system was used for the measurements with platinum test and auxiliary electrodes. The potential of the tested electrode was measured in relation to a ferrocene reference electrode (Fc+/Fc), whose standard potential is defined as zero, independently of the solvent used. Prior to taking any measurement, all the solutions were deoxidised with argon. During the measurements, an argon atmosphere was maintained over the solution. The effect of the polarisation rate on the electrooxidation of dodecyl gallate in an anhydrous medium was assessed.
Rubber blends were prepared by means of a laboratory mixing mill with rolls of the following dimensions: length, L = 330 mm, and diameter, D = 140 mm. The speed of rotation of the front roll was Vp = 20 rpm, the coefficient of friction was 1.1 and the average temperature of the rolls was approximately 40 °C.
The vulcanisation of rubber blends was carried out with the use of steel vulcanisation moulds placed between the shelves of an electrically heated hydraulic press. Teflon films were used as spacers preventing the adherence of blends to the press plates. The samples were vulcanised at 160 °C under a pressure of 15 MPa for 30 min.
The density of crosslinks in the vulcanisate network was determined by the method of equilibrium swelling, according to standard PN-74/C-04236. The vulcanisates were subjected to equilibrium swelling in toluene for 48 h at room temperature. The swollen samples were then weighed on a torsion balance and dried in a dryer at 60 °C to a constant weight. After 48 h, they were reweighed. The cross-linking density was determined on the basis of Flory–Rehner's equation as follows:
(1) |
The tensile strength of the vulcanisates was tested according to standard PN-ISO 37:1998 by means of a ZWICK tester, model 1435, for dumbbell w-3.
Ageing characteristics were determined according to standard PN-82/C-04216. Samples were subjected to the action of air at an elevated temperature (383 K) for 10 days in a dryer with thermocirculation. UV ageing was performed by means of an UV 2000 apparatus from Atlas. The test lasted for 288 h and consisted of two alternately repeating segments: a daily segment (radiation intensity 0.7 W/m2, temperature 60 °C, duration 8 h) and a night segment (no UV radiation, temperature 50 °C, duration 4 h). Climatic ageing was carried out using a Weather-Ometer (Atlas; Ci 4000). The test was based on two variable segments simulating day and night conditions, and the samples were subjected to two different cycles. The daily cycle was characterised by a radiation intensity of 0.4 W/m2, 60 °C, 240 min duration, and 80% humidity under rainwater; the night cycle was characterised by no radiation, 50 °C, 120 min duration and 60% humidity.
The ageing coefficient was calculated using S = [TS′ × EB′]/[TS × EB], where TS = tensile strength, EB = elongation at break, and TS′, EB′ = the corresponding values after ageing.
The dispersion of the co-agent in the elastomer matrix was estimated using scanning electron microscopy with a ZEISS SEM. The vulcanisates were broken apart in liquid nitrogen, and the surfaces of their fractures were examined. Prior to measuring, the samples were coated with carbon.
The oxygen induction time (OIT) tests were performed with a Mettler Toledo DSC instrument. Four-milligram samples were heated from room temperature to the test temperature, 220 °C, at a rate of 20 °C/min under nitrogen atmosphere. After 5 min at 220 °C, the gas was changed from nitrogen to air at a flow rate of 60 mL/min. When all antioxidants were consumed, the sample started to oxidise, producing a deviation in the baseline. The OIT was measured as the time between the gas switch and an intersection with a tangent from the maximum derivate after oxidation has started. Two analyses on each sample were performed to ensure the accuracy of the result.
The thermal decomposition of lauryl gallate was carried out in a differential scanning calorimeter DSC1 (Mettler Toledo). Before measurements, the apparatus was calibrated on the basis of the following standards: the temperature scale on the basis of n-octane and indium and the heat exchange according to the fusion heat of indium, 28.45 J/mg. The process conditions were measurement temperatures ranging from –150–500 °C, 20 cm3/min nitrogen flow rate, and 10 °C/min heating rate.
2.4.1 Investigating the effect of mildew fungi on rubber vulcanisates by the static method
The effect of mildew fungi on the material tested was assessed by two methods:
- • method A was used for testing the natural resistance of material in the case when there is no other nutrient substance to check whether the material tested is a source of food for microorganisms. The material was disinfected with 75% ethanol and rinsed in sterile water. The material sample to be tested was placed on a nutrient medium without carbon followed by the deposition of a suspension of microorganisms (batch I). At the same time, batch S was prepared without microorganisms. The samples were incubated at a temperature of 28 °C and RH 80% for 5 weeks. During incubation, the growth of microorganisms on the material surface was assessed;
- • method B (+ variant B′) was used to determine the fungi static effect and the influence of surface soiling on the resistance of the material tested. The material samples were placed on a full-value nutrient medium; then a uniform suspension of microorganisms was uniformly deposited. The samples were then incubated at a temperature of 28 °C and RH 80% for 5 weeks. Afterward, the growth in microorganisms on the nutrient medium and the sample was assessed by observing the appearance of the growth retardation zone around the samples. A variant B′ was also used, in which the suspension of mildew was sown on the full-value nutrient medium and incubated until it was fungi-grown, and then samples of the material to be tested were placed on it. The samples were incubated at 28 °C and RH 80% for four weeks. Afterwards, the growth in microorganisms on the nutrient medium and the sample were assessed by observing the appearance of the growth retardation zone around the samples.
2.4.2 Interpretation of the results (visual evaluation of fungi growth)
The samples exposed to biodegradation according to methods A and B were evaluated by means of a standard microscope and a stereoscopic microscope (magnification 60 ×), using an assessment scale in accordance with PN-EN ISO 846 (Table 2). The final evaluations of the studied material were interpreted according to the latter.
Evaluation of the test materials according to PN-EN ISO 846.
Method | Growth intensity | Evaluation of the test material |
A | 0 | The material is not a nutrient for microorganisms |
1 | The material contains substances that constitute a nutrient for microorganisms, or it is contaminated to a small extent, which causes a slight growth | |
2–5 | The material is not resistant to the action of microorganisms and contains substances that are nutrients for their growth | |
B + B′ | 0 | A strong fungistatic effect |
0 + Inhibition zone | A strong fungistatic effect comprising the zone around the sample | |
1 to 5 | No fungistatic effect |
3 Results and discussion
3.1 Electrochemical behaviour of dodecyl gallate
The structure of lauryl gallate includes hydroxyl groups and a carboxyl group, and it is a component of green tea. These features are primarily used in the nutrition industry. The current activity is characterised by the effect of the electrochemical reaction on the potential of the test electrodes. Examples of cyclic voltammograms and differential pulse voltammograms of lauryl gallate electrooxidation are shown in Fig. 1. The potential or the half-wave potential of the test electrode is determined from the peak of the differential pulse voltammograms.
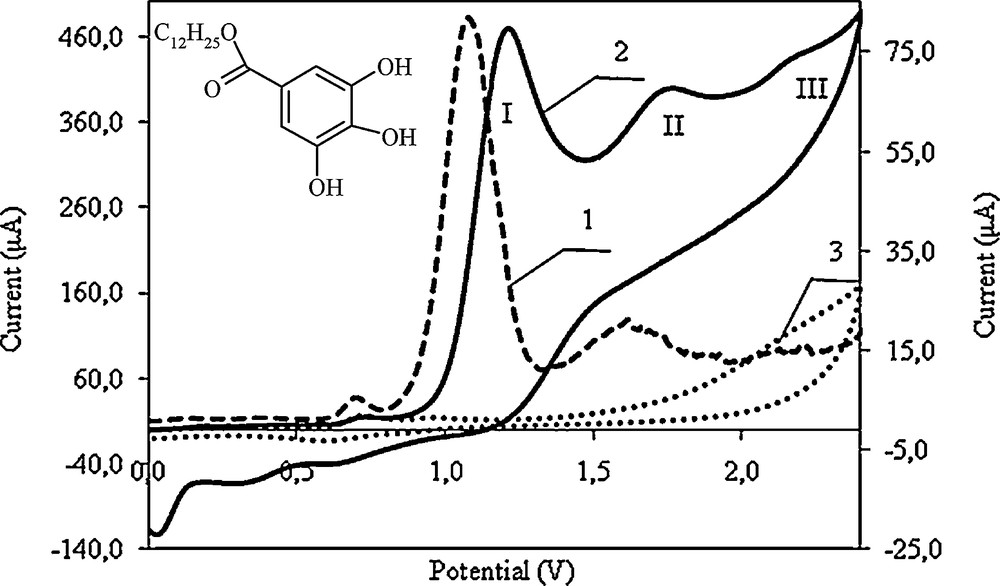
Voltammograms of dodecyl gallate electrooxidation of Pt electrode: 1. Differential pulse voltammogram, 2. Cyclic voltammogram, 3. Cyclic voltammogram recorded in the supporting electrolyte; c = 2.0 × 10−3 mol/L in 0.1 mol/L (C4H9)4NClO4 in acetonitrile, v = 0.1 V/s.
Within the potential range where the compound oxidation peaks appear, the supporting electrolyte (tetrabutylammonium perchlorate in acetonitrile at 0.1 mol/L) shows no characteristic peaks, but charges the electrical double layer (Fig. 1, curve 3). However, a small wave appears in the supporting electrolyte in the potential range from 0.4 to 1.0 V in the voltammograms. This wave can be attributed to the presence of (C4H9)4NClO4 and to the oxidation of perchlorate ions. The current of this wave is low compared to peak currents attributed to the oxidation of the primary compounds. Zieja et al. [30] reported that this wave can also be caused by the oxidation of impurities, such as water and other organic substances.
Dodecyl gallate is oxidised using at least three electrode steps at potentials lower than the potential of the decomposition of the electrolyte (Fig. 1, curves 1 and 2). The occurring electrode reactions are irremediable. The half-wave potential (E1/2) of the first step of dodecyl gallate oxidation, as determined by cyclic voltammetry, is 1.12 V; this value corresponds to the peak potential from the differential pulse voltammetry measurements. The half-wave potentials (E1/2) of the second and third steps are 1.68 V and 2.12 V, respectively.
3.2 Influence of scan rate
The influence of the speed of polarisation on the electrooxidation of dodecyl gallate in the range from 0.010 to 0.500 V/s was examined by using a cyclic voltammogram (Fig. 2). With the increase in the rate of polarization, potentials of dodecyl gallate electrooxidation slightly shifts towards more positive values, and the peak currents grow. In the first phase of electrooxidation, the potential of the spade and the electricity of the spade were examined; however, for the second and the third stages of the reaction, only the potential of the spade was examined.
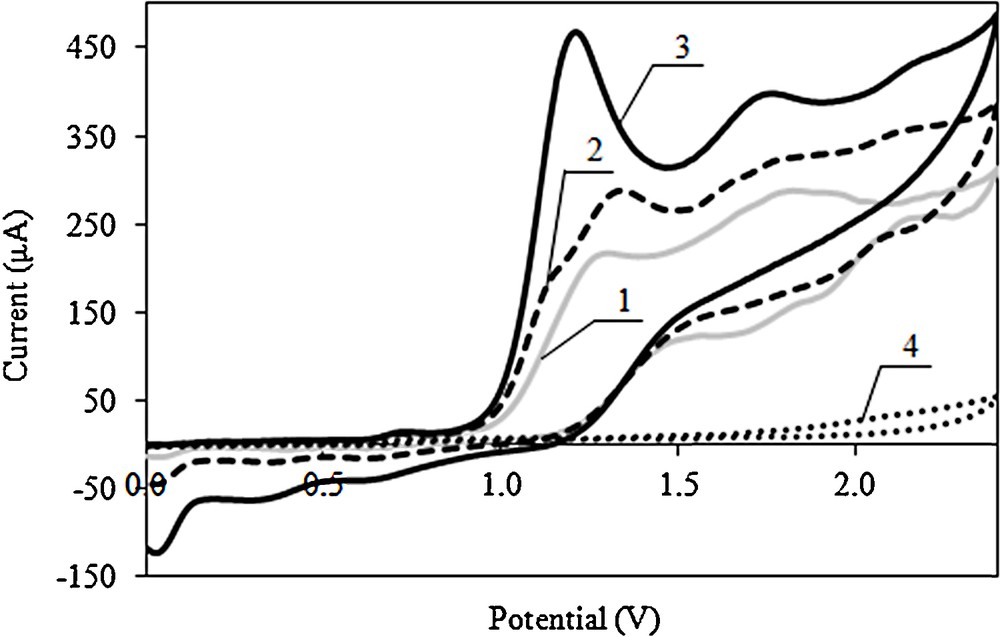
Cyclic voltammograms of dodecyl gallate oxidation of Pt electrode; c = 2 × 10−3 mol/L in 0.1 mol/L (C4H9)4NClO4 in acetonitrile at various scan rates; curve 1: 0.01 V/s, 2: 0.02 V/s, 3: 0.1 V/s, 4: cyclic voltammogram of the supporting electrolyte (0.1 mol/L (C4H9)4NClO4 in acetonitrile).
The scan rate is one of the parameters significantly affecting the electrooxidation of various compounds. Thus, the effect of the scan rate on dodecyl gallate electrooxidation was investigated in the range from 0.01 to 0.5 V/s using cyclic voltammetry (Fig. 2).
Cyclic voltammograms were used in the determination of peak current and potential for the dodecyl gallate electrooxidation. Two approaches widely used to study the reversibility of the reactions and to determine whether a reaction is adsorption- or diffusion-controlled consist of dependence analyses: ip on v1/2 and log ip on log v. Fig. 3 shows the plots for the oxidation peak of dodecyl gallate in 0.1 mol/L (C4H9)4NClO4 in acetonitrile. For reversible or irreversible systems without kinetic complications, ip varies linearly with v1/2, intercepting the origin. However, the plot of ip vs. v1/2 deviates from linearity and presents a value different from zero for the linear coefficient, if the electrode process is preceded or followed by a homogeneous chemical reaction.
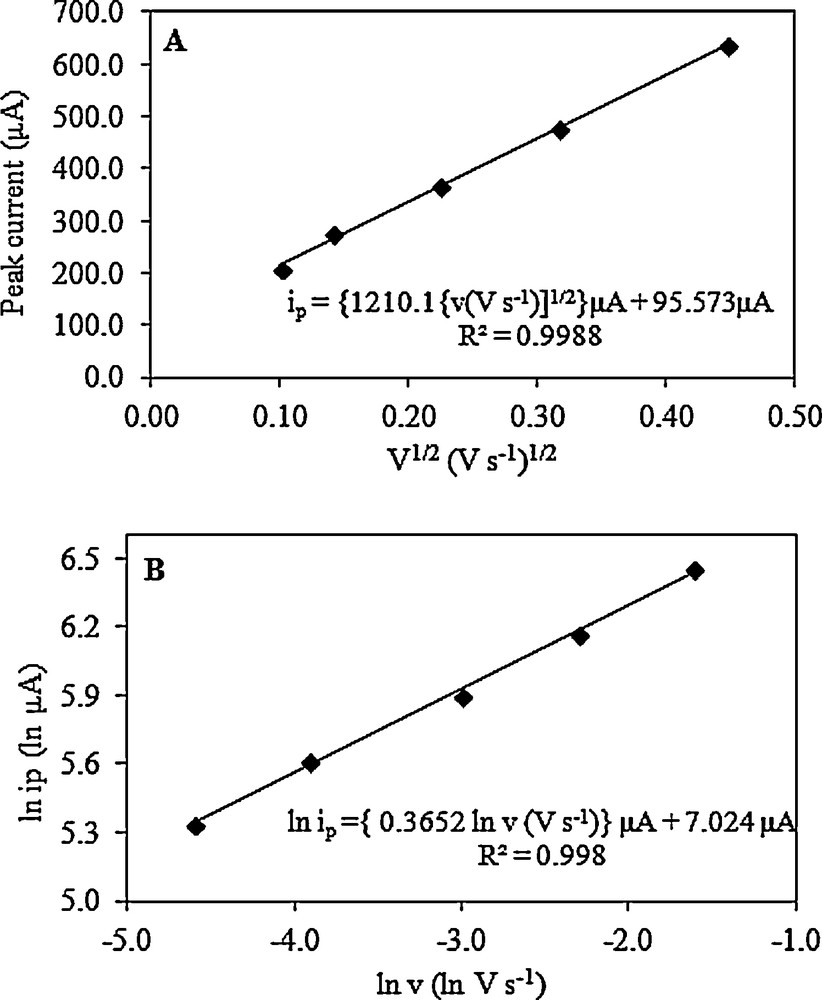
A. Dependence of the anodic peak current (ip) on the square root of the potential scan rate (v) for the oxidation of dodecyl gallate in 0.1 mol/L (C4H9)4NClO4 in acetonitrile on a Pt electrode. B. Dependence of the anodic peak current on the potential scan rate in double logarithm coordinates for the oxidation of dodecyl gallate in 0.1 mol/L (C4H9)4NClO4 in acetonitrile on a Pt electrode.
In the scan rate ranges from 0.01 to 0.5 V/s, the peak current of dodecyl gallate electrooxidation depends linearly on the square root of the scan rate and is described by the following equation:
This dependence does not cross the origin (Fig. 3A). This suggests that the electrode process of dodecyl gallate electrooxidation is controlled by diffusion and can be preceded by a chemical reaction. In contrast, the dependence of ln ip on ln v is linear (Fig. 3B) and is described by the following equation:
The slope is 0.37, indicating a diffusion-controlled electrode process. A slope close to 0.5 is expected for diffusion-controlled electrode processes, whereas a slope close to 1.0 is expected for adsorption-controlled processes [31–33].
3.3 Kinetic parameters of dodecyl gallate electrooxidation
The voltammograms recorded under linear diffusion of the first step of the electrooxidation were used to determine the peak potential (Epa), the half-peak potential (Epa/2), and the half-wave potential (E1/2). Additionally, the voltammograms were used to calculate an anodic transition coefficient (βnβ) and a heterogeneous rate constant (kbh) of the electrode process for the half-wave potential [34]. The parameters were calculated from the following equations:
(2) |
(3) |
(4) |
F = Faraday constant (96,487 C/mol),
R = universal gas constant (8.314 J/K/mol), and
T = temperature (K).
The diffusion coefficient was estimated according to Hayduk and Laudie's equation [35] as follows:
(5) |
The calculated value of the diffusion coefficient is 4.36 × 10−6 cm2/s.
The half-wave potential of step I (E1/2) is 1.12 V and that of electrode step II is 1.07 V. The calculated anode transition coefficient (βnβ) is 0.64, and the heterogeneous rate constant of step I of dodecyl gallate electrooxidation at the half-wave potential (kbh) is 7.02 × 10−4 cm/s.
3.4 Dodecyl gallate oxidation processes
Quantum chemical parameters are required to determine reactivity and energy potentials. There is no research available on the positions of the electron load or the reactivity of the individual sites (Fig. 4) [33]. The highest occupied energy calculated (EHOMO = potential ionisation) of orbital EHOMO, is a measure of the facility of giving away electrons and, thus, indicates a more receptive or oxidising site. Calculated totals of the highest occupied molecular energy of orbital EHOMO = –9.14 eV. High electron density in the dodecyl gallate is in the benzene ring, on the carbon atoms connected with the hydroxyl groups, suggesting the ease of oxidising hydroxyl groups. The mechanism of dodecyl gallate oxidation in the electrode next stages and antioxidant activity is connected with the quantity of hydroxyl groups in the aromatic nucleus of this connection. On the basis of electroanalytical studies and data in the literature, it is possible to suggest the mechanism of dodecyl gallate oxidation (lauryl gallate) (Scheme 1) [25].

Electron density and probable sites in dodecyl gallate molecules that are susceptible to electrooxidation.

3.5 Effect of dodecyl gallate on the cross-linking density of Engage vulcanisates
The addition of natural antioxidants to elastomeric materials is intended to improve their stability. However, it is important that the additives exert no influence on the deterioration in polymer processing conditions and the properties of the final products. The natural antioxidant used in this study belongs to a group of polyphenols. The mechanism of its action in oxidation processes consists in scavenging the free radicals generated by the initiation of the oxidation reactions. The mechanism of peroxide cross-linking is based on redox reactions; so the peroxide radicals participating in cross-linking can sometimes react with the antioxidant used, which can be manifested by a change in the cross-linking density, compared to that of a standard vulcanisate. Figs. 5 and 6 show the effect of degradation factors, such as UV radiation, climatic conditions or thermo-oxidative ageing, on changes in the cross-link density. During the first 48 h of ageing, the greatest changes in ve are visible in the case of thermo-oxidative ageing and UV-induced ageing, and after 96 h, a considerable decrease in the concentration of network nodes under the influence of the synergistic action of climatic conditions is observed. Smaller changes are visible in the case of other types of ageing. After 96 h of ageing of Engage vulcanisates with UV radiation, climatic conditions or thermal treatment, changes in the cross-link density are constant, and changes in ve are within the error limits. In summary, the cross-link density of the Engage rubber vulcanisates changes during ageing. In contrast, the standard vulcanisate Engage, under the influence of climatic ageing, shows only slight changes, including only an increase in the concentration of network nodes in the vulcanisate. It is most probable that the action of elevated temperature and radiation resulted in the cross-linking of ethylene groups present in the ethylene structure. The vulcanisate cross-link density linearly decreased during the action of degradation factors.
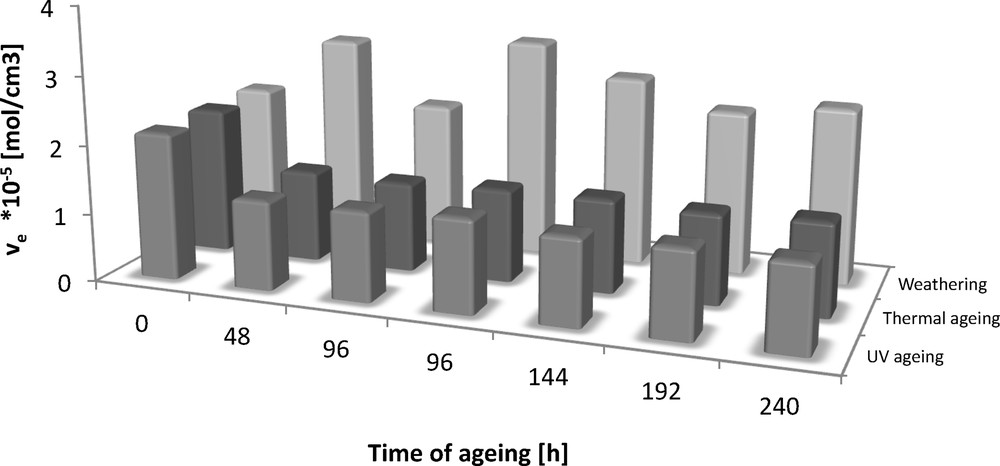
Effect of ageing on the cross-linking density of Engage vulcanizates (reference sample).

Effect of ageing on the cross-linking density of Engage vulcanizates with dodecyl gallate.
3.6 Effect of dodecyl gallate on the stabilisation of Engage vulcanisates after ageing
The thermal stability of polymers is of great importance during their processing. Additionally, each additive should be stable at its cross-linking temperature or during the preparation of polymeric blends. Therefore, samples of dodecyl gallate were examined using thermogravimetric analysis to determine their thermal stability. Its thermogravimetric curve shows an endothermic peak at approximately 100 °C, explained by dehydroxylation accompanied by melting. The decomposition of a 2% gallate mass is not noticeable before the temperature reaches 290 °C, while a 50% loss in weight was observed at 353 °C. Thus, it may be assumed that the antioxidant will be stable during the processing of the polymeric materials. Gallate is less thermally stable than the commercial stabiliser of the HALS type used in industry, with a mass loss of 50% at 465 °C, but it is still stable enough to be used in polymer processing (Fig. 7).
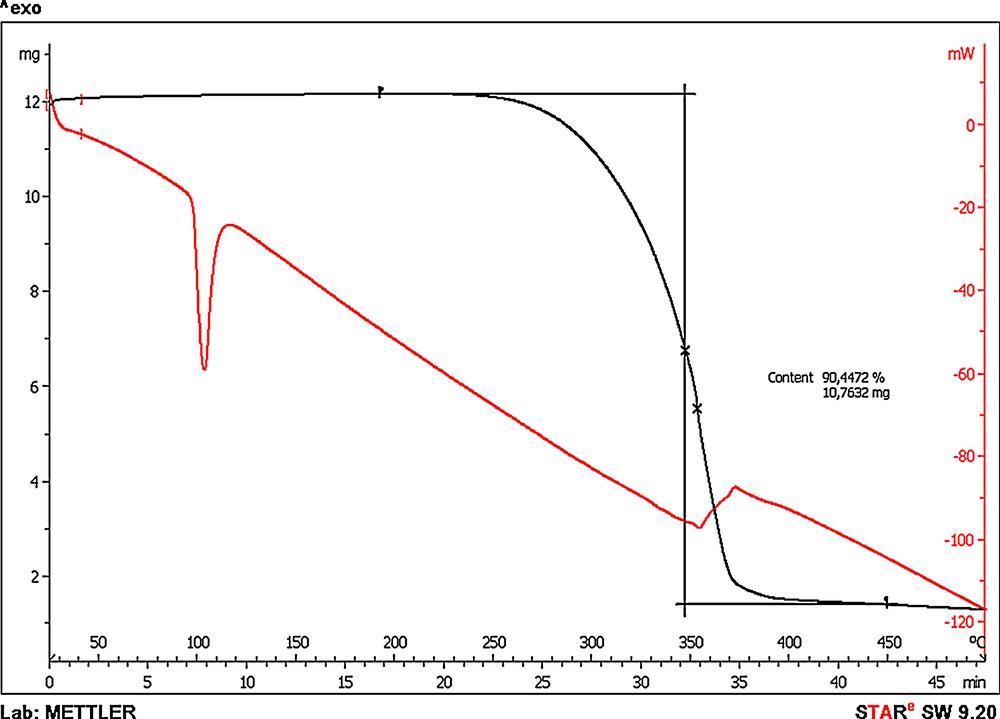
(Color online.) DSC (differential scanning calorimetry) curve of dodecyl gallate.
The addition of dodecyl gallate to the ethylene–octene rubber resulted in a decrease in tensile strength of 3 MPa and an elongation of 50% (Figs. 8 and 9). The tensile strengths of both the vulcanisate sample containing lauryl gallate and the Engage standard sample linearly decreased during the action of the degradation factors. However, it seems that the tensile strength decreasing effect changes as a function of ageing time. The stability of Engage vulcanisate was also improved after 288 h of ageing. The tensile strength of the vulcanisate containing dodecyl gallate decreased to 5 MPa, while the vulcanisate with no antioxidant was totally degraded after 244 h of UV ageing as well as after climatic and thermo-oxidative ageing (Fig. 9). The most destructive action was observed after the thermal and UV ageing processes for both samples of Engage vulcanisates. However, the changes in the mechanical properties of the sample stabilised with dodecyl gallate were considerably smaller than those in the case of the reference sample. The elongation at break (Figs. 10 and 11) for the sample containing the antioxidant versus the duration of UV, climatic and thermal ageing processes changed linearly by a maximum of 100%, but that of the reference sample changed by amounts up to 300%. The ageing coefficients (Figs. 12 and 13) calculated on the basis of changes in the deformation energy after ageing, in relation to that before ageing, also confirm the improvement in the resistance of Engage vulcanisates to ageing due to the addition of dodecyl gallate.
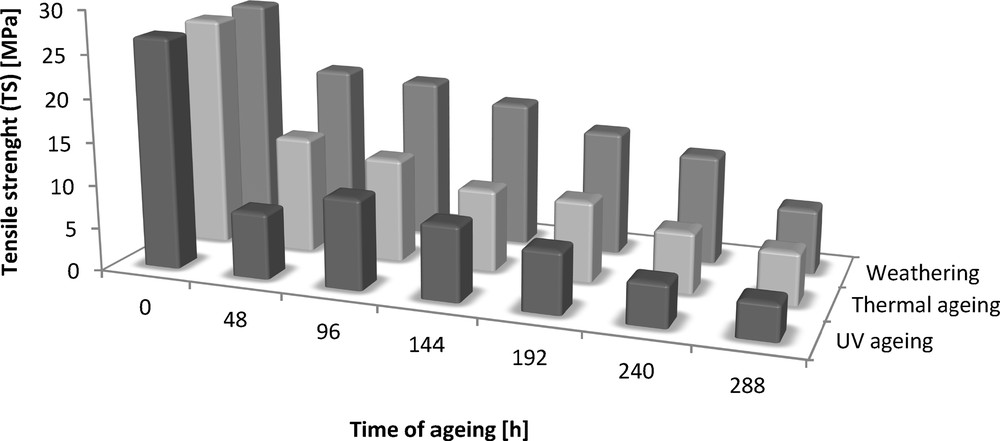
Tensile strength (TS) of the Engage vulcanizates subjected to ageing.
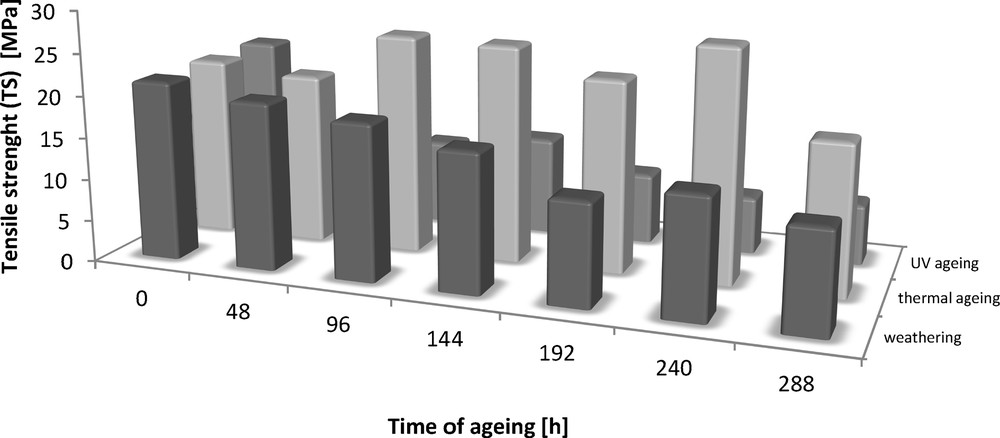
Tensile strength (TS) of the Engage vulcanizates containing dodecyl gallate subjected to ageing.
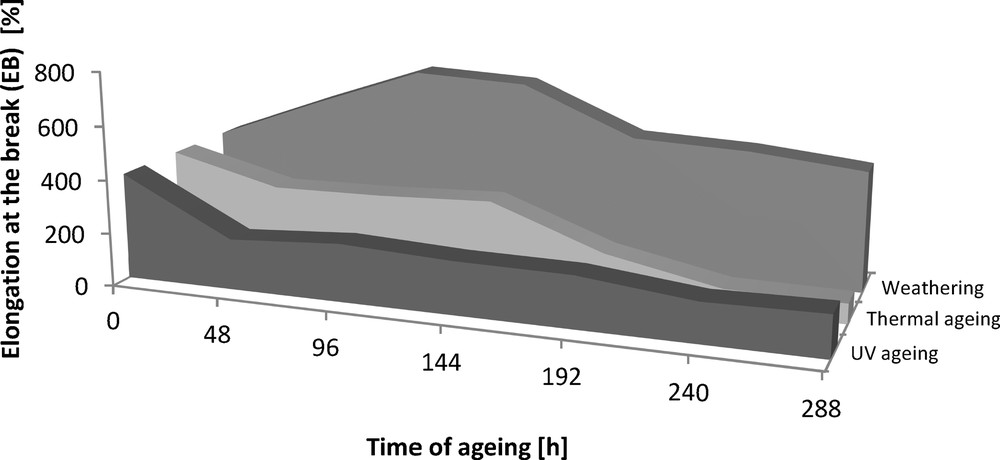
Elongation at the break (EB) of the Engage vulcanizates subjected to ageing.
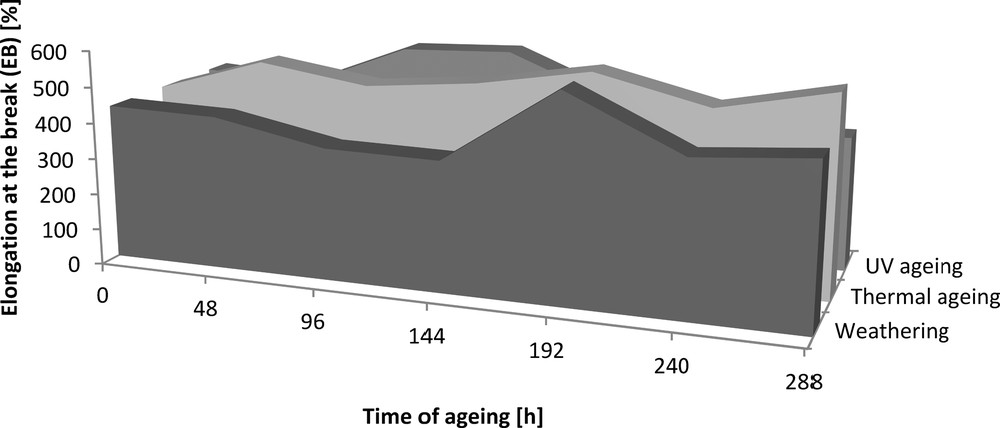
Elongation at the break (EB) of the Engage vulcanizates containing dodecyl gallate subjected to ageing.

Ageing coefficient (K) of Engage vulcanizates.
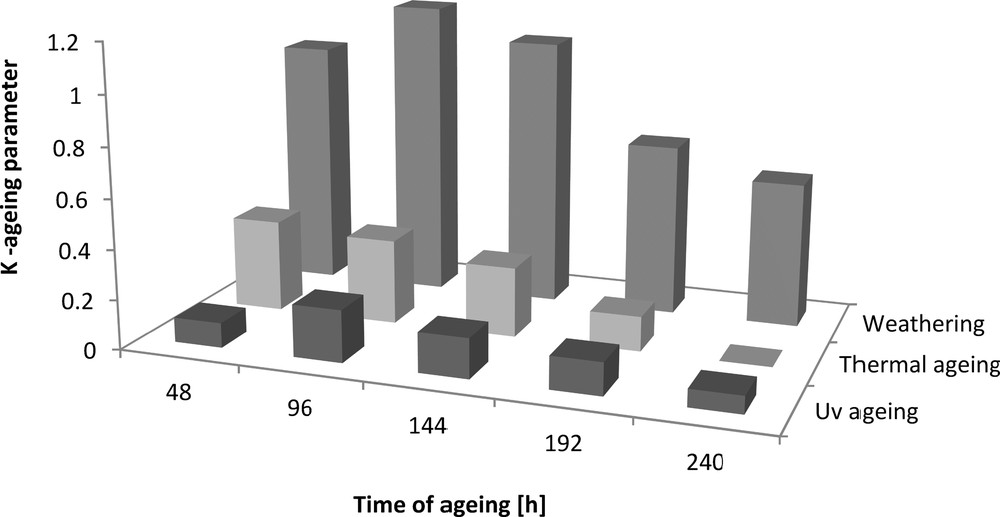
Ageing coefficient (K) of Engage vulcanizates containing dodecyl gallate.
The stabilising effect of dodecyl gallate on ethylene–octene rubber is validated by the oxygen index (OIT) values determined by the thermogravimetric method (Fig. 14). The oxygen induction time of Engage vulcanisation was 62.94 min and that of the reference sample was only 2.88 min. Thus, a visible stabilisation effect has been obtained when a natural antioxidant prolongs the oxygen induction by as much as 60.06 min, as illustrated by the exothermal peak in the thermogravimetric curve, determined at the temperature of the Engage vulcanisate oxidation (240 °C). Commercial stabilisers prolong OIT time only by several minutes. Moreover, the addition of gallate decreases the oxidation energy of Engage vulcanisate from 4848 J/g for the reference sample to 1651 J/g for the sample containing the antioxidant (Fig. 14).
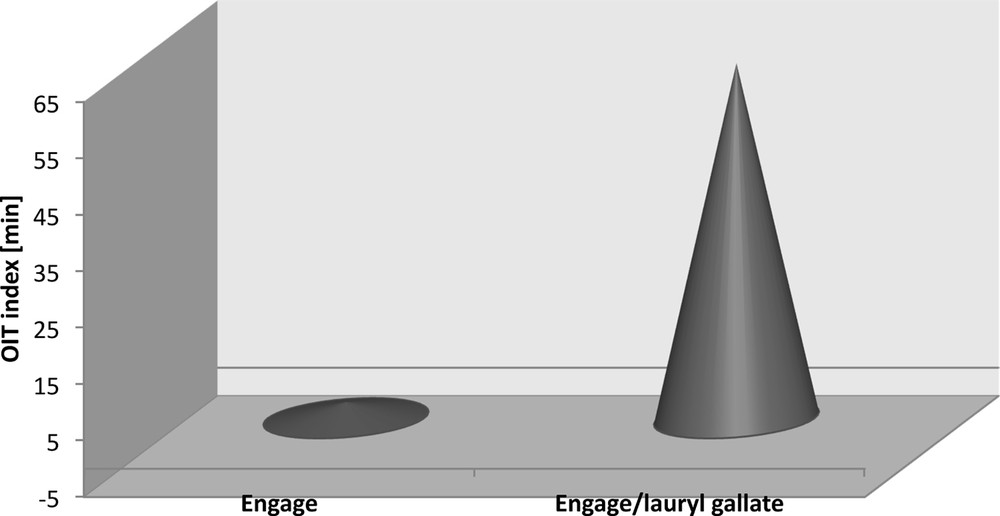
OIT index of the Engage vulcanizates measurement by the TG method.
The effect of the stabiliser on the biodeterioration of Engage vulcanisates was determined using two methods: method A (Table 3), in which the polymeric material was the only nutrient for microorganisms, and method B (Table 4), in which the polymeric material with its impurities was used. From the test results, in the case when only a disinfected sample is used as the nutrient for the mildew fungi, the susceptibility of the elastomeric material to biodegradation is increased (decomposition degree 2–3) compared to the case when the reference sample (decomposition degree 1). A similar tendency is observed in the case of methods B and B′. When samples are assumed to contain impurities, an increase in the susceptibility to decomposition by the mildew fungi used (decomposition degree 1–2) is expected compared to the case of the reference sample (decomposition degree 4). Method B shows that the trend towards increasing the material susceptibility to biodegradation is proportional to the concentration of dodecyl gallate in the polymer sample (Tables 3 and 4). Therefore, the addition of the natural antioxidant makes the elastomeric materials environmentally friendly by increasing their susceptibility to biodegradation. A method for using synthetic polymer materials directed towards their biodegradation after their exploitation life can then be proposed.
(Color online.) Influence of the microorganisms on the biodegradation of the Engage vulcanizates (method A).
Composite | Growth of microorganisms | |||
Part I | Picture | Part S | Picture | |
Reference sample | 1 | 1 | ||
Laryl gallate (1.25%) | 3 | 1 | ||
Lauryl gallate (4%) | 2 | – | – |
(Color online.) Influence of the microorganisms on the biodegradation of the Engage vulcanizates (method B and B′).
Composite | Growth of microorganisms | ||||
B | B′ | ||||
Sterile sample | |||||
Reference sample | 4 | 3 | 3 | ||
Lauryl gallate (1.25%) | 2 | 5 | 4 | ||
Lauryl gallate (4%) | 1 | 4 | – |
4 Conclusions
Dodecyl gallate is a component of green tea that possesses anti-oxidising properties. The addition of the antioxidant from the group of gallic acid esters improved the resistance of ethylene–octene rubber vulcanisates to UV, climatic and thermo-oxidative ageing processes. The change in deformation energy after the ageing of the vulcanisate sample containing gallate was considerably smaller compared to that of a reference sample.
Moreover, this antioxidant significantly prolonged the oxygen induction and decreased the energy emitted during oxidation by a factor of three. DSC and Tg measurements allowed the determination of the thermal stability of the antioxidant at temperatures of polymer processing up to 250 °C.
Tests of polymer–fungi interactions showed that the addition of dodecyl gallate to the elastomeric materials increases their susceptibility to biodegradation. In summary, the use of gallic acid esters improves the stability of elastomers in ageing processes under specified destruction. Simultaneously, the antioxidant increases the ecological benefit from the elastomer process by increasing the product's susceptibility to biodegradation.
Natural antioxidants can also be a pro-ecological alternative to aromatic amines with steric hindrance or to synthetic polyphenols used now in the industry.
Acknowledgements
This study was supported by Ministry of Science of Higher IP 2012 037072.