1 Introduction
Herbs and spices are invaluable resources and are useful in daily life as food additives, flavours, fragrances, pharmaceuticals, colors, or directly in medicine. This use of plants has a long history all over the world, and over the centuries, humanity developed better methods for the extraction of aromas from such materials. Aromas are complex mixtures of volatile substances generally present at low concentrations. Before such substances can be used or analysed, they have to be extracted from the matrix. Several extraction solvents or processes have been developed in order to obtain the best aromatic substance to satisfy the needs of the perfumer and of the flavourist, in accordance with the worldwide legislation governing the use of this product.
Currently, there are only two kinds of solvents that can be used in chemistry: solvents obtained from petroleum industry and solvents of agricultural origin, the so-called “bio-solvents”. Hexane has been used for decades for extraction of aromas in the perfume industry [1]. It offers satisfactory performances due to its low boiling point added to its low polarity. However, many works dealt with the toxic and hazardous effects of this solvent [2–5], and several investigations were also achieved using alternative solvents with the aim of more effective and greener extraction procedures, safer for users and more environmentally friendly [1,6–11]. Nevertheless, and despite its ranking on top of the list of the hazardous solvent, n-hexane is still the solvent of choice for extraction of aromas.
Because of negative effects on health and environment and of the recent REACH regulations, the need exists for replacing n-hexane. There are two possible solvents or alternative methods. A screening of non-polar or polar alternative solvent allows us to evaluate the best aromatic substance for the perfumer and the flavourist. Monoterpene hydrocarbons are less valuable than oxygenated compounds in terms of their contribution to the fragrance of the essential oil. Conversely, the oxygenated compounds are highly odoriferous and, hence, the most valuable. An alternative to these methods could be the extraction with supercritical carbon dioxide, since CO2 is a green solvent, readily available, cheap, with low critical temperature. The supercritical fluid extraction (SFE) has been applied successfully to other species as Rosemary (Rosemarinus officialis) [12], oregano (Origanum vulgare), thyme (Thymus zygis), sage (Salvia officinalis) [13], and orange (Citrus sinensis) [14].
In this paper, the potential of alternative solvents has been compared with that of a conventional solvent, n-hexane, as the current solvents and commercial situation calls for research with new extraction media. We have applied nine alternative solvents (α-pinene, MeTHF, ethyl acetate, methyl acetate, ethyl lactate, butanol, isopropanol, ethanol and CO2 supercritical fluid) and n-hexane to extract aroma from blackcurrant buds, largely used in perfumery. This study has been performed via experimentation and simulation using Hansen solubility methodology for the comprehension of the dissolution mechanism. We make appropriate comparisons in terms of extraction yields and aromatic profile.
2 Material and methods
2.1 Plants material
Frozen commercial blackcurrant buds (Ribes nigrum L.) were purchased from “Les côteaux bourguignons” cooperative, France. The initial moisture was 52.7 ± 1.1%. It was determined by a moisture analyser (MB35 by OHAUS).
2.2 Reagents
n-Hexane (analytical grade), ethyl acetate (purity 99%), ethanol (purity 96%), methyl acetate (purity 99%) were supplied by VWR International, Germany. (S)-Ethyl lactate (purity 99.9%) was purchased from Merck, Germany. 2-Propanol (purity 99.9%) α-pinene (purity 98%) and 1-butanol (99.4%) were provided by Sigma Aldrich, Germany. MeTHF was provided by Pannakem, USA.
2.3 Computational methods: HSPIP software
Hansen solubility parameters (HSP) are based on the concept that the total cohesive energy density is approximated by the sum of the energy densities required to overcome atomic dispersion forces (δd2), molecular polar forces arising from dipole moments (δp2), and hydrogen bonds (exchange of electrons, proton donor/acceptor) between molecules (δh2), as given in the following equation:
(1) |
The programme offers different ways to calculate HSPs: Yamamoto (Y-MB), Stefanis–Panayiotou, Van Krevelen, Hoy methods. The Yamamoto and Stefanis–Panayiotou methods are most recent [15,16]. The Stefanis–Panayiotou method is a new methodology for the calculation of groups’ contributions to HSPs. The molecular structures are decomposed into two kinds of functional groups: the first groups corresponding to UNIFAC ones, which describe the overall structure, and the second groups, which improve description. Hiroshi Yamamoto adapted his neural network (NN) technique; this adaptive system changes its structure based on external and internal information that flows through the network. This method calculates, thus, parameters directly from the molecular structure in computational format. For the third version, Hiroshi carried out a huge analysis of results on a database of many thousands of molecules, including many pharmacological, cosmetic and fragrance chemicals. He was able to refine his list of group fragments and also to test novel NN and Multiple Regression (MR) fits. For the user, the only difference from previous editions is that the estimates are often improved–particularly for very large molecules where we acknowledged that the original Y-MB encountered problems. In addition to the HSP values, Y-MB provides estimates of many other important parameters such as MPt, BPt, vapour pressures, critical constants and environmental values. Finally, the Yamamoto method has been chosen for this study.
For HSP solvent optimization, a simple composite affinity parameter, the relative energy difference (RED) number, has been calculated using Eq. (2) to determine whether the alternative solvent and the solute are miscible,
(2) |
(3) |
The factor 4 in Eq. (3), based on Prigogine's Corresponding States Theory, has proved to effectively expand the dimensions in order to give spherical plots. In general, the parameters follow the classical “like dissolve like” rule: the smaller Ra is, the greater the affinity between solute and solvent. It means that potentially good solvents exhibit RED numbers smaller than 1, while inappropriate solvents have progressively higher RED numbers larger than 1. The chemical structures of the solvents and solutes discussed in this article could be mutually transformed by JChemPaint version 3.0.1 software to their simplified molecular input line entry syntax (SMILES) notations, which were subsequently used to calculate the solubility parameters of various alternative solvents and volatile aroma compounds in bud blackcurrant. These solubility parameters were further modelled to a three-dimensional HSP sphere for better visualizing the solute/solvent interaction (HSPiP Version 4.0, Denmark).
An example compound with unknown HSPs is δ-3-carene. The knowledge of general chemical structure of δ-3-carene is of great importance for the prediction of its HSP (Fig. 1, No5). This chemical structure is then transformed to its SMILES notation CC1 = CCC2C(C1)C2(C)C for further HSP calculation using the Yamamoto–Molecular Break (Y-MB) method, which can break SMILES into corresponding functional groups and then estimate the HSPs of δ-3-carene. This method has been embedded in HSPiP software in order to facilitate the direct calculation of the HSP of δ-3-carene (δd: 17.0, δp: 1.3, δh: 2.0, δtotal: 17.17, Table 1). Other compounds in Fig. 1 could be handled in a similar way.
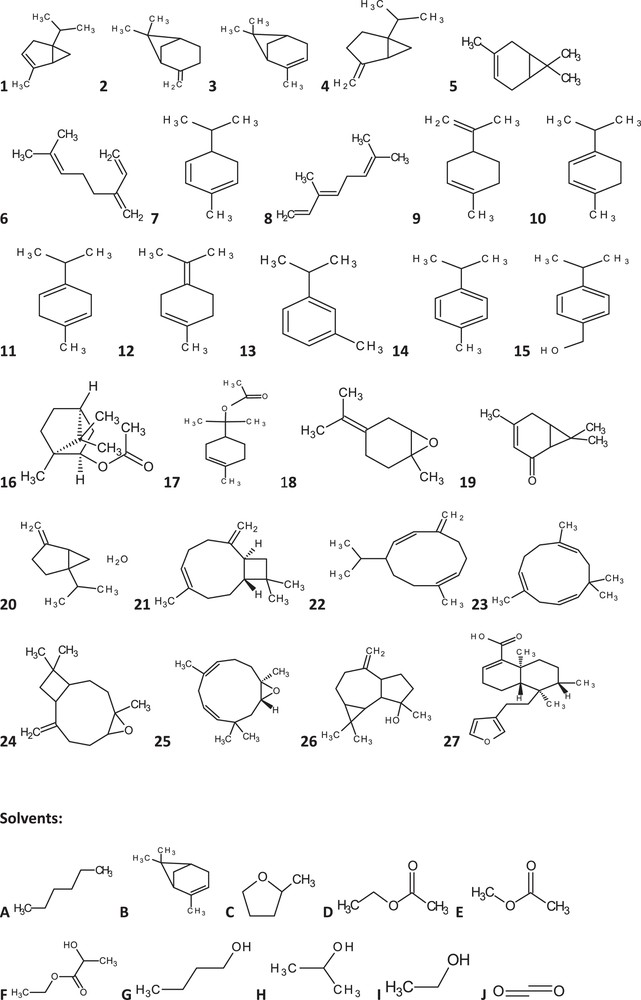
Blackcurrant buds’ aromas.
Hansen solubility parameters (HSP).
Hansen parameters | ||||
No. | Compounds | δd (MPa1/2) | δp (MPa1/2) | δh (MPa1/2) |
1 | α-Thujene | 16.4 | 2.4 | 2.5 |
2 | β-Pinene | 16.9 | 1.6 | 1.8 |
3 | α-Pinene | 17 | 1.3 | 2 |
4 | Sabinene | 16.3 | 2.5 | 0 |
5 | δ-3-Carene | 17 | 1.3 | 2 |
6 | Myrcene | 15.8 | 2 | 4.2 |
7 | α-Phellandrene | 16.4 | 1.9 | 3 |
8 | β-ocimene | 16 | 2 | 4.4 |
9 | Limonene | 16.7 | 2.2 | 4.9 |
10 | α-Terpinene | 16.4 | 0.7 | 2.7 |
11 | δ-Terpinene | 16.6 | 2.9 | 2.9 |
12 | Terpinolene | 16.9 | 1.8 | 4.8 |
13 | m-Cymene | 17.9 | 1.2 | 1.8 |
14 | p-Cymene | 18.5 | 2.6 | 1.9 |
15 | p-Cymen-8-ol | 18.3 | 5.7 | 10.2 |
16 | Bornyl acetate | 16.8 | 4.3 | 4.5 |
17 | α-Terpinyl acetate | 16.3 | 3.6 | 4.8 |
18 | Terpinolene epoxid | 16.6 | 4.4 | 4.2 |
19 | 3-Caren-5-one | 17.4 | 8 | 3 |
20 | Sabinene hydrate | 17 | 5.2 | 12.1 |
21 | β-caryophyllene | 16.9 | 1.4 | 2.2 |
22 | Germacrene d | 16.5 | 1 | 2.9 |
23 | α-Humulene | 16.7 | 1.6 | 3.2 |
24 | Caryophyllene oxid | 17.1 | 3.2 | 0 |
25 | Humulene epoxid | 17 | 3.2 | 4.1 |
26 | Spathulenol | 17.5 | 3.2 | 4.4 |
27 | Hardwickic acid | 17.6 | 2.9 | 4 |
Solvents | ||||
A | Hexane | 15.2 | 0.8 | 2 |
B | α-Pinene | 17 | 1.3 | 2 |
C | MeTHF | 16.9 | 4.2 | 4.1 |
D | Ethyl acetate | 15.6 | 6 | 7.2 |
E | Methyl acetate | 15.6 | 6.1 | 8.1 |
F | Ethyl lactate | 16 | 7.6 | 12.5 |
G | Butanol | 15.6 | 6.6 | 15.8 |
H | Isopropanol | 15.1 | 8 | 14.3 |
I | Ethanol | 15.8 | 8.8 | 19.4 |
J | CO2 | 15.2 | 0 | 7.1 |
2.4 Solid–liquid extraction
Extraction from blackcurrant bud at the boiling point of each solvent (hexane, α-pinene, MeTHF, ethyl acetate, methyl acetate, ethyl lactate, butanol, isopropanol or ethanol, respectively) was done under conventionally heated reflux. Twenty-five grams of blackcurrant bud were precisely weighed and placed in a flask containing 100 mL of solvent. The buds were submerged and extracted at the boiling point of each solvent for two hours.
The sample was filtered, then the oil in the flask was desolventised in the rotary vacuum evaporator and finally stored at 4 °C until used. All experiments were carried out in triplicates.
2.5 Supercritical fluid extraction (SFE)
Extraction was carried out in a dynamic supercritical fluid extractor unit (Water's instrument, SuperParticle SAS200 System) comprising a 2-L cylinder extraction cell and a separator of 0.5-L capacity, with independent temperature and pressure control. A co-solvent pump was connected to the extraction line in order to supply the modifier (organic solvent at high pressure) at a pre-established flow rate, to be mixed with the CO2 flow before reaching the extraction vessel. Ethanol (purity 96%, VWR international) was used as a co-solvent. Briefly, the extraction procedure consisted in placing 170 g of blackcurrant bud finely ground and dried inside the extractor to form the fixed bed of particles, followed by the control of the process variables (temperature, pressure and solvent flow rate, Fig. 2).
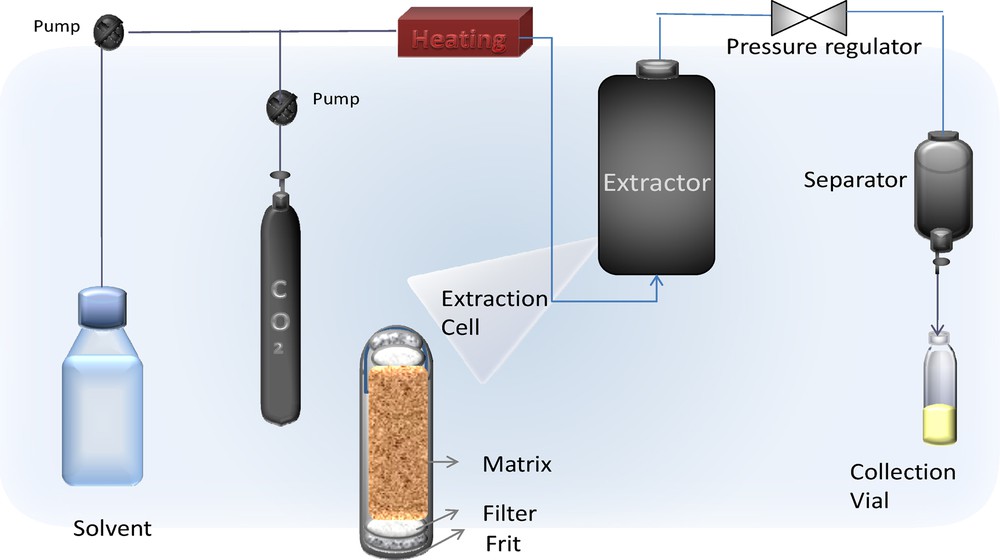
(Color online.) Supercritical fluid extraction (SFE).
The assays were conducted in similar temperature and flow rate conditions. The extractions were performed at 35 °C and a constant flow rate of 20 g/min. The first extraction was performed at a constant pressure of 100 bar and the second extraction was carried out at a constant pressure of 200 bar. The co-solvent was separated from the solute using low-pressure methods (separators at 0 °C). The samples recovered were solid and pasty. In order to ensure an accurate determination of extraction yield with time, the separator was washed with ethanol and the residual material recovered in each case was mixed with the corresponding solid fraction. Ethanol was evaporated under vacuum and kept at 4 °C before chromatographic analysis. The extractions were performed at least three times.
2.6 GC and GC–MS identification
2.6.1 Gas chromatography by flame ionic detector (FID)
GC analysis was carried out using an Agilent 7890A gas chromatograph, under the following operating conditions: vector gas, helium; injector and detector temperatures, 300 °C; injected volume, 1 μL; split ration 10:1; ZB-1MS™ (polydimethylsiloxane, 60 m × 0.25 mm i.d. × film thickness 0.25 μm; Phenomenex, Le Pecq, France). Temperature was programmed at 60 °C for 8 min, then increased from 60 to 120 °C at 3 °C/min, then to 250 °C at 5 °C/min, then maintained under isocratic mode during 10 min, then increased to 300 °C at 15 °C/min, then maintained under isocratic mode during 20 min. Retention indices were determined with C6 to C30 alkane standards as references. Relative amounts of individual components are based on peak areas obtained without FID response factor correction. Three replicates were performed for each sample. The average of these three values and the standard deviation were determined for each component identified.
2.6.2 Gas chromatography–mass spectrometry analysis
GC–MS analysis was carried out using an Agilent 7890A coupled to an Agilent 5975 C MS (Agilent, Massy, France). The samples were analysed according to the same chromatographic conditions as above (2.6.1); ion source temperature, 230 °C; transfer line temperature, 280 °C; ionization energy, 70 eV; electron ionisation mass spectra were acquired over the mass range 29–450 amu.
2.6.3 Identification of the components
The identification of the components was based on computer matching against commercial (NIST05, Wiley) and home-made databases, laboratory mass spectra libraries built up from pure substances, and MS literature data combined with comparison of GC retention indices (RI) on measured on an apolar column. RIs were calculated with the help of a series of linear alkanes C6–C30 investigated on an apolar column (ZB-1MS™). Compounds that were available in the laboratory were confirmed by co-injection of the external standard compound.
3 Results and discussion
3.1 Composition of extracts
The compounds were identified using GC–MS, while the content of separated components was measured by GC–FID (Table 2). A total of 30 major compounds (in agreement with the literature) were identified in blackcurrant extract extracted using n-hexane. The compounds that were identified in aroma extracts constituted 73% of the total integrated GC peak area of each terpene in the n-hexane extract. The content in monoterpenes and sesquiterpenes was 40.58% and 7.15%, respectively, while the amount of oxygenated monoterpenes and sesquiterpenes was 17.9% and 7.36%, respectively. The most abundant volatile compounds in blackcurrant buds were monoterpenes, such as δ-3-carene (21.45%) and terpinolene (11.34%). Other quantitatively important compounds in aroma extracts of buds were sabinene (4.93%), β-caryophyllene (4.51%), caryophyllene oxide (4.16%), p-cymen-8-ol (2.85%), trans-β-ocimene (2.79%), β-phellandrene (2.06%), β-myrcene (1.86%), α-humulene (1.82%), cis-β-ocimene (1.69%), spathulenol (1.61%), humulene epoxide II (1.59%), limonene (1.41%), terpinolene epoxide (1.22%), and 3-caren-5-one (1.13%).
Composition of blackcurrant bud aromatic extracts detected by gas chromatography.
Alternatives solvents | ||||||||||||
Hexane | MeTHF | Ethyl acetate | Isopropanol | Butanol | Ethanol | Methyl acetate | Ethyl lactate | α-pinene | Supercritical CO2 | |||
100 bar | 200bar | |||||||||||
Compounds | RI | Area% | ||||||||||
Monoterpenes | ||||||||||||
α-Thujene* | 929 | 0.25 | 0.28 | 0.24 | 0.12 | |||||||
α-Pinene* | 938 | 0.93 | 0.79 | 0.8 | tr | 16.27 | ||||||
Sabinene | 968 | 4.93 | 3.88 | 3.82 | tr | 0.14 | tr | tr | tr | |||
β-Pinene* | 973 | 0.85 | 0.75 | 0.82 | tr | tr | tr | |||||
β-Myrcene* | 983 | 1.86 | 1.46 | 1.52 | tr | tr | 0.22 | 0.13 | ||||
2 Carene* | 1001 | 0.31 | 0.27 | 0.29 | tr | tr | tr | |||||
α-Phallandrene* | 998 | 0.17 | 0.15 | 0.16 | tr | tr | tr | |||||
δ-3-Carene* | 1002 | 21.45 | 17.55 | 18.13 | 0.29 | 0.71 | 1.97 | 1.26 | ||||
α-Terpinene* | 1009 | 0.81 | 0.81 | 0.69 | 0.68 | 0.19 | 0.12 | 0.29 | 0.14 | |||
p-Cymene* | 1013 | 0.82 | 0.56 | 0.57 | 0.13 | 0.13 | 0.2 | 0.11 | ||||
β- Phellandrene* | 1022 | 2.06 | 1.57 | 1.61 | 0.19 | 0.29 | tr | 0.45 | 0.25 | |||
Limonene* | 1025 | 1.41 | 1.07 | 1.11 | 0.17 | 0.22 | 0.16 | 0.25 | 0.12 | |||
trans-β-Ocimene* | 1026 | 2.79 | 2.24 | 2.28 | 0.15 | 0.29 | 0.77 | 0.33 | ||||
cis-β-Ocimene* | 1037 | 1.69 | 1.35 | 1.39 | 0.12 | 0.22 | 0.52 | 0.2 | ||||
γ-Terpinene* | 1040 | 0.25 | 0.29 | 0.29 | 0.31 | 0.11 | tr | tr | ||||
Oxygenated monoterpenes | ||||||||||||
cis-Sabinene hydrate* | 1068 | 0.35 | ||||||||||
Terpinolene* | 1080 | 11.34 | 8.16 | 8.05 | 1.06 | 2.24 | tr | 4.3 | 1.86 | |||
trans-Sabinene hydrate* | 1097 | 0.33 | 0.23 | 0.22 | 0.3 | 0.38 | 0.56 | 0.38 | ||||
Terpinolene epoxide | 1.22 | 0.46 | 0.58 | 0.21 | 0.97 | 0.52 | ||||||
p-Cymen-8-ol* | 1163 | 2.85 | 1.73 | 1.89 | 3.27 | 3.31 | 3.3 | 2.65 | tr | 5.46 | 3.67 | |
Bornyl acetate* | 1270 | 0.36 | 0.35 | 0.38 | 0.67 | 0.36 | 0.68 | 0.53 | ||||
3-Caren-5-one | 1.13 | 0.69 | 0.79 | 1.57 | 1.61 | 1.81 | 1.91 | 1.93 | ||||
α-Terpinyl acetate* | 1340 | 0.32 | 0.35 | 0.33 | 0.45 | 0.45 | 0.83 | 0.74 | ||||
Sesquiterpenes | ||||||||||||
β-Caryophyllene* | 1420 | 4.51 | 6.37 | 6.22 | 7.71 | 9.46 | 2.27 | 3.35 | 3.54 | 0.14 | 9.53 | 0.82 |
α-Humulene* | 1452 | 1.82 | 2.32 | 2.32 | 2.9 | 3.6 | 1.51 | 3.4 | 1.56 | 0.1 | 3.89 | 4.11 |
Germacrene D* | 1480 | 0.82 | 1.29 | 1.19 | 1.58 | 2.03 | 2.19 | 2.34 | ||||
Oxygenated sesquiterpenes | ||||||||||||
Spathulenol* | 1576 | 1.61 | 1.14 | 1.16 | 2.16 | 1.83 | 1.27 | 2.87 | 2.27 | |||
Caryophyllene oxide* | 1562 | 4.16 | 2.69 | 2.81 | 4.27 | 4.3 | 1.67 | 8.43 | 7.43 | |||
Humulene epoxide II* | 1600 | 1.59 | 1.04 | 1.09 | 4.01 | 1.86 | 1.03 | 3.14 | 2.9 | |||
Other compound | ||||||||||||
Hardwickic acid | 5.1 | 11.06 | 12.55 | 5.73 | 17.89 | 17.28 | 8.32 | 21.15 | ||||
Extraction times | 120 min | 150 min | ||||||||||
Yield % | 3.87% | 7.10% | 8.78% | 5.77% | 7.77% | 4.62% | 14.66% | 8.78% | 2.62% | 1.03% | ||
Total non-oxygenated compounds | 47.73 | 42.88 | 43.44 | 13.74 | 17.35 | 3.78% | 6.75% | 5.10% | 16.79% | 20.28% | 9.81% | |
Total oxygenated compounds | 25.26 | 17.06 | 17.52 | 26.9 | 16.96 | 5.11% | 2.65% | 3.96% | 29.77% | 22.61% |
However, their relative proportions depend on the extraction solvent used. It was interesting to note that MeTHF and ethyl acetate show relative proportions similar to the case of the n-hexane extract, with 42.88% and 43.44% of non-oxygenated compounds, respectively, while the amount of oxygenated compounds was 17.06% and 17.52%, respectively.
Thus, these agro solvents showed potential to be alternatives to petroleum-derived solvents as n-hexane used in the perfume industry.
3.2 Solubility solute–solvent by Hansen's parameters
Theoretical screening methods are based on a thermodynamic description of the investigated system. Here, the selection of solvents is based upon the solubility parameters of solvent–solute systems. The useful prediction method proposed by Yamamoto was applied to calculate HSPs of alternatives solvents and terpenes in blackcurrant extracts (Table 1). Two dimensional (2D) graph of δp versus δh parameters (Fig. 3) shows the different aromas in blackcurrant and the different alternative solvents according to Hansen's parameters. The influence of the structure of the molecule on polarity is presented. In fact, terpenes and n-hexane display similar HSP values, whereas oxygenated compounds have different HSPs due to their hydroxyl group or carboxyl group, which resulted in a higher δh value. The calculated HSP values were close to those of some alternative solvents, which demonstrated that terpenes may dissolve in these alternative solvents according to the principle “like extracts like”. The relative energy difference (RED) numbers have been used to characterize and quantify solute–solvent interactions. Table 3 represents the RED modelling optimization of solvents for the extraction of target terpene. All solvents (0 < RED < 1) that were close to the centre (RED = 0) could be considered as good solvents for each terpene. The solubility of alternative solvents used in the extraction of target solutes has been respectively characterized using the 2D graph for an easy understanding of the solubility of various solvents in solid–liquid extraction. (circles materialize the prediction data). The alternative solvents showed various theoretical solubilities for each terpene, with a significant difference that would be validated by experiments. This result could be explained by the difference in the solvents’ polarities. Hexane and α-pinene included clusters of monoterpenes and sesquiterpenes. Indeed, α-pinene solvent showed solubility parameters with aroma compounds similar to those of hexane. MeTHF and ethyl acetate are more polar than hexane, and thus included clusters of oxygenated monoterpenes (aldehydes and ketones).
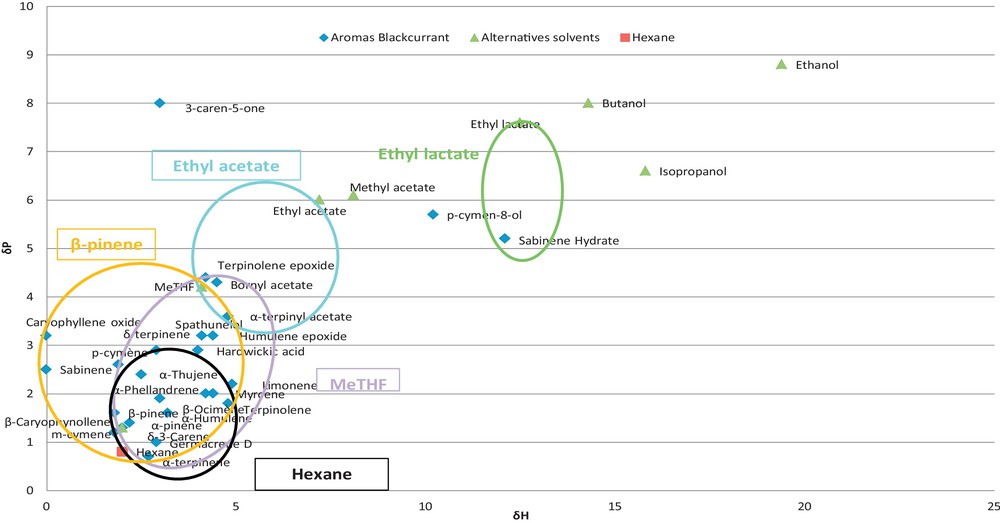
(Color online.) Alternative solvents for solubilizing blackcurrant aromas according to Hansen's settings.
Relative energy difference (RED).
Solvents (RED = 0) | |||||||||||
A | B | C | D | E | F | G | H | I | J | ||
Compounds | Hexane | α-Pinene | MeTHF | Ethyl acetate | Methyl acetate | Ethyl lactate | Butanol | Isopropanol | Ethanol | CO2 | |
Monoterpenes | |||||||||||
1 | α-Thujene | 0.73b | 0.43b | 0.65b | 1.53c | 1.73c | 2.82c | 3.51c | 3.33c | 4.53c | 1.43c |
2 | β-Pinene | 0.87b | 0a | 0.87b | 1.86c | 2.04c | 3.1c | 3.77c | 3.62c | 4.79c | 1.62c |
3 | α-Pinene | 0.91b | 0a | 0.9b | 1.89c | 2.06c | 3.1c | 3.76c | 3.63c | 4.77c | 1.59c |
4 | Sabinene | 0.86b | 0.68b | 1.15c | 2.03c | 2.24c | 3.38c | 4.1c | 3.88c | 5.11c | 1.996c |
5 | δ-3-Carene | 0.91b | 0b | 0.9b | 1.89c | 2.06c | 3.1c | 3.76c | 3.63c | 4.77c | 1.59c |
6 | β-Myrcene | 0.69b | 0.83b | 0.78b | 1.25c | 1.42c | 2.51c | 3.12c | 2.96c | 4.16c | 0.93b |
7 | α-Phellandrene | 0.71b | 0.42b | 0.68b | 1.52c | 1.7c | 2.78c | 3.43c | 3.28c | 4.46c | 1.28c |
8 | β-Ocimene | 0.78b | 0.8b | 0.71b | 1.24c | 1.4c | 2.46c | 3.08c | 2.93c | 4.12c | 0.93b |
9 | Limonene | 1.10c | 0.77b | 0.55b | 1.24c | 1.38c | 2.36c | 2.99c | 2.87c | 4.01c | 1.08c |
10 | α-Terpinene | 0.63b | 0.38b | 0.98b | 1.78c | 1.95c | 3c | 3.61c | 3.49c | 4.65c | 1.27c |
11 | γ-Terpinene | 0.90b | 0.5b | 0.47b | 1.42c | 1.61c | 2.69c | 3.39c | 3.21c | 4.4c | 1.46c |
12 | Terpinolene | 1.13c | 0.71b | 0.63b | 1.37c | 1.5c | 2.45c | 3.07c | 2.98c | 4.09c | 1.12c |
13 | m-Cymene | 1.35c | 0.45b | 1.07c | 2.14c | 2.3c | 3.26c | 3.92c | 3.82c | 4.91c | 1.92c |
14 | p-Cymene | 1.71c | 0.82b | 1.05c | 2.14c | 2.3c | 3.19c | 3.9c | 3.78c | 4.83c | 2.2c |
Oxygenated monoterpenes | |||||||||||
15 | p-Cymen-8-ol | 2.85c | 2.42c | 1.72c | 1.55c | 1.45c | 1.37c | 1.96c | 1.99c | 2.73c | 2.24c |
16 | Bornyl acetate | 1.34c | 0.98b | 0.11b | 1c | 1.17c | 2.2c | 2.94c | 2.75c | 3.92c | 1.49c |
17 | α-terpinyl acetate | 1.13c | 0.97b | 0.38b | 0.92b | 1.09c | 2.17c | 2.87c | 2.69c | 3.88c | 1.2c |
18 | Terpinolene epoxide | 1.27c | 0.97b | 0.16b | 0.99b | 1.18c | 2.24c | 2.99c | 2.78c | 3.98c | 1.49c |
19 | 3-Caren-5-one | 2.12c | 1.71c | 1.02c | 1.47c | 1.63c | 2.48c | 3.34c | 3.05c | 4.18c | 2.5c |
20 | Sabinene hydrate | 2.90c | 2.71c | 2.02c | 1.43c | 1.24c | 0.79b | 1.21c | 1.3c | 2.12c | 2.02c |
Sesquiterpenes | |||||||||||
21 | β-caryophyllene | 0.86b | 0.08b | 0.85b | 1.82c | 1.99c | 3.04c | 3.7c | 3.56c | 4.71c | 1.53c |
22 | Germacrene d | 0.69b | 0.34b | 0.88b | 1.71c | 1.88c | 2.92c | 3.54c | 3.42c | 4.58c | 1.27c |
23 | α-humulene | 0.83b | 0.34b | 0.7b | 1.75c | 1.42c | 2.79c | 3.43c | 3.3c | 4.45c | 1.29c |
Oxygenated sesquiterpenes | |||||||||||
24 | Caryophyllene oxide | 1.23c | 0.69b | 1.06c | 2.07c | 2.28c | 3.36c | 4.11c | 3.9c | 5.09c | 2.17c |
25 | Humulene epoxide | 1.20c | 0.71b | 0.25b | 1.26c | 1.42c | 2.42c | 3.13c | 2.97c | 4.12c | 1.42c |
26 | Spathulenol | 1.43c | 0.81b | 0.4b | 1.37c | 1.51c | 2.42c | 3.12c | 3c | 4.09c | 1.56c |
Other oxygenated compound | |||||||||||
27 | Hardwickic acid | 1.40c | 0.71b | 0.48b | 1.5c | 1.64c | 2.56c | 3.25c | 3.13c | 4.22c | 1.6c |
a RED = 0 perfect solvency.
b 0 < RED < 1 good solvency.
c RED > 1 poor solvency.
3.3 Comparison between experimental data and simulation
The experimental data were compared with results from modelling. Coupling the Hansen method to experimental solubility profiles was found to be good tools for predicting single-use solvents’ properties. It has some defects in the case of non-standard solvents with large molecules, complex multi-component systems, etc. However, these defects have not completely affected the interest in selecting HSPs as a green alternative solvent. Hansen's method describe α-pinene as a good alternative for aroma extraction, but experimentally the flavour profile is very different from that of the hexane extract. This difference can be explained by the difficulty to evaporate the solvent. Coupling Hansen's method to experimental solubility profiles, MeTHF appeared as the alternative solvent most likely to replace hexane. The 2-D graph of δp versus δh (Fig. 4) shows the different blackcurrant aroma and the different alternative solvent according to Hansen's parameters. Circles materialize experimental and prediction data for n-hexane and MeTHF. This graph allows visualizing the miscibility of aromas and alternative solvents from both experimental and theoretical points of view. The results with MeTHF were in good consistency with predictive HSP results. In other words, MeTHF has been chosen for two reasons: the first one is that there is a good correlation between theory and practice, and the second one is that the aromatic extract is comparable to the extract using hexane. Furthermore, MeTHF is an agrosolvent produced from by-products of agriculture (waste of corn cobs) and is not a carcinogenic, mutagenic, and reprotoxic (CMR) compound [11].
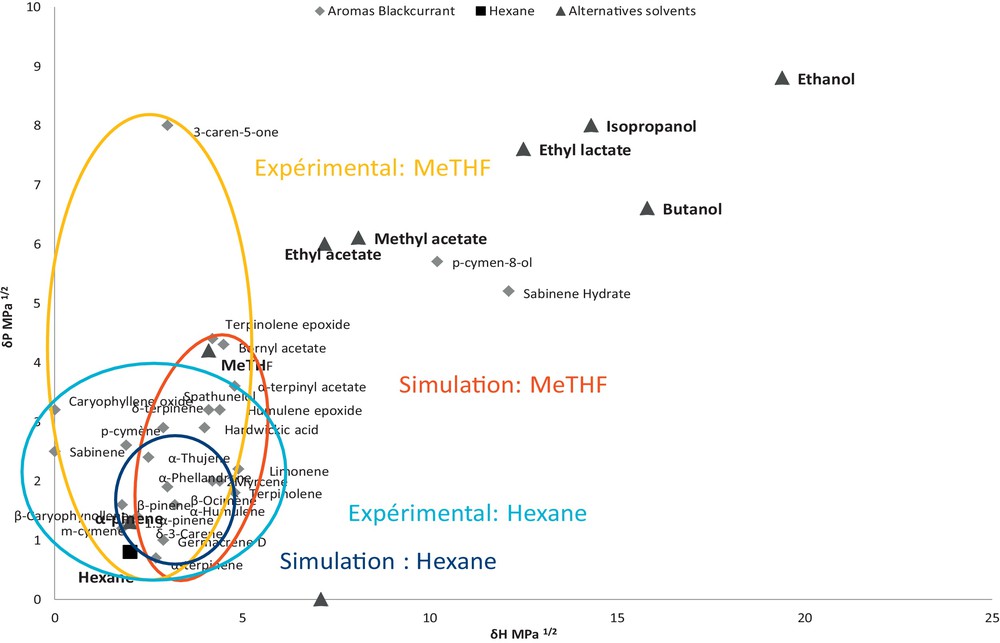
(Color online.) Hexane and MeTHF: simulation and experiments.
4 Conclusion
In this study, we evaluated the performance of nine alternative solvents (α-pinene, MeTHF, ethyl acetate, methyl acetate, ethyl lactate, butanol, isopropanol, ethanol and CO2 supercritical fluid) for extracting aromas from blackcurrant buds (Ribes nigrum L) compared to n-hexane, commonly used. MeTHF proved theoretically and experimentally to be a preferential solvent that can be safely and effectively used to replace n-hexane in the extraction of aromas. It is also a promising solvent for n-hexane substitution in terms of quality of the extract (oxygenated terpenes). It is an alternative solvent that is recognized as economically viable and environmentally benign to be used as a substitute for petroleum-origin solvents such as n-hexane.
Acknowledgements
Aurore Filly thanks “Région PACA” (Provence–Alpes–Côte d’Azur) and competitivity cluster PASS (Pôle Arômes Senteurs et Saveurs) for her doctoral grant.