1 Introduction
Phosphorus (P) is an essential nutrient for all forms of life. It is necessary for storage and processing of genetic information [1]. It is generally considered as a limited factor for crop growth in agricultural soils, emphasizing the need to replenish soil phosphorus in order to enhance and maintain productivity. As a result, during the last century, humanity has massively mobilized phosphorus from natural geological resources for synthetic fertilizers production. Indeed, according to the international fertilizer industry association [2], the consumed amounts of phosphorus-rich fertilizers have increased from about 73.8 million tons in 1972 to more than 142.6 million tons in 2010. In this context, based on phosphorus consumptions and natural reserves patterns, Cordell et al. [3] have estimated that the peak global production of phosphorus would be reached around 2035, after which demand would out-strip supply.
At the same time, relatively high quantities of phosphorus are discharged into receiving water bodies and there is no environmental or technological reasons preventing its recovery and reuse. According to Biswas et al. [4], the average discharged phosphorus amount in Western Europe alone has exceeded 250,000 tons per year. In Tunisia, this amount was estimated to be about 1840 tonnes per year [5]. On the other hand, phosphorus and nitrogen are considered as the main chemical elements responsible for water body eutrophication. This process can seriously disturb the balance of organisms present in water and negatively affect water quality, mainly through the depletion of the dissolved oxygen level as the algae decay [6]. Thus, phosphorus recovery from wastewaters and its reuse for agricultural purposes could bring practical solutions regarding both the intensive exhaustion of high-grade phosphate ores and the reduction in the biodiversity of the P-polluted water bodies. Currently, the main technologies allowing phosphorus removal and recovery from wastewaters are accumulation into micro-organisms, struvite crystallization and sorption onto natural materials technologies [3,7,8]. Biological phosphorus accumulation requires highly skilled operations regarding especially the type of the micro-organisms, the nature of the carbon source and the contents of volatile fatty acids [1]. Struvite production from wastewater treatment systems is confronted to the high cost of the used chemical reagents for pH adjustment and artificial magnesium adding [9]. Phosphorus removal and recovery from wastewaters using low-cost, abundant and renewable materials through adsorption or precipitation processes has been pointed out this last decade as an interesting research option [10]. Several organic and mineral natural materials and wastes have been tested for phosphorus removal from synthetic or real wastewaters such as pyrrhotite [11], phosphate mine slimes [5] and Posidonia oceanica fibres [12]. The best phosphorus removal efficiencies have been registered at alkaline pH for calcium-rich materials such as sepiolite [13], oyster shell [14], dolomite [15], shell sand [16], and crab shells [17].
Powdered marble wastes also contain relatively high contents of calcium and, as a consequence, could efficiently remove phosphorus from aqueous solutions. Very little work has been carried out regarding the use of this raw or modified material for the removal of phosphorus from highly concentrated effluents. The main related studies have concerned their reuse as porous media filter in subsurface flow-constructed wetlands technique [18,19] and for phosphorus removal from moderately P-rich synthetic solutions [20]. More than 56 million tons of marble wastes have been produced worldwide in 2003 during the quarrying, processing, and polishing procedures [21,22]. In Tunisia, these amounts were estimated to be overall about 0.7 million tons of produced marbles; industry produces about 1 million tons and generates huge amounts of wastes [21]. These wastes are generally disposed in empty pits or landfills, without any reuse. The reuse of these wastes for phosphorus removal and recovery from wastewaters is environmentally beneficial because it preserves water bodies against eutrophication, diminishes the discharged PMW amounts in landfills and allows the possible recycling of the resulting complex phosphorus low-cost material in agriculture as a fertilizer.
The main goals of this research work are:
- • to test the efficiency of RPMW and CPMW for phosphorus removal from highly concentrated phosphorus synthetic solutions and real wastewater under different experimental conditions;
- • to carry out an in-depth critical investigation of the phosphorus removal mechanisms through not only specific analyses, but also a fundamental approach.
2 Materials and methods
2.1 Solid matrixes preparation and characterization
In this study, the RPMW were collected from a marble manufacture located in Borj Cedria city (25 km to the south of Tunis, Tunisia). They originated from large stones of “Bianco Gioia” marble. These wastes were firstly sieved mechanically using a sieve with a mean diameter of 1 mm in order to remove any existing fragments. Then, they were washed with distilled water and air dried for several days to a constant weight. The CPMW were obtained from the calcination under an O2 atmosphere of RPMW for 1 h at a temperature of 1000 °C using an electric furnace “Thermolyne Muffle furnace, 1400”. The particle size distribution of the two used solid matrixes was achieved using a Malvern Mastersizer STD06 laser granulometer. Their elemental composition was determined by energy dispersive spectroscopy (EDS) using a Quanta-200-Fei apparatus. Finally, the pHs of zero point charge (pHZPC) of the two solid matrices were determined according to the solid addition method using 0.01 M KNO3 solutions, 1 g of solid matrix for initial pH values of 2, 4, 6, 8, 10, and 12 [23].
2.2 Studies of calcium and OH−release from RPMW and CPMW
The dissolved calcium and hydronium concentrations are key parameters that significantly affect the degree of phosphorus removal from aqueous solutions [15]. Therefore the impact of the initial aqueous pH on the capacities of RPMW and CPMW in releasing Ca2+ and OH−ions has been determined in a series of batch experiments. The tested initial pH values were set to 5, 8, and 11. These experiments were conducted in triplicate at room temperature (20 ± 2 °C) in 120-mL capped flasks. For each experiment, 0.1 g of RPMW or CPMW was shaken in 100 mL of distilled water (blank assays: without phosphorus) at 400 rpm using a Varimag-poly15 magnetic stirrer. pH values and total Ca2+ concentrations of the samples were measured at several contact times. The pH values of the solutions were expressed in OH−concentrations (). The experimental release capacities of Ca2+ and OH−release (; ) from RPMW and CPMW were calculated as follows:
(1) |
(2) |
2.3 Batch experiments for phosphorus removal by RPMW and CPMW from synthetic solutions
The synthetic phosphorus solutions were prepared from potassium dihydrogen phosphate (KH2PO4), acquired from Fisher Scientific. A stock phosphorus solution of 1000 mg·L−1 was prepared with distilled water and used during this study. The batch experiments consisted in determining the effect of contact time, initial aqueous concentration, solutions pH and solid matrixes dosage on phosphorus removal by RPMW and CPMW. These experiments were conducted at 20 ± 2 °C in 120-mL capped flasks. During these assays, a defined amount of RPMW or CPMW was shaken in 100 mL of phosphorus solution for a fixed time at 400 rpm using a Varimag-poly15 magnetic stirrer. The residual phosphorus concentration and final aqueous pH were measured after sample filtration through 0.45-μm filters. Table 1 summarizes the experimental conditions of the performed assays relative to phosphorus removal from synthetic solutions by RPMW and CPMW.
Summary of the experimental conditions for the study of phosphorus removal from synthetic solutions by RPMW and CPMW.
Assays | C0,p (mg·L−1) | D (g·L−1) | pH | tc (min) |
Effect of tc | 350 | 1 | 5 | 1; 5; 15; 30; 60; 120; 180 (CPMW) 1; 5; 15; 30; 60; 120; 180; 300; 480; 720; 960 (RPMW) |
Effect of initial C0,P and pH | 150; 200; 250; 300; 350 | 1 | 5; 8; 11 | 960 (RPMW) 180 (CPMW) |
Effect of the solid dosage | 350 | 1; 2; 4; 6; 8; 10; 12; 14 | 5 | 960 (RPMW) 180 (CPMW) |
The removed phosphorus amount at a given time, t, (qt,P: mg·g−1) by RPMW or CPMW was calculated as below:
(3) |
The phosphorus removal efficiency (PRE) of RPMW or CPMW at a given time is deduced as follows:
(4) |
Each analysis point reported in this study was an average of at least three independent parallel sample solutions. The corresponding standard deviations of the results reported hereafter were ± 3%.
In order to get a better understanding of the mechanisms involved in phosphorus removal by RPMW and CPMW, scanning electron microscopy (SEM) observations, energy dispersive spectroscopy (EDS) and Fourier transform infrared spectroscopy (FTIR) analyses were performed on the surface of these solid matrixes before and after phosphorus removal experiments. SEM and EDS analyses have been carried out after coating of the samples with conductive carbon with a Quanta-200-Fei apparatus. The FTIR analyses were conducted using the KBr method with a Magna-IR-560 apparatus. The related spectral resolution is 1 cm−1, measured between 400 and 4000 cm−1. On the other hand, X-ray diffraction (XRD) analyses were performed in order to characterize the solid phase (precipitate) formed after contact between phosphorus and calcium ions. This crystalline phase was analyzed using an X-ray diffractometer Cu Kα radiation, PW 1710, Philips, and scans were conducted from 0° to 60° at a rate of 2°·min−1.
2.4 Batch experiments for phosphorus removal by CPMW from real wastewaters
CPMW has been used to carry out additional assays regarding phosphorus removal from real wastewater. The main aim of this kind of experiments is to check the CPMW efficiency for relatively complex solution compositions. The used wastewater has been collected from a manufacture of dairy products located in Soliman city in northeastern Tunisia. It has high chemical oxygen demand, phosphorus and calcium contents (Table 2). During these experiments, in order to compare the phosphorus removal efficiencies observed for synthetic solutions, the initial aqueous pH was fixed to 5 for the used wastewater. The tested CPMW doses were fixed to 1, 4, and 10 g·L−1. As for the synthetic solutions, triplicate experiments were performed for a contact time of 3 h.
Main physicochemical characteristics of the used real wastewater.
Parameter | Content |
pH (–) | 4.76 |
COD (mg·L−1) | 26,200 |
Phosphates (mg·L−1) | 1000.00 |
Calcium (mg·L−1) | 1375.00 |
Magnesium (mg·L−1) | 464.75 |
Potassium (mg·L−1) | 1312.00 |
Carbonates (mg·L−1) | 18.30 |
Chlorides (mg·L−1) | 518.82 |
The molar ratios (MR) of removed calcium to phosphorus (mol Ca/mol P) from the real wastewater were calculated as follows in order to get a better understanding of the nature of the formed precipitate:
(5) |
2.5 Analytical methods
The analysis of phosphorus species concentrations was performed spectrophotometrically at 880 nm, following the ascorbic acid method [24], using a Thermo Spectronic UV1 model. Calcium concentrations were measured through the EDTA method [25]. During the assays, the pH measurements were performed by a laboratory pH meter (692 pH/Ion Meter, Metrohm).
3 Results and discussion
3.1 Used solid matrixes characterization
The physicochemical characterization of the two used solid matrixes is shown in Table 3. RPMW and CPMW can be considered as very fine solid porous media and therefore used as fine reactive filters media with probable high removal capacities. Indeed their mean diameters are evaluated at about 28.0 and 32.1 μm for CPMW and RPMW, respectively. The presence of relatively small size particles in the RPMW and CPMW should play an important role in physicochemical reactions with phosphate ions (Fig. 1a–b). EDS analyses indicate that RPMW is exclusively formed by calcite with high contents of Ca, C and O. However, CPMW contains higher contents of Ca and O due to the calcination process (Fig. 1c–d). Very low Mg contents are observed for both RPMW and CPMW. No Fe or Al is detected. Since Ca is important for the removal of phosphorus via sorption and precipitation processes [9], the RMPW and especially CPMW could present an interesting ability for phosphorus removal from aqueous solutions.
Main physicochemical characteristics of the used solid matrices.
RPMW | CPMW | |
Mean diameter d50 (μm) | 32.12 | 27.96 |
Carbon (%) | 20.01 | 0 |
Oxygen (%) | 46.32 | 50.38 |
Magnesium (%) | 0.75 | 1.42 |
Calcium (%) | 32.93 | 48.18 |
pH of zero point charge (pHPZC) | 8.06 | 10.43 |
Specific surface area (m2·g−1) | 0.14 | 0.19 |

(Color online.) SEM observations and EDS analyses of RPMW (a, c) and CPMW samples (b, d).
It is important to underline that the RPMW calcination has increased the specific surface area and the pHZPC from 0.14 to 0.19 m2·g−1 and from 8.06 to 10.43, respectively. Since phosphorus removal via adsorption process is favored for pH values lower than pHZPC [26,27], phosphorus removal by CPMW should be more privileged than RPMW for wider aqueous pH intervals.
3.2 Studies of calcium and OH−release from RPMW and CPMW
The experimental results of the blank tests (without phosphorus) regarding calcium and hydronium release by RPMW and CPMW showed that Ca2+ and OH− release kinetics were approximately instantaneous. Indeed, almost one minute was sufficient to attain stable Ca2+ concentrations and aqueous pH values. This equilibrium time is negligible compared to that observed in the case of other solid supports used in the literature such as steel slags [28], aluminum waste [29], and activated oyster shells [30], where these durations were estimated to be about 7 days, 4 h and 40 min, respectively.
As shown in Table 4, at a given initial aqueous pH, the equilibrium release capacities of OH−and Ca2+ are so much important for the CPMW compared to RPMW. For instance, at an initial aqueous pH of 5, the and for CPMW were evaluated at 149.3 and 33.9 mg·g−1, values that were more than 38 and 78 times higher than the ones obtained for RPMW. This result is due to the fact that the dissolution of CPMW in water is more favored than that of RPMW [17]. The RPMW and CPMW dissolution reactions into water are respectively given by:
(6) |
(7) |
Capacity release of OH−and Ca2+ from RPMW and CPMW under different aqueous initial pH.
RPMW | CPMW | |||||
Initial pH | Final pH | (mg·g−1) | (mg·g−1) | Final pH | (mg·g−1) | (mg·g−1) |
5 | 9.45 | 3.90 | 0.479 | 12.27 | 149.33 | 33.92 |
8 | 9.65 | 1.90 | 0.742 | 12.4 | 137.33 | 45.74 |
11 | 9.77 | 1.10 | 0 | 12.56 | 124.00 | 85.43 |
On the other hand, the initial pH values of the aqueous solutions have a significant influence on Ca2+ release from the two studied solid matrixes. In fact, relatively high calcium concentrations were released by CPMW for the acidic tested initial pH compared to the other pH values: rising the initial pH from 5 to 8 and 11 decreases Ca2+ concentrations from 149.3 to 137.3 and 124.0 mg·L−1, respectively. This Ca2+ dissolution in water was accompanied by a net increase in the aqueous pH, which reaches values between 12.27 and 12.40. The presence of relatively high Ca2+ and OH−concentrations would favor the removal of phosphorus through the precipitation of calcium phosphate complexes. For all the tested initial pHs, the capacities of Ca2+ release from RPMW were relatively low. The maximal released concentration did not exceed 4 mg·L−1. Thus, even if the final pH values were relatively important compared to the initial ones, phosphorus removal through a precipitation phenomenon as calcium phosphate compounds should be negligible. However, the presence of Ca2+ can increase the adsorption of phosphate because this cation can form HCO3–Ca–HPO4 bonds at the carbonate sites.
It is important to underline that several studies have proved that CaO dissolution into the aqueous solutions plays an important role in Ca2+ release and in the increase of the corresponding pH. In this regard, Barca et al. [28] have studied phosphorus removal by steel slags collected from 10 production sites in Europe. They demonstrated that for three types of basic oxygen furnace steel slags, the equilibrium duration characterized by a relative stable Ca2+ concentrations and aqueous pH was evaluated at 4–7 days. Furthermore, for a relatively high dosage (40 g·L−1), these slags, which are constituted by about 52.9% of CaO, allowed the release of Ca2+ at concentrations between 128 and 384 mg·L−1. The final pHs of the synthetic solutions were comparable to the ones found in our studies, since they ranged from 12.0 to 12.5.
3.3 Batch experiments for phosphorus removal by RPMW and CPMW from synthetic solutions
Phosphorus removal from synthetic solutions by RPMW and CPMW has been investigated under different experimental conditions: contact time, initial phosphorus concentration, initial aqueous pH, and solid matrixes doses (see Table 1).
3.3.1 Effect of contact time
The experimental results relative to the determination of the impact of the contact time on phosphorus removal by RPMW and CPMW showed that this process is clearly time dependent (Fig. 2). For an initial aqueous phosphorus concentration of 350 mg·L−1, an initial aqueous pH of 5 and solid matrixes dosages of 1 g·L−1, the removed amounts of phosphorus (qt) increased rapidly for contact times lower than 45 and 180 min for CPMW and RPMW, respectively: at these times, they reached about 80% and 77% of the totally adsorbed amount, respectively. For periods greater than 60 and 180 min, the uptake is further increased, but at a slower rate. Since the phosphorus precipitation process with calcium ions is approximately instantaneous (see Section 3.2), this kinetic behavior is certainly linked to the adsorption process. Indeed, at the beginning of the removal experiments, the adsorption rate was relatively rapid because phosphorus ions were mainly adsorbed at the exterior surfaces of RPMW and CPMW. When the adsorption of the exterior surfaces of the used solid matrixes reached saturation, the phosphorus ions entered the related particles and were absorbed with a slower rate by the interior surface of the particles. This finding is in concordance with the ones obtained by Jellali et al. [12] and Karaca et al. [15] when they studied phosphorus removal by phosphate mine slimes and dolomite, respectively.

(Color online.) Effect of contact time on phosphorus removal by RPMW and CPMW (C0,P = 350 mg·L−1; pH = 5; dosage = 1 g·L−1; T = 20 ± 2 °C).
The equilibrium state, characterized by approximate constant removed phosphorus amounts was reached in about 3 and 8 h for CPMW and RPMW, respectively. This difference might be mainly due to the modification of the physicochemical characteristics of CPMW caused by the calcination process. The equilibrium state of these two solid matrixes was faster in comparison with other materials: about 20, 24 and 48 h for wollastonite [31], dolomite [15], and zeolite [32], respectively. Since the equilibrium time is an important parameter for batch assay upscaling (especially energy consumption imputed to agitation), the use of CPMW becomes more interesting than that of RPMW. Furthermore, at the equilibrium state, the phosphorus amount removed by CPMW was evaluated at 181.9 mg·g−1, which is more than 74% higher than the one obtained for RPMW. In this context, Krogstad et al. [33], Willadsen et al. [34], and Johansson and Hylander [35] showed that materials containing CaCO3 removed less phosphorus than substrates with more aggressive CaO or Ca(OH)2 content. Furthermore, the higher is the heating temperature (up to 1000 °C), the higher is the CaO content and phosphorus retention capacity. For instance, Kwon et al. [36] showed that heating oyster shell above 650 °C makes the CaCO3 content fall and increases the phosphorus removal efficiency from 0% to 98%.
3.3.2 Effect of initial phosphorus aqueous concentrations and pH
The experimental results regarding the determination of the effect of the initial aqueous phosphorus concentrations at initial pH values of 5, 8 and 11 for material dosage of 1 g·L−1 for RPMW and CPMW are given in Fig. 3a–b, respectively. At a constant initial pH, the removed phosphorus amounts at equilibrium (qe) increased with increasing the initial phosphorus concentrations. For instance, at an initial aqueous pH of 5, the retention phosphorus capacities rise from about 74.7 to 103.9 mg·g−1 for RPMW and from 120.6 to 181.2 mg·g–1 for CPMW as the initial concentration increased from 150 to 350 mg·L−1. This finding could be explained by the fact that the higher the initial aqueous phosphorus concentration is, the more important is the concentration gradient between the aqueous solution and the solid phase, which results in more important diffusion rates. Furthermore, for the higher initial aqueous concentrations, the contact probability between phosphorus species contained in the aqueous phase and the used porous media might be more privileged [5]. Similar findings were also reported by Karaca et al. [15] and Karageorgiou and al. [37], when they studied phosphorus removal from aqueous solutions by dolomite and calcite, respectively.
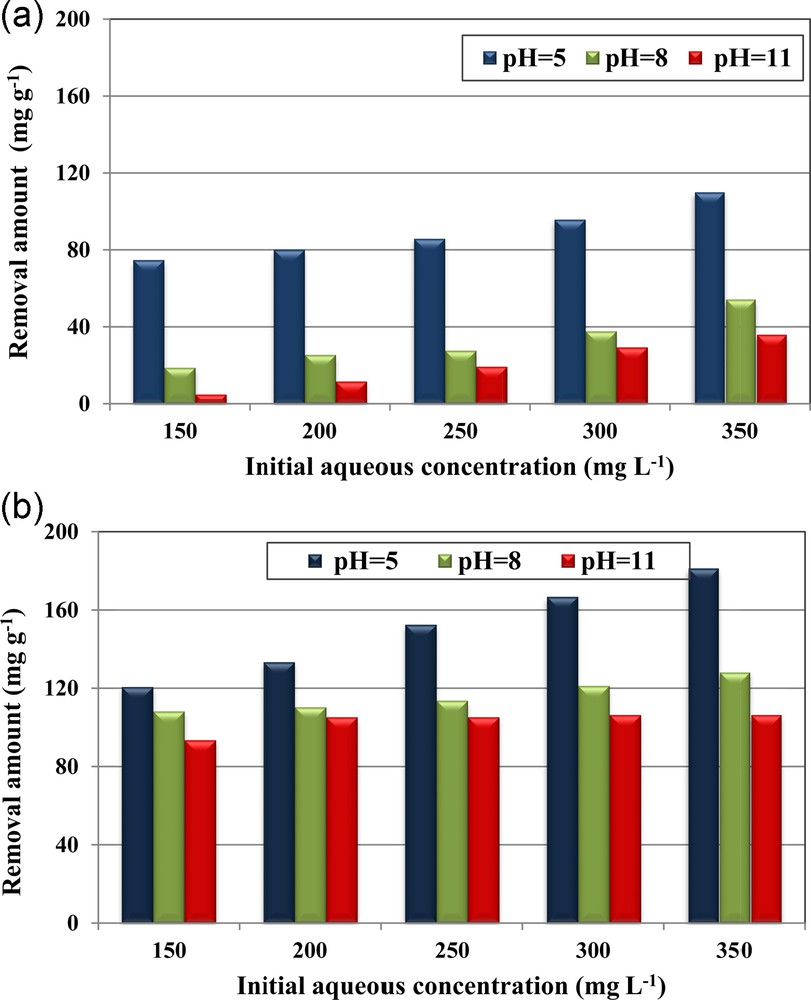
(Color online.) Effect of initial phosphorus aqueous concentrations and pH on phosphorus removal by RPMW (a) and CPMW (b) (dosage = 1 g·L−1; T = 20 ± 2 °C).
For all the used aqueous phosphorus concentrations, it appears that CPMW exhibits relatively higher removal capacities than the RPMW. In fact, the removed phosphorus amounts by CPMW were about 79%, 65%, 57%, 54% and 53% higher than the ones determined for RPMW for initial aqueous concentrations of 150, 200, 250, 300, and 350 mg·L−1, respectively. This outcome might be the result of the combined effects of:
- • the improvements in the structure of CPMW after calcination through the improvements in the structure of CPMW after calcination due to an increase in the specific surface area and in pHZPC values (see Table 3), which favors the retention of phosphorus for a wide range of aqueous pHs;
- • the importance of the precipitation process in the case of CPMW due to the corresponding important calcium ions release compared to RPMW (see Table 4).
Table 5 gives a comparison of RPMW and CPMW capacities in removing phosphorus from aqueous solutions with other natural materials. The main parameters making easier this comparison, such as granulometry and the dosage of the solid matrix, the initial pH and the initial phosphorus concentration, are also included. On the basis of this comparison, it appears that the use of CPMW for phosphorus removal from aqueous solutions can be considered as a promoting technology for not only wastewaters treatment, but also for the reuse of the enriched solid matrix with phosphorus in agriculture as a fertilizer.
Comparison of RPMW and CPMW with other common used materials on phosphorus removal.
Material | Characteristics | Dosage (g·L−1) | Initial pH | Equilibrium time (h) | C0,P (mg·L−1) | Removal capacity (mg·g−1) | Reference |
RPMW | d50 = 32.12 μm Ca (%) = 32.93 Mg (%) = 0.75 | 1 | 5 | 16 | 350 | 103.2 | This study |
CPMW | d50 = 27.96 μm Ca (%) = 48.18 Mg (%) = 1.42 | 1 | 5 | 3 | 350 | 181.2 | This study |
Alunite | SA = 153 m2·g−1 Particle size: 90–710 μm Al2O3 = 22.6% SiO2 = 43.6% K2O = 5.1% | 10 | 5 | 8 | 150 | 40.5 | [38] |
Lanthanum (III) loaded granular ceramic | Particle size: 3–5 mm Si = 33.22% Al = 7.05% Ca = 2.36% Mg = 1.22% | 10 | 7 | 12 | 10 | 0.6 | [39] |
Wollastonite | Particle size: 1–3 mm; Ca = 15.1%; Mg = 1.33%; Fe = 2.15%; Al = 0.55% | 5 | 7.4 | 20 | 1800 | 12.0 | [31] |
Iron hydroxide–eggshell waste | Particle size: 50–315 μm SiO2 = 14% MgO = 0.4% CaCO3 = 98.2% | 7.5 | 7 | 4 | 110 | 8.6 | [40] |
Shell sand | Ca = 31.8%; Mg = 1.46%; Fe = 0.22%; Al = 0.15% | 30 | – | 5–1000 | 3.5 | [41] | |
Raw dolomite | SA = 0.14 m2 g−1 SiO2 = 14% MgO = 0.4% CaCO3 = 98.2% | 2 | 3.45 | 24 | 100 | 47.1 | [15] |
Apatite | Particle size: 0.5–0.9 mm Ca (%) = 37% Mg (%) = 1.6 P (%) = 16.8% | 20 | 7 | 6 | 150 | 4.73 | [42] |
On the other hand, Fig. 3a–b showed that the initial aqueous pH plays an important role in removing phosphorus from aqueous solutions by both RPMW and CPMW. Indeed, the RPMW and CPMW removal capacities significantly decreased when increasing the initial pH. For example, for RPMW, for an initial concentration of 350 mg·L−1, the removed phosphorus amounts decreased from about 110 mg·g−1 at an initial pH of 5 to less than 55 and 36 mg·g−1 for initial pH values of 8 and 11, corresponding to percentage decreases of about 51% and 67%, respectively. The same behavior has been registered for the CPMW, but with a relatively slower decrease. Indeed, for the same initial concentration, the removed phosphorus amount by the CPMW at pH of 5, determined to be 181.1 mg·g−1, has decreased by about 29% and 41% when the initial pH was increased to 8 and 11 respectively. This trend is in concordance with those reported when investigating phosphorus removal by dolomite [15], calcite [26], steel slags [28] and raw and activated red mud and fly ash [43] and arsenic (III) by macrocyclic sulfur compounds [44]. It is primarily due to the fact that when the aqueous pH values were lower than the pHZPC of the used solid matrix, their surface sites were positively charged, which favors the retention of the negatively charged phosphorus ionic species. However, as the pH of the solution is increased, these surface sites become negatively charged, which results in an electrostatic repulsion of the phosphorus anion species. Moreover, when the solution's pH increases, the concentrations of OH− become more important: this makes the retention of phosphorus anion species due to the competition phenomenon significantly decrease. Additionally, as proved in Section 3.2, the released Ca2+ concentrations decreased when increasing the initial pH, which will result in the formation of lower amounts of calcium phosphate precipitates.
It is important to underline that compared to the case of blank solutions (without phosphorus, see Section 3.2), the decrease in phosphorus concentrations, when using CPMW, was accompanied by a significant decrease in pH and calcium ions’ concentrations (Fig. 4a–b). For instance, for a phosphorus concentration of 350 mg·L−1 and initial pH of 5, the Ca2+ concentrations and pH value decreased from 149.3 mg·L−1 and 12.27 (blank test) to 3.9 mg·L−1 and 8.33, respectively. Depending on the instantaneous pH values and calcium concentrations, different Ca–PO4 precipitates may be formed, such as amorphous calcium phosphates (ACPs), dicalcium phosphate (DCP), dicalcium phosphate dihydrate (DCPD), octocalcium phosphate (OCP), tricalcium phosphate (TCP) and the most stable hydroxyapatite (HAP) [45]. Several studies have proved that among these precipitates, the last one seems to be the most probable because of its measured Ca2+ concentrations range and also of the net pH reduction versus time indicating a consumption of OH−ions [28,46–48]. The chemical reaction related to HAP formation is given by:
(8) |
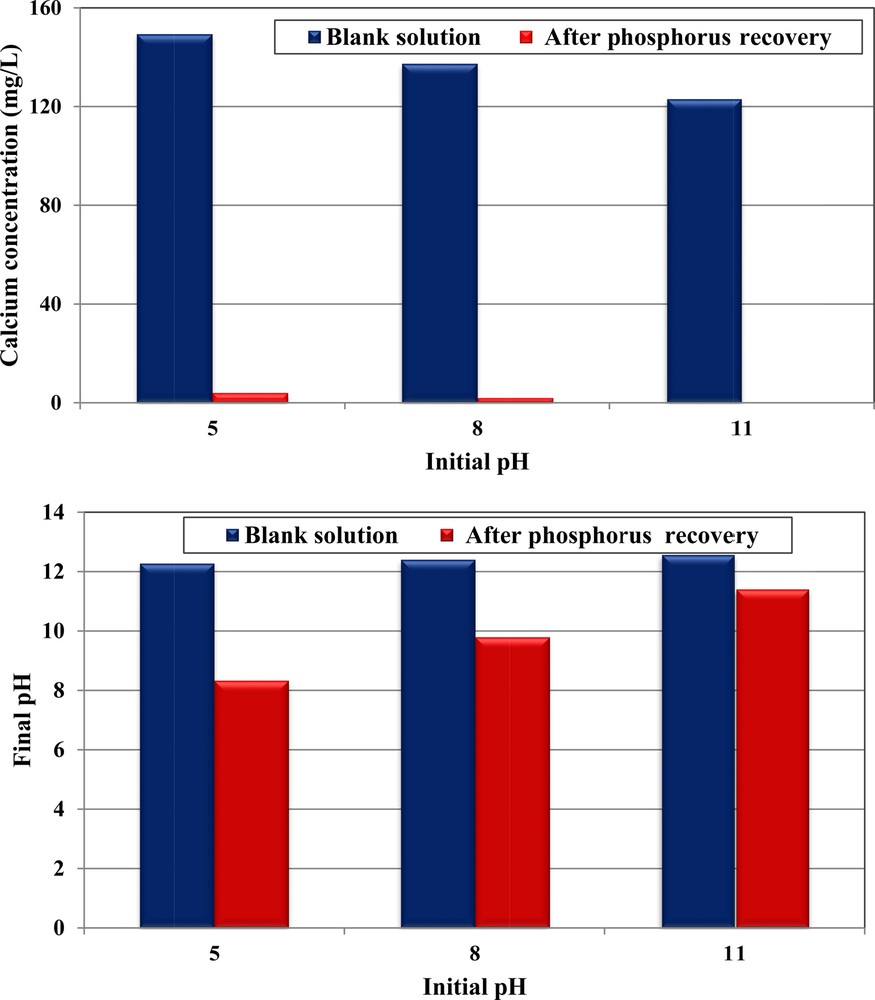
(Color online.) Comparison of calcium concentrations and final pH between blank assays and after phosphorus removal by CPMW (dosage = 1 g·L−1; C0, P = 350 mg·L−1; T = 20 ± 2 °C).
Furthermore, for all the studied initial pHs, the final Ca2+ concentrations were relatively low, suggesting that they were the limiting factor for calcium phosphate precipitation (Fig. 4a). As a consequence, the phosphorus removal efficiencies were more important at acidic pH characterized by the highest Ca2+ release. Indeed, the phosphorus concentrations have decreased from 350 mg·L−1 to about 185.5, 228.6 and 252.4 mg·L−1 for initial pHs of 5, 8, and 11 respectively. The same trend has been registered during the study of phosphorus removal by modified dolomite [15].
The MRs of consumed Ca/P calculated according to Eq. (5) were evaluated to 1.13, 1.41 and 1.60 for initial pH values of 5, 8 and 11, respectively. They were all lower than 1.67, which is the MR relative to hydroxyapatite formation [28,49]. This finding proves that phosphorus removal by CPMW includes not only precipitation as calcium phosphate calcium complexes, but also adsorption onto the surface of the particles. It is important to underline that as the initial aqueous pH increases, the calculated MR become closer to 1.67, which indicates that the contribution of the adsorption phenomenon to the overall removal process becomes weak. This result is in concordance with the studies related to phosphorus removal by steel slags [28].
Concerning RPMW, it is assumed that phosphorus removal was carried out through adsorption, since no significant calcium concentrations were released into the distilled water for all the studied initial pH (see Table 4).
3.3.3 Effect of RPMW and CPMW dosages
The results of the experiments related to phosphorus removal from the synthetic solutions using various dosages of RPMW and CPMW at an initial pH and aqueous concentration of 5 and 350 mg·L−1, respectively, are shown in Fig. 5. It clearly appears that the residual phosphorus concentrations decreased when increasing the dosages of the used solid matrixes. Both CPMW and RPMW were very efficient in removing phosphorus from the relatively highly concentrated aqueous solution (C0 = 350 mg·L−1), since RPMW and CPMW dosages equal to only 4 g·L−1 remove more than 55% and 78%, respectively. Moreover, a complete removal of phosphorus was obtained with relatively low dosages of 14 and 10 g·L−1 of RPMW and CPMW, respectively. Therefore, RPMW and especially CPMW could be considered as attractive materials for phosphorus from aqueous solutions compared to steel slags [28] and apatite [42], where solid dosages of 40 and 20 g·L−1 were needed to ensure significant efficiencies. This may be attributed to the fact that increasing the adsorbent dose provides a greater surface area or more adsorption sites for the phosphorus ions [50] and also allows the CPMW to release important calcium concentrations and OH− in the aqueous phase, which privileges phosphorus removal through calcium phosphates precipitation.
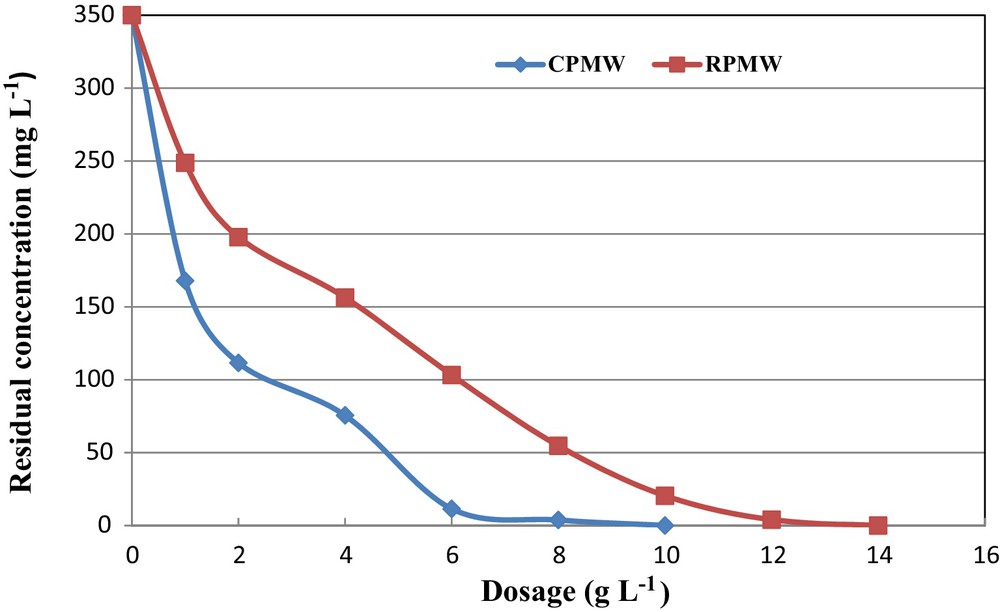
(Color online.) Effect of RPMW and CPMW dosage on phosphorus removal (pH = 5; dosage = 1 g·L−1; T = 20 ± 2 °C).
3.3.4 Phosphorus removal mechanism exploration
The RPMW and CPMW surface before and after phosphorus removal at an initial aqueous pH of 5 were examined using SEM and EDS analyses. The SEM analyses showed that after phosphorus removal, no significant variation in the morphology of RPMW was registered. However, the surfaces of CPMW were covered by crystalline shiny phases that could be imputed to calcium phosphate precipitates (Fig. 6a). On the other hand, EDS analyses of both RPMW and CPMW confirm the existence of the adsorption process, since new peaks corresponding to the phosphorus element have been registered for the two solid matrixes (Fig. 6b–c). Moreover, FTIR analyses of RPMW and CPMW before and after phosphorus adsorption confirm phosphorus removal through an adsorption process by these two solid matrixes (Fig. 6d–e). Indeed, besides the peaks observed at about 3400 relative to OH− band [51] and at 1440 and 875 cm−1 related to CO32−bands [52], some new peaks were registered at about 1220, 1132, 1057, 600, 576 and 526 cm−1. These new peaks are related to PO43−bands [53].


(Color online.) SEM observations of CPMW after phosphorus adsorption (a) and calcium phosphate precipitate (f); EDS analyses after phosphorus removal for CPMW (b); RPMW (c) and the calcium phosphate precipitate (g); FTIR analyses of RPMW (d) and CPMW (e) before and after phosphorus removal and calcium phosphate precipitate (h); DRX analysis of the calcium phosphate precipitate (i).
On the other hand, SEM and EDS analyses of the formed precipitate demonstrated that it is a crystalline phase mainly formed of Ca, P and O, indicating that it is a calcium phosphate species (Fig. 6f–g). XRD and FTIR analyses of this phase prove that it is most probably HAP (Fig. 6h–i) because of their large similarity with the HAP pure phase XRD and FTIR patterns [48,51]. The main related observed peaks corresponding to PO43− bands were located at 1035, 962, 867, 602, 563 and 476 cm−1, respectively.
Thus, for RPMW, phosphorus removal is mainly attributed to the adsorption process, since no significant quantity of calcium ions was released. However, for CPMW, phosphorus removal was the result of the following stages:
- • instantaneous CaO dissolution in the aqueous phase leading to the release of Ca2+ and OH−ions;
- • precipitation of HAP due to the reaction between PO43−ions, Ca2+ and OH−ions;
- • HAP adsorption and/or crystallization onto the CPMW particles;
- • adsorption of PO43−ions after boundary layer diffusion and intraparticular diffusion.
3.4 Batch experiments for phosphorus removal by CPMW from real wastewaters
Phosphorus removal from real wastewater collected from a manufacture of dairy products has been performed using different doses of CPMW, since it has exhibited more efficiency compared to RPMW. Even for a high initial aqueous phosphorus concentration of 1000 mg·L−1, the phosphorus removal efficiency exceeds 65.9% for a CPMW dose of only 1 g·L−1 (Fig. 7). Then, this efficiency increases when increasing the used CPMW doses. In fact, more than 73.7% and 97.0% of phosphorus were removed when using 4 and 10 g·L−1, respectively. The phosphorus removal efficiencies by CPMW from the used wastewater were more important than the one obtained for the synthetic solution (Fig. 7). This finding could be attributed to the existence of relatively high calcium concentrations in the used wastewater (see Table 2). The decrease in phosphorus concentrations in the used wastewater was accompanied by a significant decrease in Ca2+ concentrations and also of the pH values. The final pH solutions after phosphorus removal from the used real wastewater were relatively low compared to the ones obtained for synthetic solutions (C0,P = 1000 mg·L−1; = 0 mg·L−1) and from blank tests (C0,P = = 0 mg·L−1). Indeed, for CPMW doses of 1, 4 and 10 g·L−1, the final pH values of the blank assays were measured at 11.30, 11.95, and 12.22, respectively (Fig. 8). These values decreased to 6.66, 8.3, and 11.77 respectively for the synthetic solutions. However, due to the relatively high initial Ca2+ concentrations into the real wastewaters (see Table 2), the related final pHs were equal to 5.41, 5.32 and 4.96, respectively. This dramatic decrease is mainly linked to the consumption of OH−ions for the formation of HAP. Besides, the residual Ca2+ concentrations in the aqueous phase were relatively important for all the used CPMW, indicating that Ca2+ never limits the formation of the phosphates calcium precipitate (Table 6). This behavior is mainly due to the fact that the Ca2+ contents of the used wastewater (1375 mg·L−1, see Table 2) constitute a further source of Ca2+ ions that significantly contribute to the improvement of phosphorus removal through the precipitation mechanism. The same trend has been pointed out by Barca et al. [28] for phosphorus removal from wastewaters (collected from a small wastewater treatment plant) at initial concentrations of 5–100 mg·L−1 through HAP precipitation with Ca2+ concentrations varying between 28 and 384 mg·L−1. At the end of the assays, in spite of the relatively high residual concentrations of phosphorus and calcium (especially for the low doses), no further precipitation of phosphates calcium complexes was observed. This finding is due to the low final pH of the wastewater (< 5.41).

(Color online.) Phosphorus removal efficiency by CPMW from real wastewater and synthetic solutions (initial pH = 5; C0,P = 1000 mg·L−1; T = 20 ± 2 °C).

(Color online.) Effect of the used CPMW dosage onto the final pH for the wastewater in comparison with blank and synthetic solutions (initial pH = 5; C0,P = 1000 mg·L−1; T = 20 ± 2 °C).
Phosphorus removal efficiencies and molar ratio of removed Ca/P from real wastewater.
Dose (g·L−1) | Residual P (mg·L−1) | Consumed P (mmol) | Initial Ca2+ (mg·L−1) | Residual Ca2+ (mg·L−1) | Consumed Ca2+ (mmol) | Ca/P |
1 | 341.4 | 0.950 | 1524.3 | 912.0 | 1.531 | 1.62 |
4 | 262.5 | 1.060 | 1949.3 | 1112.0 | 2.093 | 1.97 |
10 | 21.6 | 1.407 | 2157.7 | 1230.0 | 2.319 | 1.65 |
The MR of removed Ca2+ to P from the used wastewater was evaluated to 1.62, 1.65 and 1.97 for used CPMW doses of 1, 10 and 4 g·L−1, respectively (Table 6). Because of the theoretical MR of Ca/P for HAP formation is equal to 1.67, the MR lower than this value could be explained by specific phosphorus adsorption onto the CPMW particles. However, MR values higher than 1.67 could be an indicator of the formation of other calcium precipitates, such as CaCO3 [28,54].
4 Conclusions
This study shows that raw and calcined powdered marble wastes can be considered as attractive materials for phosphorus removal from synthetic solutions, especially in acidic media. For RPMW, phosphorus was removed by adsorption onto the surfaces particles. However, for CPMW, due to the release of relatively important calcium ions, precipitation as hydroxyapatite is the predominant mechanism. The use of CPMW for phosphorus removal from a dairy wastewater demonstrates that the Ca2+ contents of the wastewater constitute a further source of Ca2+ that efficiently contribute to the improvement of the phosphorus removal efficiencies. Further investigations are needed with upscaled setups in order:
- • to confirm these promising results;
- • to test the valorization of these enriched solid matrixes as fertilizers in agriculture;
- • to determine the effects of their application as fertilizers onto soils characteristics and plants growth.