1 Introduction
The development of environmentally benign, efficient and economical methods for the synthesis of biologically interesting compounds remains a significant challenge in the synthesis of organic compounds. Multicomponent reactions, via which the products are formed from readily available starting materials in a single step, comply with the principles of green chemistry in terms of economy of steps as well as of their simple experimentation, atom economy and high yields of the products [1].
Heterocyclic compounds are widely distributed in nature and are essential to life. Benzothiazole derivatives are important classes of heterocyclic compounds that occur widely in the pharmaceutical industry. For example, compounds containing a 2-aminobenzothiazole motif have attracted an interest because they exhibit a wide range of biological activities such as antifungal [2], anti-infective and herbicidal [3], antimalarial [4], anti-tubercular [5], antimicrobial [6], and anticancer [7] ones. Additionally, they are applied in coordination chemistry as Schiff bases [8,9]. Among them, 1-(benzothiazolylamino)phenylmethyl-2-naphthols have two biologically active parts, 2-aminobenzothiazole and Betti's base. Betti's base derivatives [1-(α-aminoalkyl)-2-naphthols] have provided convenient access to many useful synthetic building blocks via the amino and phenolic hydroxy functional groups [10]. The preparation of 1-(benzothiazolylamino)phenylmethyl-2-naphthols is based on the three-component condensation reaction of an aldehyde, 2-aminobenzothiazole and 2-naphthol. For this purpose, several catalysts, including LiCl [11], sodium dodecyl sulfate (SDS) [12], HPA [13], NaHSO4.H2O [14], trichloroisocyanuric acid (TCCA) [15], 3-methyl-1-(4-sulfonic acid)propylimidazolium hydrogen sulfate ([(CH2)3SO3HMIM][HSO4]) [16] have been used to facilitate this reaction.
Over the last years, ionic liquids (ILs) have been extensively used in almost all application fields of chemistry such as organic and inorganic syntheses, catalysis, electrochemistry, and chromatography. It is due to their remarkable properties such as non-flammability, negligible vapor pressure, wide liquid range and high thermal, chemical and electrochemical stability. The use of Brønsted acidic ionic liquids as catalysts or in the dual role of catalysts and solvents has also brought major improvements in many organic reactions. However, their high cost, large consumption and difficult recovery as well as the separation of products cause limitations in their large-scale application. Furthermore, ionic liquids (especially Brønsted acidic ionic liquids) have some degree of instability in the presence of air and moisture. Immobilization of ionic liquids on the surface of a solid support is a useful way to combine the advantageous characteristics of ionic liquids and solid properties. In other words, the immobilized ionic liquids offer additional features compared to pure ionic liquids that facilitate handling, separation and reuse procedures, and minimize the amount of IL utilized in reactions [17].
The utilization of waste materials is a very desirable goal for green chemistry in order to keep a green environment. Rice husk, the outer covering of rice grains that are obtained during the milling process, is one of the main agricultural residues. Societies often dispose of rice husk waste using open burning, which leads to environmental pollution and brings damages to the land and to the surrounding area in which it was dumped. The application of rice husk as an energy source for biomass power plants, rice mills and brick factories is increasing due to its high calorific power [18]. In this combustion, rice husk ash (RHA) is produced. RHA has a light weight; therefore, the disposal of bulky RHA could be a problem. RHA contains considerable amount of amorphous silica (up to 80%) and a small proportion of impurities such as K2O, Na2O, and Fe2O3 [19]. Therefore, RHA possesses high silica content, so it has been employed to produce zeolites and silica powders [20,21].
Several papers have been published on the application of the modified amorphous silica obtained from rice husk ash as the catalyst [22–24]. Although useful, the reported method for the preparation of pure silica from rice husk ash needs various stages and a long time [20]. On the basis of these points and our investigations, we concluded that, in the same conditions, the use of RHA as a support for the preparation of the catalysts is better than the use of silica, which is prepared via a precipitation method comprised of various stages and requiring a lot of time [25,26].
2 Experimental
2.1 General
Chemicals were purchased from Fluka, Merck, and Aldrich chemical companies. All yields refer to the isolated products. Products were characterized by comparison of their physical constants, IR and NMR spectroscopic data with those of authentic samples and others reported in the literature. The purity determination of the substrate and reaction monitoring were accompanied by TLC on silica gel polygram SILG/UV 254 plates.
2.2 Instrumentation
The 1H NMR and 13C NMR were run on a 400 MHz Bruker Avance in DMSO-d6 using TMS as an internal standard. The FT-IR spectra were run on Alpha FT-IR spectrometer, Bruker company (Germany). Our thermogravimetric analyses (TGA) were performed on Polymer Laboratories PL-TGA thermal analysis instrument (England). The samples were heated from 25 to 600 °C at a ramp of 10 °C/min under an N2 atmosphere. Scanning election microphotographs (SEM) were obtained on a SEM-Philips XL30. X-ray diffraction (XRD) measurements were performed at room temperature on diffractometer Model XRD 6000, PHILIPS Xpert pro using Co Kα radiation (λ = 1.7890 Å) with a beam voltage and a beam current of 40 kV and 30 mA, respectively.
2.3 Preparation of 1-methyl-3-(trimethoxysilylpropyl)-imidazolium chloride ([pmim]Cl)
A mixture of 10 mmol of 1-methylimidazole and 10 mmol (3-chloropropyl)trimethoxysilane was refluxed at 90 °C for 30 h. Then, the reaction mixture was cooled down. The crude product was washed with Et2O (2 × 5 mL) and dried under vacuum. The obtained product was a slightly yellow viscous oil.
2.4 Preparation of 1-methyl-3-(trimethoxysilylpropyl)-imidazolium chloride supported on RHA (RHA-[pmim]Cl)
Was dissolved 0.6 g (2 mmol) of [pmim]Cl in 25 mL of CH2Cl2 and treated with 2 g of RHA (used in our previous report [25]). The reaction mixture was refluxed under stirring for 3 days. Then, the reaction mixture was cooled to room temperature, the solid was isolated by filtration and washed with 20 mL of boiling dichloromethane to remove the unreacted ionic liquid. In the next step, the material was dried to give 2.5 g RHA-[pmim]Cl as a gray powder.
2.5 Preparation of 1-methyl-3-(trimethoxysilylpropyl)-imidazolium hydrogen sulfate supported on RHA (RHA-[pmim]HSO4)
Three grams of RHA-[pmim]Cl were suspended in 20 mL of dry CH2Cl2. Under vigorous stirring, 2.9 mmol of concentrated H2SO4 (97%) were added dropwise in an ice bath (0 °C). The mixture was then warmed to room temperature and heated under reflux for 30 h. When the formed HCl was completely distilled off, the solution was cooled and CH2Cl2 was removed under vacuum to afford RHA-[pmim]HSO4 (3.16 g) as the product (Scheme 1).
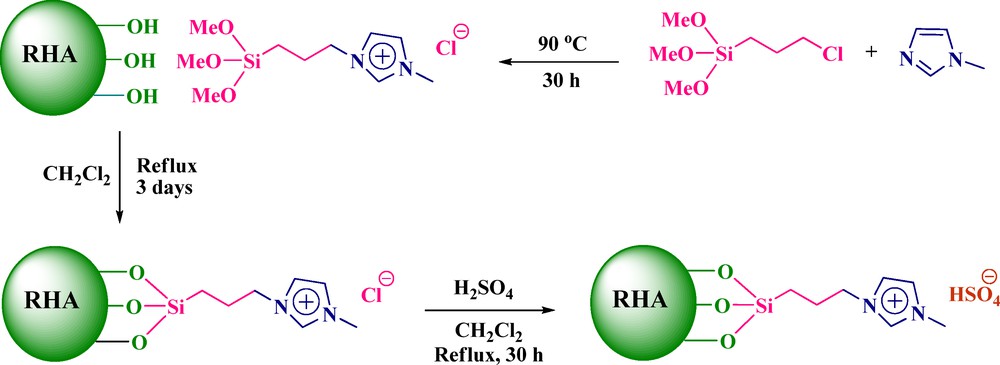
(Color online.) Preparation of the acidic ionic liquid supported on rice husk ash (RHA) (RHA-[pmim]HSO4).
2.6 General procedure for the preparation of 1-(benzothiazolylamino)phenylmethyl-2-naphthols
A mixture of the aldehyde (1 mmol), 2-aminobenzothiazole (1 mmol), 2-naphthol (1 mmol) and RHA-[pmim]HSO4 (150 mg) was heated at 100 °C under solvent-free conditions for the appropriate time (Table 1). After completion of the reaction (monitored by TLC), ethyl acetate (10 mL) was added and the catalyst was separated by filtration. The solvent was evaporated, and the products were purified by recrystallization from aqueous ethanol. The spectral data of new compounds are as follows.
Preparation of 1-(benzothiazolylamino)phenylmethyl-2-naphthol derivatives catalyzed by RHA-[pmim]HSO4under solvent-free conditions.
Entry | Aldehyde | Time (min) | Yield (%) | Melting point (°C) | |
Found | Reported [Ref.] | ||||
1 | C6H5– | 4 | 93 | 201–203 | 202–204 [16] |
2 | 2-Cl–C6H4– | 5 | 89 | 188–190 | 187–189 [16] |
3 | 3-Br–C6H4– | 3 | 93 | 203–205 | 203–205 [16] |
4 | 4-Cl–C6H4– | 3 | 92 | 208–210 | 209–210 [11] |
5 | 4-Br–C6H4– | 3 | 91 | 211–213 | 200–202 [16] |
6 | 3-CH3–C6H4– | 5 | 93 | 189–191 | – |
7 | 2-OCH3-C6H4– | 3 | 90 | 170–172 | 168–170 [16] |
8 | 3-CH3O–C6H4– | 5 | 92 | 184–186 | 184–186 [16] |
9 | 4-CH3O–C6H4– | 4 | 93 | 172–174 | 172–173 [15] |
10 | 2-NO2–C6H4– | 5 | 90 | 218–220 | 215–216 [16] |
11 | 3-NO2–C6H4– | 5 | 92 | 190–192 | 191–194 [12] |
12 | 4-NO2–C6H4– | 4 | 91 | 186–188 | 187–189 [16] |
13 | 4 | 94 | 197–199 | – | |
14 | 4 | 90 | 195–197 | – | |
15 | 4 | 89 | 209–211 | 210–212 [16] | |
16 | 5 | 87 | 215–217 | – |
Table 1, entry 6: IR (neat) ν = 3310, 1599, 1545, 1510, 1449 cm−1; 1H NMR (DMSO-d6, 400 MHz): δ = 2.22 (s, 3H), 7.02–7.80 (m, 15H), 8.80 (s, 1H), 10.15 (br, 1H) ppm; 13C NMR (DMSO-d6, 100 MHz): δ = 21.7, 119.3, 121.4, 121.4, 122.9, 123.7, 123.7, 125.9, 126.0, 126.8, 126.9, 127.1, 127.4, 127.5, 128.4, 128.5, 129.1, 130.0, 131.2, 132.6, 137.5, 143.0, 152.6, 153.6, 166.8 ppm.
Table 1, entry 13: IR (neat) ν = 3300, 1620, 1595, 1539, 1502, 1445 cm−1; 1H NMR (DMSO-d6, 400 MHz): δ = 7.02–7.95 (m, 18H), 8.99 (s, 1H), 10.24 (s, 1H) ppm; 13C NMR (DMSO-d6, 100 MHz): δ = 118.6, 119.0, 119.5, 121.5, 121.6, 122.9, 124.4, 125.5, 125.9, 126.02, 126.6, 126.78, 126.80, 127.9, 128.17, 128.22, 129.09, 129.14, 130.2, 131.2, 132.3, 132.7, 133.2, 140.7, 152.4, 153.8, 166.9 ppm.
Table 1, entry 14: IR (neat) ν = 3310, 1620, 1593, 1535, 1502, 1440 cm−1; 1H NMR (DMSO-d6, 400 MHz): δ = 3.81 (d, J = 22.81 Hz, 1H), 3.88 (d, J = 22.81 Hz, 1H), 7.05–7.97 (m, 18H), 8.88 (s, 1H), 10.21 (s, 1H) ppm; 13C NMR (DMSO-d6, 100 MHz): δ = 53.8, 118.6, 118.9, 119.0, 119.4, 120.1, 120.2, 121.4, 121.5, 122.9, 123.2, 125.3, 125.5, 125.9, 126.8, 127.0, 127.2, 127.6, 129.1, 129.1, 130.1, 131.3, 132.7, 139.9, 141.3, 142.0, 152.6, 153.7, 166.8 ppm.
Table 1, entry 16: IR (neat) ν = 3350, 1599, 1540, 1518, 1445 cm−1; 1H NMR (DMSO-d6, 400 MHz): δ = 7.24–7.77 (m, 26H), 8.81 (s, 2H), 10.14 (br, 2H) ppm; 13C NMR (DMSO-d6, 100 MHz): δ = 118.1, 118.5, 118.8, 119.0, 121.3, 121.4, 122.8, 125.9, 125.9, 126.4, 127.2, 129.0, 129.9, 130.0, 131.2, 132.5, 140.8, 152.5, 153.6, 153.6, 166.7 ppm.
2.7 Characterization of the catalysts
2.7.1 FT-IR analysis
The infrared spectra of RHA and RHA-[pmim]HSO4 are shown in Fig. 1. In the case of RHA, the peaks at 3450 and 1635 cm−1 are respectively attributed to the stretching and bending modes of the SiOH groups and of the adsorbed water. The strong peaks at 1100, 800 and 467 cm−1 are assigned to the asymmetric stretching, the symmetric stretching and the bending modes of SiO2, respectively [20].
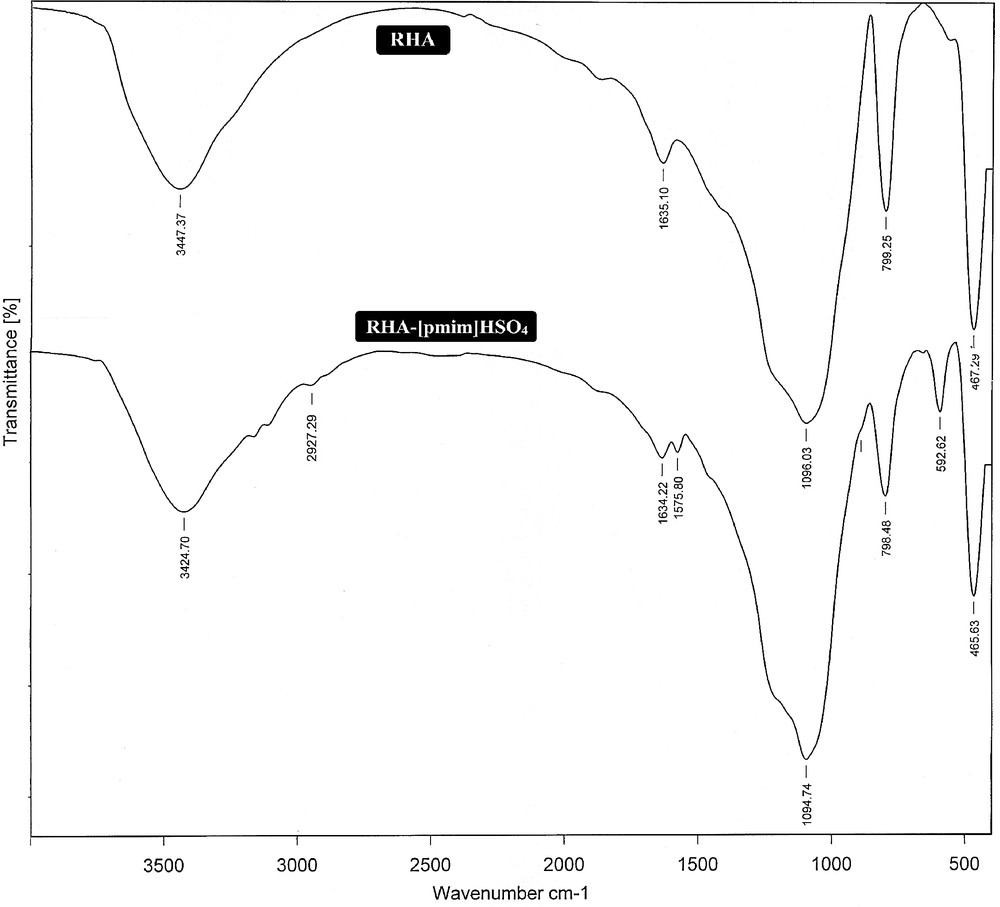
Infrared spectroscopy (FT-IR) spectra of rice husk ash (RHA), RHA-[pmim]Cl and RHA-[pmim]HSO4.
RHA-[pmim]HSO4 catalysts were also characterized on the basis of their FT-IR data (Fig. 1). The peaks at 1575 cm−1 can be assigned to the CC band of the imidazolium ring attached to a silica surface. Additional bands at 3160 and 2927 cm−1 were due to CH stretching and deformation vibrations, confirming the functionalization of the material with the ionic liquid [27]. The absorption of the SO stretching modes lies between 1170 and 1060 cm−1, so the broad band around 1100 cm−1 can be assigned to the stretching modes of SiO and SO bands, which are overlapped together. The SO stretching mode of the functional group of sulfuric acid lies around 592 cm−1, proving the successful preparation of the catalyst [28].
2.7.2 Powder X-ray diffraction
Fig. 2 represents the X-ray diffraction (XRD) patterns of the RHA and RHA-[pmim]HSO4. As it can be seen in the RHA pattern, a broad peak that appeared around 2θ, equal to 22°, clearly indicates that the silica of rice husk ash was mainly in amorphous form [29]. The peak intensities of RHA-[pmim]HSO4 remained almost unchanged compared to the RHA pattern, which indicates an ordered mesoporosity of the support, even after modification.
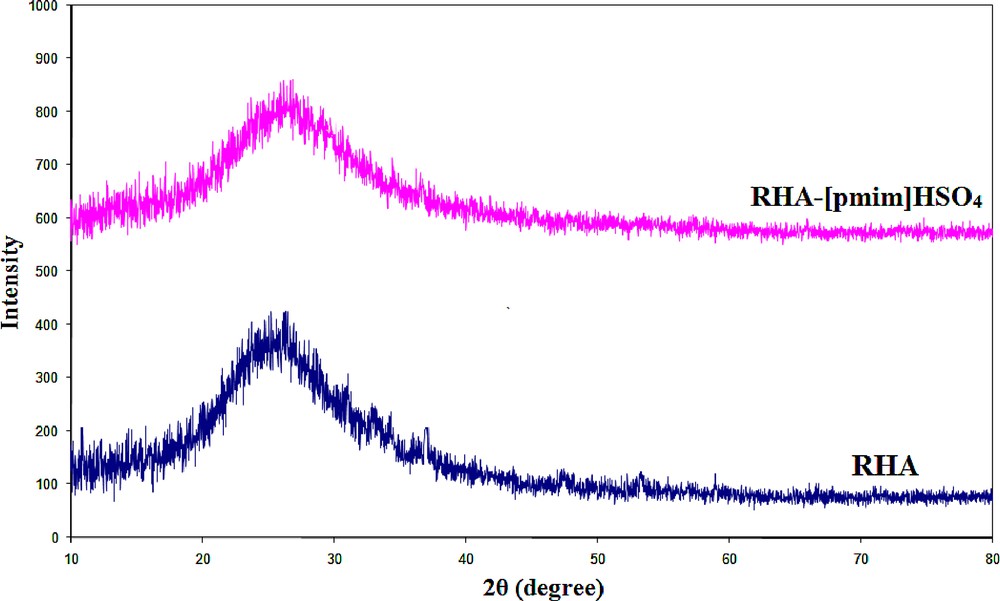
(Color online.) X-ray diffraction (XRD) patterns of rice husk ash (RHA) in comparison of RHA-[pmim]HSO4.
2.7.3 SEM analysis
The samples of RHA and RHA-[pmim]HSO4 were analyzed by scanning electron microscopy (SEM) with ×30,000 and 50,000 magnifications for determining the size distribution, particle shape and surface morphology, as represented in Fig. 3. The pictures show that RHA has a porous and irregular shape [29]. These images also show that the primary morphology of RHA is changed after modification with the ionic liquid. Indeed, the comparison of RHA and RHA-[pmim]HSO4 clearly indicated that the particles are aggregated after modification.
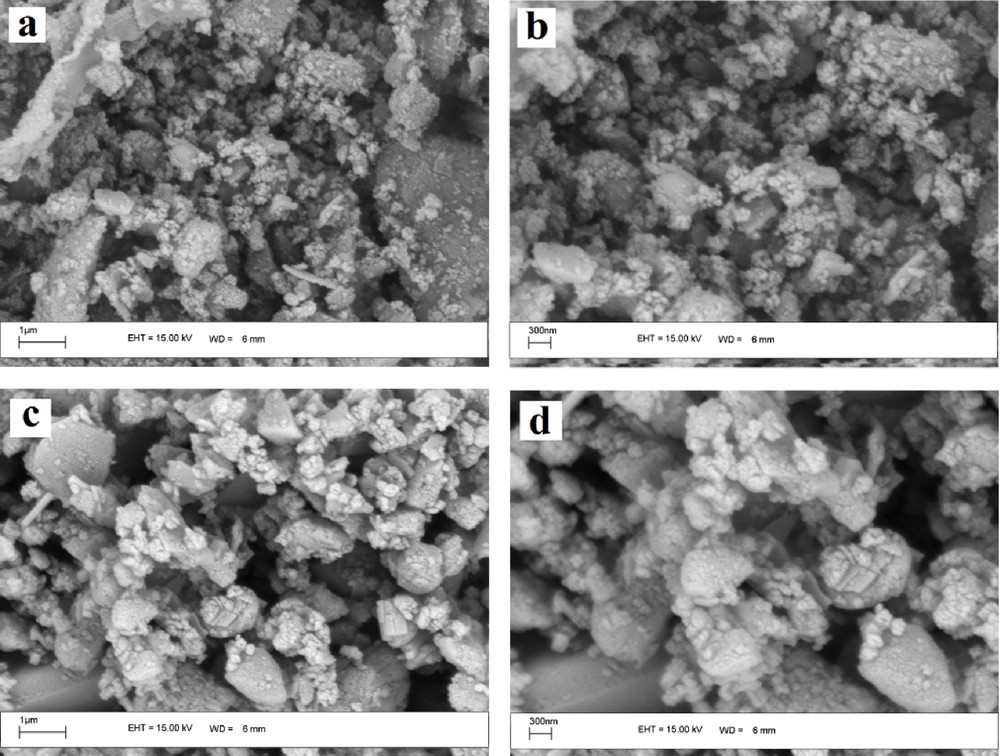
Scanning electron microscope (SEM) images of rice husk ash (RHA) and RHA-[pmim]HSO4. RHA (a) ×30,000 and (b) ×50,000 magnifications and RHA-[pmim]HSO4 (c) ×30,000 and (d) ×50,000 magnifications.
2.7.4 Thermal analysis
The thermal stability of the samples was investigated by thermogravimetric analysis (TGA) in which the observed weight loss was associated with the loss of the organic components attached to the RHA (Fig. 4). The results indicate that RHA showed a weight change below 100 °C due to the loss of physically adsorbed water and then had a steady weight loss at a temperature lower than 600 °C, which could be ascribed to the loss of terminal groups such as OH terminal groups [25].
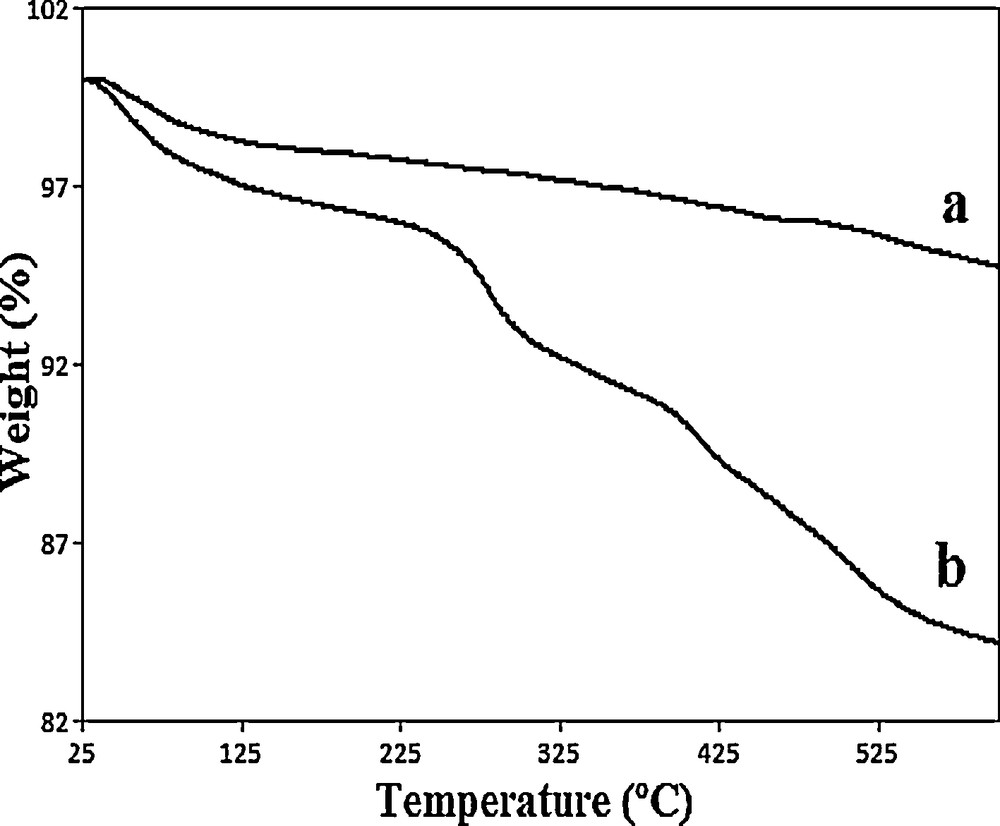
Trichloroisocyanuric acid (TGA) curves of rice husk ash (RHA) (a) and RHA-[pmim]HSO4 (b).
After immobilization of the ionic liquid, the weight loss increased, which was due to the further increase of the organic components on the RHA. The TGA analysis of RHA-[pmim]HSO4 shows a completely different decomposition type from that of RHA. The first step appeared at < 150 °C because of the loss of water and the second weight loss that started at 230 °C can be attributed to the decomposition of the immobilized ionic liquid moieties. Complete loss of all the covalently attached organic structure is seen in the temperature range between 230 and 570 °C [30]. These results indicate that the immobilized acid catalyst is apparently stable up to about 230 °C. Furthermore, the tendency of the absorption of water on the surface of the catalyst is increased after immobilization of the Brønsted acidic ionic liquid.
2.7.5 pH analysis of the catalyst
For pH analysis of the catalyst, to 25 mL of an aqueous solution of NaCl (1 M) with a primary pH 5.2, RHA-[pmim]HSO4 (0.5 g) was added and the resulting mixture was stirred for 2 h at room temperature, after which the pH of the solution decreased to 1.8. This is equal to a loading of 0.8 mmol H+/g [28].
3 Results and discussion
On the basis of the information obtained from the studies on RHA-[pmim]HSO4, we anticipated that this reagent can be used as an efficient solid acid catalyst for the promotion of the reactions that need the use of an acidic catalyst to speed up. So, we were interested in investigating the applicability of this reagent in the promotion of the synthesis of 1-(benzothiazolylamino)-phenylmethyl-2-naphthols.
At first and for optimization of the reaction conditions, the reaction of 4-chloro benzaldehyde, 2-aminobenzothiazole and 2-naphthol to its corresponding 1-(benzothiazolylamino)-phenylmethyl-2-naphthols was selected as a model reaction in various conditions. For choosing the reaction media, different solvents such as dichloromethane, acetonitrile, ethanol, and water, and also solvent-free conditions were used, and the best results were obtained in solvent-free conditions at 100 °C. Subsequently, the effect of the amount of RHA-[pmim]HSO4 on the reaction was investigated. It was observed that an amount of 150 mg of RHA-[pmim]HSO4 was the optimal catalyst loading for this reaction, and higher catalyst loading did not improve the yield of the product to a greater extent. Finally, the best result was obtained using 150 mg (12 mol%) of RHA-[pmim]HSO4 at 100 °C under solvent-free conditions (Scheme 2).

(Color online.) Synthesis of 1-(benzothiazolylamino)phenylmethyl-2-naphthol derivatives in the presence of rice husk ash (RHA)-[pmim]HSO4.
After optimization of the reaction conditions and in order to establish the effectiveness and the acceptability of the method, we explored the protocol with a variety of aromatic, aliphatic and heterocyclic aldehydes under the optimal conditions. The results are presented in Table 1. It was observed that under similar conditions, a wide range of aromatic aldehydes containing electron-withdrawing as well as electron-donating groups such as Cl, Br, CH3, OCH3, and NO2 in ortho-, meta-, and para-positions of the benzene ring easily were converted to the corresponding products in short reaction times with high isolated yields (Table 1, entries 1–12). As can be seen, the effect of the nature of the substituents on the aromatic ring did not show strongly obvious effects in terms of yields and reaction times under the selected reaction conditions. Furthermore, polycyclic aromatic aldehydes such as 2-naphthaldehyde and fluorene-3-carbaldehyde also provided the desired product in very good yields (Table 1, entries 13–14). Pyridine-4-carbaldehyde as a heterocyclic aldehyde was also used as a substrate in these conditions, and the desired product was successfully obtained with high yields (Table 1, entry 15).
The proposed mechanism for the synthesis of 1-(benzothiazolylamino)phenylmethyl-2-naphthol in the presence of RHA-[pmim]HSO4 as a promoter is shown in Scheme 3. On the basis of this mechanism, RHA-SO3H catalyzes the reaction by the activation of the carbonyl groups against the nucleophilic attack.

Proposed mechanism for the synthesis of 1-(benzothiazolylamino)phenylmethyl-2-naphthol derivatives catalyzed by rice husk ash (RHA)-[pmim]HSO4.
To check the reusability of the catalyst, the reaction of 4-chloro benzaldehyde, 2-aminobenzothiazole and 2-naphthol under the optimized reaction conditions was studied again. When the reaction was completed, ethyl acetate was added and the catalyst was separated by filtration. The recovered catalyst was washed with ethyl acetate, dried and reused for the same reaction. This process was carried out over six runs and all reactions led to the desired products without significant changes in terms of reaction time and yield, which clearly demonstrates the practical recyclability of this catalyst (Fig. 5).
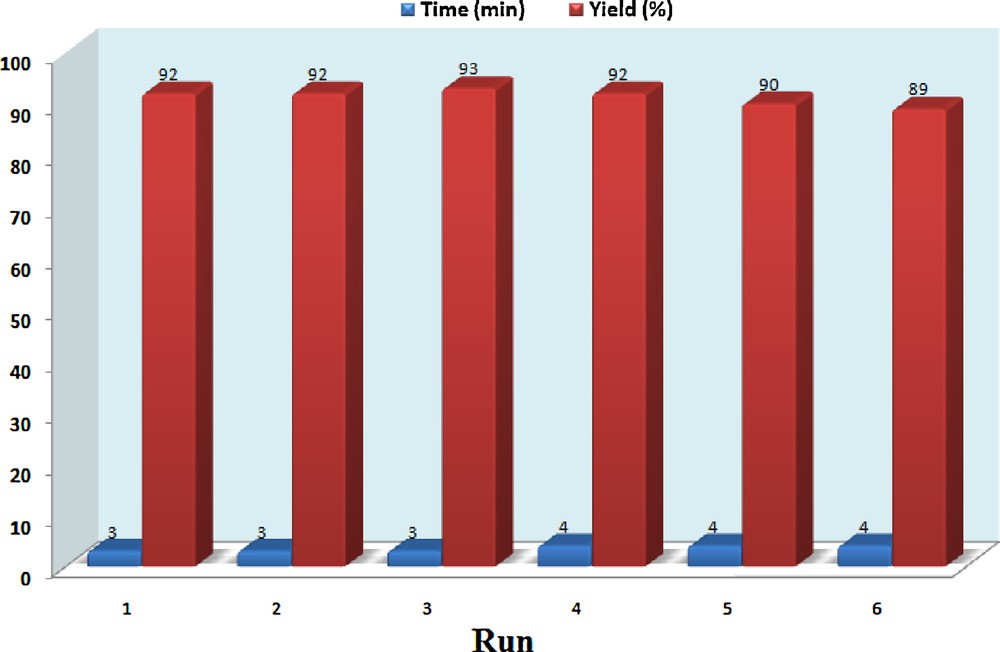
(Color online.) Reusability of the catalyst.
In order to show the efficiency of the present method, we have compared our results obtained from the reactions of 3-nitro benzaldehyde, 2-aminobenzothiazole and 2-naphthol to produce 2′-aminobenzothiazolo-(4-nitro-phenyl)-methyl-2-naphthol catalyzed by RHA-[pmim]HSO4 with other results reported in the literature (Table 2). It is clear that the present method is superior in terms of reaction time and yield. Furthermore, to compare the applicability and efficiency of RHA-[pmim]HSO4 with that of the other catalysts, we have calculated the TOF of these catalysts in these reactions. As it is clear, the TOF values showed that RHA-[pmim]HSO4 is more effective than the compared catalysts.
Comparison of the results of the reaction between 3-nitro benzaldehyde, 2-aminobenzothiazole and 2-naphthol catalyzed by RHA-[pmim]HSO4 with those obtained by some of the reported catalysts.
Entry | Catalyst | Catalyst | Reaction conditions | Time (min) | Yield (%) | TOF (h−1)a | Ref. |
1 | LiCl | 71 mmol | 90 °C/H2O | 6 h | 91 | 0.002 | [11] |
2 | HPA | 3 mol% | 60 °C/neat | 5.2 h | 86 | 5.5 | [13] |
3 | NaHSO4.H2O | 10 mol% | 100 °C/neat | 30 | 59 | 12 | [14] |
4 | TCCA | 10 mol% | 80 °C/neat | 40 | 90 | 13 | [15] |
5 | [(CH2)3SO3HMIM]HSO4 | 10 mol% | 100 °C/neat | 30 | 55 | 11 | [16] |
6 | RHA-[pmim]HSO4 | 12 mol% | 100 °C/neat | 5 | 92 | 92 | This work |
a Turn over frequency (TOF) represents the average number of substrate molecules converted into the product per molecule of catalyst per hour.
4 Conclusions
In conclusion, in this study we have introduced RHA-[pmim]HSO4 as a highly powerful supported acidic ionic liquid for the simple and efficient synthesis of 1-(benzothiazolylamino)phenylmethyl-2-naphthol derivatives. The procedure gave the products in excellent yields in very short reaction times with high TOF. The catalyst shows high thermal stability and was recovered and reused without any noticeable loss of activity. Furthermore, the ease of preparation and handling of the catalyst, the simple experimental procedure, the use of an inexpensive and reusable catalyst and solvent-free conditions are other advantages of this method. Further studies on some more practical applications of the RHA-[pmim]HSO4 catalyst in other organic reactions are currently underway in our laboratory.
Acknowledgments
We are thankful to the Research Council of the University of Guilan for partial support of this work.