1 Introduction
Textile industry is an important water pollutant source in developing countries since its discharged wastewater could contain persistent organic dyes and also toxic by-products. The presence of these pollutants in water bodies generally cause serious public concern regarding human and aquatic organisms health, fauna and flora growth due to their toxic, mutagenic or carcinogenic properties [1,2]. Indeed, even very low concentrations of dyes in water (less than 1 ppm for some dyes) are highly visible and not recommended [3]. Methylene blue (MB) is the most commonly used substance for cotton, wood, and silk dyeing. The exposition to MB causes serious injuries to human and animals’ eyes and in some cases could lead to mental confusion and methemoglobinemia [3]. Thus, appropriate treatment of wastewaters containing such dyes has recently drawn increasing scientific attention in order to reduce their negative impacts onto both human health and ecosystems’ biodiversity and to favor their reuse [4].
Various treatment processes have been applied for dye removal from wastewaters such as biological degradation [5], coagulation–flocculation [6], Fenton's oxidation [7], membrane separation [8], ozonation [9]. However, some of these technologies are confronted with the production of large amounts of sludge and are not sufficiently efficient. The others have the drawback of high cost operation, which limits their full-scale application [7]. Therefore, in the course of this last decade, considerable interest has been paid to developing innovative and green technologies such as anodic oxidation (AO) and adsorption onto natural and inexpensive materials.
Anodic oxidation is considered as a friendly environment technology using innovative materials. It is mainly based on the generation of highly reactive hydroxyl radicals (HO.) having high thermodynamic standard potential equal to 2.38 V [10]. Besides, additional oxidizing powerful agents can be electrogenerated with different electrolytes supports (active chlorine, persulfates, etc.) [11]. Several anodes have been tested for dye removal from effluents such as graphite, platinum, doped and undoped PbO2, and recently boron-doped diamond electrodes (BDDs). The latter, which constitutes polycrystalline diamond layers synthesized on substrate such as silicon through microwave plasma enhanced chemical vapor, exhibits many advantages such as chemical resistance, high lifetime, and especially interesting removal efficiencies and non-selectivity of the dyes [12,13]. However, the achievement of high removal performances of dyes from effluents is essentially dependent on electrolyte matrix [11], pH and electrolysis time [11,14,15], and generally requires important energy consumption and sometimes produces very toxic intermediate by-products [16]. Furthermore, studies on kinetic dyes’ removal by AO demonstrated that the corresponding removal rates were relatively important at the beginning of the experiments, and then they were sensibly reduced versus time [4,13,14] as they are fitting an apparent first-order kinetic in general. Thus, important energy saving could be performed if AO is used only as a pretreatment of dyes containing wastewaters followed by another low-cost technology.
On the other hand, adsorption, which is a well-known technology, has been identified as an attractive method for removing dyes from effluents. Compared to the other common methods, adsorption has the advantages of low cost, flexibility, and design simplicity. Moreover, adsorption does not result in the formation of toxic intermediate substances. The main used adsorbents for dye removal from aqueous solutions (either organic or inorganic) included activated carbon [17], natural materials such as clays [18] and zeolite [19], and waste materials and by-products from agriculture and industry, such as cotton waste [20], olive pomace [21], sewage sludge [22], and fly ash [23]. One of the most promising categories of organic materials is the lignocellulosic waste materials group, which is available in abundance, renewable and cheap. Sawdust is one of these wastes, and has been already widely used for the removal of pollutants from wastewater, such as dyes, ammonium, oils, salts, heavy metals [24–28]. One of the main advantages of sawdust is its low cost, since it requires minimal processing and is an abundant forestry residue [29]. The main factors influencing dyes sorption onto sawdust are [25,30] the dye chemical structure and its concentration in the effluent, the origin and the dose of the sawdust as well as its pretreatment and physical characteristics, the physicochemical characteristics of the wastewaters (pH, ionic strength, and temperature). However, to achieve complete dye removal, relatively huge amounts of sawdust are required. The resulting polluted sawdust present a sludge disposal problem if they are not regenerated with chemical reagents [24,30].
In this regard, a combination of AO followed by adsorption onto sawdust may overcome their respective limitations and synergize the advantages of their dye removal efficiencies. The success of such approach would permit an important economic gain and a net reduction of the used sawdust amounts to be discharged or regenerated.
The main goals of this research work were to investigate:
- • the color and COD removal and the kinetic of MB degradation by AO under different aqueous initial pH;
- • the main parameters affecting MB sorption onto sawdust;
- • the effectiveness of the combination of AO followed by sorption in removing MB and the related economic gain and reduction of the needed sawdust amounts.
2 Material and methods
2.1 MB solutions preparation and analysis
Methylene blue (C16H18ClN3S, MW = 319.852 g·mol−1, purity = 98%), acquired from Fisher Scientific, was used during AO and adsorption batch tests (Fig. 1). A stock MB solution of 1000 mg·L−1 was prepared with distilled water and used throughout this study for the preparation of the desired solutions having specific physicochemical characteristics. The MB color removal was evaluated by measuring the absorbance decrease at 664 nm by using an UV-visibe spectrometer apparatus (Thermo Spectronic UV1). In addition, the related chemical oxygen demands (COD) were determined according to the dichromate standard method [31]. Each analysis point reported in this study was an average of at least three independent parallel sample solutions. The standard deviation of the results was ± 3%.
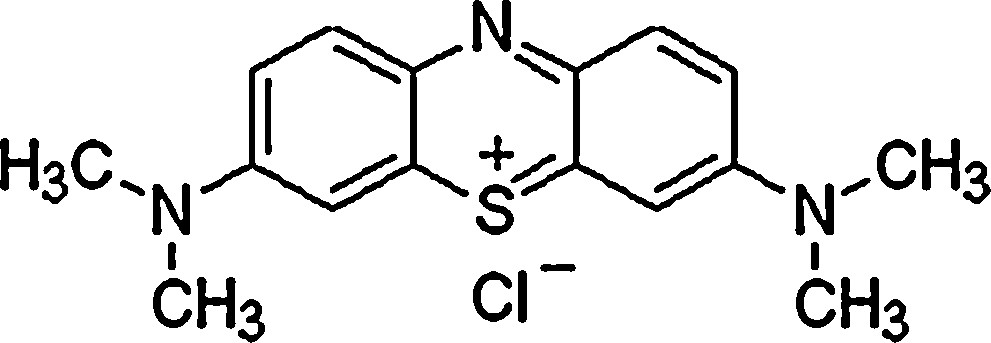
Molecular structure of methylene blue.
2.2 Sawdust preparation and properties
Eucalyptus globulus sawdust was obtained from a local sawmill in Beja (northwestern Tunisia). It was firstly washed thoroughly with distilled water in order to remove impurities. Then, it was air-dried for several days. The physicochemical characterization of sawdust has demonstrated that its BET specific surface was relatively low and equal to 1.43 m2·g−1 [26]. However, it has high relatively important exchangeable cations. The main exchangeable cations in sawdust are: calcium (1.8 cmol+·kg−1), followed by sodium (1.3 cmol+·kg−1), potassium (1.0 cmol+·kg−1), and magnesium (0.6 cmol+·kg−1) [26]. Finally, according to the method given by Ngah and Hanafiah [32], the pHZPC of the used sawdust was evaluated at 6.8.
To obtain information on the main functional groups involved in MB adsorption, Fourier Transform Infrared Spectroscopy (FTIR) analyses of sawdust before and after MB adsorption were performed. A mass of 0.1 g of sawdust was ground and encapsulated in 1 g of KBr and infrared spectra were obtained with a Magna-IR560 Nicolet spectrometer. IR absorbance data were obtained for wave numbers in the range from 400 to 4000 cm−1.
2.3 Anodic oxidation experiments
The kinetic of MB removal from synthetic solutions by AO process has been followed at different initial aqueous pH values of 3, 5, 6.2, 9, and 11 for selected times between 15 and 360 min. The anodic oxidation of MB was performed galvanostatically (j = 40 mA·cm−2). Experiments were conducted in a mini-Diacell provided by Adamant Technologies, i.e. an electrolytic cell that is composed of a BDD electrode as an anode and stainless steel as a cathode with a 3-mm gap between the electrodes. The anode is a monopolar p-silicon covered with a 2–3-μm-thick BDD layer with a 12.5-cm2 rectangular surface (50 × 25 mm). The reactivity of the BDD electrode is guaranteed by 1 M of an H2SO4 solution circulation during 30 min, prior to each electrolysis experiment [33,34]. The mini-Diacell is supplied with the dye solution from a reservoir tank (Vs = 1000 mL) using a peristaltic pump working in recycling mode. The MB initial concentration was set to 58 mg·L−1.
2.4 Adsorption onto sawdust experiments
Batch experiments consisted in determining the MB removal kinetic and at equilibrium as well as evaluating the effect of some key parameters such as solution pH and adsorbent dosage on MB removal by sawdust. These experiments were conducted at 20 ± 2 °C in 120-mL capped flasks. During these assays, a precise amount of sawdust was shaken in 100 mL of MB solution during a desired time at 400 rpm using a Varimag-poly15 magnetic stirrer. The dissolved MB concentrations and the related COD contents were determined after the centrifugation of the suspension at 3000 rpm for 15 min using a Sigma4-15 apparatus.
2.4.1 Kinetic and isotherms studies
The study of the MB adsorption kinetics and the adapted related isotherms is very useful for a better understanding of the involved mechanisms and also for the design of future large-scale adsorption facilities. In this work, five initial MB concentrations (from 10 to 100 mg·L−1) were used and their progress were followed at various times from 1 to 960 min. The adsorbent dosage and aqueous pH were fixed to 4 g·L−1 (dry amount) and 6.2 (natural: without any adjustment), respectively.
Many models are used to fit the kinetic and equilibrium adsorption experiments. The most famous ones for kinetics are the pseudo-first-order, pseudo-second-order, intraparticle and film diffusion models [35] and, for the equilibrium, the Freundlich, Langmuir, and Temkin models [36]. In this study, the used kinetic and equilibrium models and their linear forms are given in Tables 1 and 2 respectively.
Kinetic models used for MB adsorption onto sawdust.
Model | Equation | Linear form |
Pseudo-first-order | (1) | (2) |
Pseudo-second-order | (3) | (4) |
Film diffusion | (5) | |
Intraparticle diffusion | (6) |
Isotherms models used for MB adsorption onto sawdust.
Isotherm | Equation | Linear form | Plot |
Langmuir | (7) | (8) | |
Freundlich | (9) | (10) | |
Temkin | (11) | (12) |
2.4.2 Effect of initial pH solutions and sawdust dosages
The effects of the initial aqueous pH solutions and sawdust doses on synthetic MB removal were investigated in a series of experiments with varying pH values: 3, 5, natural (without adjustment), 9, and 11, and varying doses from 1 to 16 g·L−1. During these assays, the initial MB concentration and contact time were fixed to 58 mg·L−1 and 4 h, respectively. This time is considered to be sufficient to attain an equilibrium state between MB and the adsorbent material. The pH adjustment was done using small volumes (lower than 1 mL) of 0.1 M HNO3 and NaOH solutions.
2.4.3 MB removal by combined AO and adsorption onto sawdust technologies
Combined treatment of MB has been investigated for the two following cases:
- • AO of a synthetic MB solution at an initial concentration of 58 mg·L−1 and pH of 6.2 for 1 h followed by adsorption onto sawdust. The tested doses were fixed to 0.5, 1, 3, and 5 g·L−1;
- • AO of the same synthetic solution during 2 h, followed by adsorption onto sawdust. The used doses were set to 0.125, 0.5, 1, and 3 g·L−1.
The used contact time between MB and sawdust particles was fixed to 4 h, which is largely sufficient to attain an equilibrium state characterized by stable residual MB concentrations.
2.5 MB removal calculation
2.5.1 MB removal efficiencies
The MB removal efficiencies by AO and/or adsorption onto sawdust processes have been followed not only through color removal but also through global mineralization (via COD contents measurements) as cited below:
(13) |
(14) |
2.5.2 Average current efficiency
The average current efficiency (ACE) can be defined as the part of the current directly used for the oxidation of organic compounds from the beginning of electrolysis. The ACE of the MB anodic oxidation was calculated using the following relationship [14]:
(15) |
2.5.3 Energy consumption
The energy consumption (EC) per unit of removed COD mass (W·h·gCOD−1) at time t was determined as follows [13]:
(16) |
2.5.4 MB adsorbed amounts
The amounts of the adsorbed MB onto sawdust were calculated from the decrease of the MB concentration in the aqueous solutions. At a given time t, the amount of adsorbed MB onto the sawdust, Qt (mg·g−1), was obtained as follows:
(17) |
3 Results and discussion
3.1 Removal of methylene blue by anodic oxidation
3.1.1 Color and COD removal
The effect of pH on dye removal using AO on a BDD electrode is generally considered as an important operating parameter. Due to the large variety of the existing dyes with different chemical structures and properties, removal efficiencies versus pH are sometimes contradictory [4,37,38]. In the present work, the MB degradation by anodic oxidation has been conducted according to the experimental conditions cited in Section 2.3. The experimental results (Fig. 2a) showed that color removal is clearly dependent on the initial pH of the synthetic solutions, essentially during the first electrolysis stages (t < 180 min). As it can be seen in Fig. 2a, acidic to natural pH is the optimal range for MB discoloration efficiency. Indeed, for example, after 1 h of treatment, MB discoloration decreases from 61.2% to 29.1% when the initial pH was raised from 3 to 11. This finding could be due to the production of additional oxidants such as persulfates rather than OH, which enhance the efficiency of color removal [2]. On the other hand, for all the studied pH values, the MB kinetic degradation by AO is relatively important for times lower than 2 h for acidic to natural pH. At this time, the MB discoloration efficiencies were about 91.5%, 84.0%, 75.3% and 60.1% for pH of 3, 6.2, 9 and 11 respectively. For higher times, MB degradation increased, but with a much slower rate. A complete discoloration has been observed for all the tested pH values after about 4.5 h. A similar result has been reported by Akrout et al. [11] when studying the removal of cibacron yellow by AO on a BDD electrode.
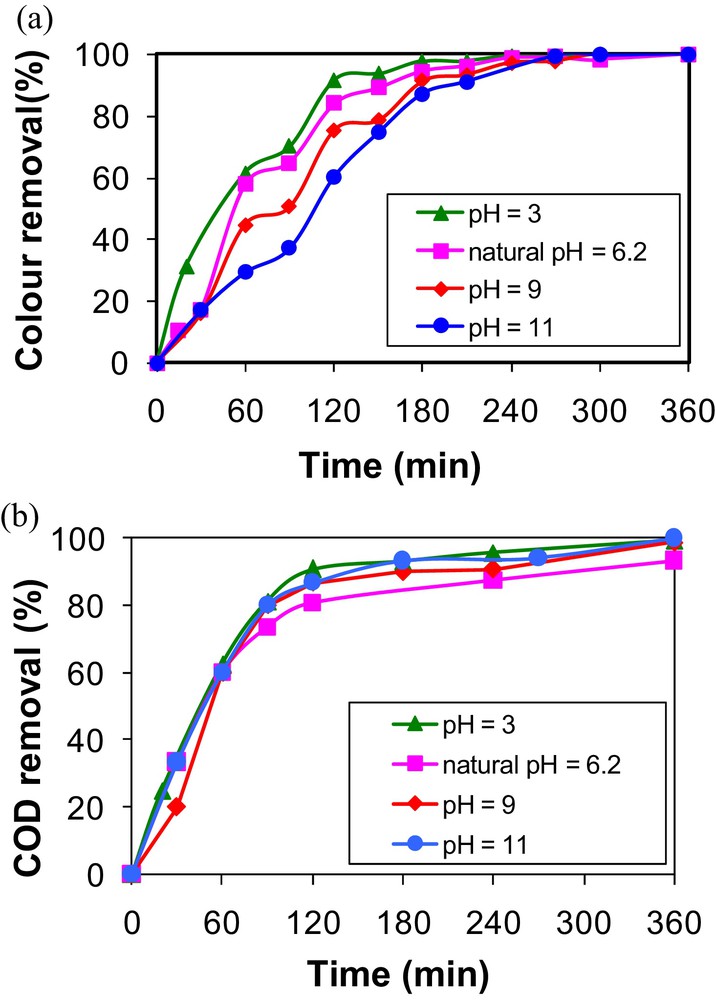
Effect of initial solutions pH on methylene blue removal by anodic oxidation (j = 40 mA·cm−2, C0 = 58 mg·L−1) (a) color removal; (b) COD removal.
MB concentration decays for all the studied pH were fitted to the pseudo-first-order kinetic model (Table 3). It appears that relatively high discoloration constant rates (Kdiscoloration) are obtained for acidic to natural pH media, which suggests that hydroxyl radicals and persulfates are very powerful oxidants for MB color removal. Lower constant rates were observed for alkaline pH, which could be explained by the fact that at high pH values (> 8.5), significant concentrations of present within the solution may scavenge the OH formed from hydroxyl anions. As a consequence, the MB discoloration rate may be reduced compared to the situation in acidic to natural pH media [39].
pH effect on kinetic data of MB discoloration using AO treatment, assuming pseudo-first-order reactions.
pH | 3 | 6.2 | 9 | 11 |
Kdiscoloration (×10−2) | 1.91 (R2 = 0.99) | 1.48 (R2 = 0.96) | 1.29 (R2 = 0.96) | 1.04 (R2 = 0.91) |
On the other hand, contrary to color, COD removal by AO on BDD demonstrates a low dependency on the initial aqueous pH values (Fig. 2b). Indeed, no significant difference on COD removal was registered for all the tested pH. Two phases were depicted regarding COD removal versus time. In the first one, a relatively fast kinetic was observed for times lower than 2 h. At this time, COD removal attained an average value of about 86.4% for all studied pH. From this time, COD removal efficiencies increased, but with a much slower rate. A complete mineralization of the MB molecules and of the related electrogenerated by-products has been observed after an electrolysis time of 6 h.
Discoloration and COD removals are relatively similar at acidic pHs (3 and 6.2). However, at alkaline pHs (9, 11), the COD removal is higher than discoloration, which is not common, as discoloration kinetics is in general higher than mineralization. One explanation could be the absorption of by-products at the maximum wavelength of MB due to the stabilization of the chromophore group at alkaline pHs. It is worth mentioning that according to a recent paper by Yang et al. [40], dealing with ultrasound electrochemical degradation of MB wastewater using a nanocoated electrode, the main intermediates might be: cyclohexyl(3-(dimethylamino)phenyl)methanone, 2-((4-aminophenyl)thio)aniline, cyclohexyl(phenyl) methanone, 3-isocyanatophenol, formic acid, acetic acid, and other small organic acids.
It is important to underline that the complete discoloration and COD removal depend not only on the physicochemical characteristics of the dyes, but also on the experimental electrolysis conditions. Indeed, Canizares et al. [41] obtained a complete color and COD removal after 4 h of AO treatment using Si/BDD for Eriochrome Black-T removal (COD0 = 100 mgO2 L−1; j = 30 mA cm−2). However, about 15 h of electrolysis were needed to ensure a complete mineralization of acid blue 22 for an initial concentration of 147.5 mg·L−1, an applied current of 40 mA cm−2, and an active surface of 25 cm−2 [14].
3.1.2 Energy consumption and average current efficiency
EC and ACE evolution versus electrolysis time are given in Fig. 3a and b respectively. From Fig. 3a, it can be clearly seen that for all the studied pH, EC slightly increases for times lower than 2 h. Then, they exponentially increase with the electrolysis time. For instance, at natural pHs, the required energy after 1 hour of AO treatment is about six times lower than the one necessary for a complete mineralization of MB. On the other hand, from Fig. 3b, the ACE values decreased versus the electrolysis time. Maximum efficiencies were obtained essentially during the initial AO treatment phases. ACE losses were registered during the experiment for all the studied pHs. This behavior is attributed to the discontinuous electrochemical anodic oxidation of the MB solution with conductive diamond anodes [37]. The ACE decays to about 30% at the end of the AO treatment, which reflects a slow destruction of the accumulated aliphatic acids, which are produced from the oxidation of MB [13].
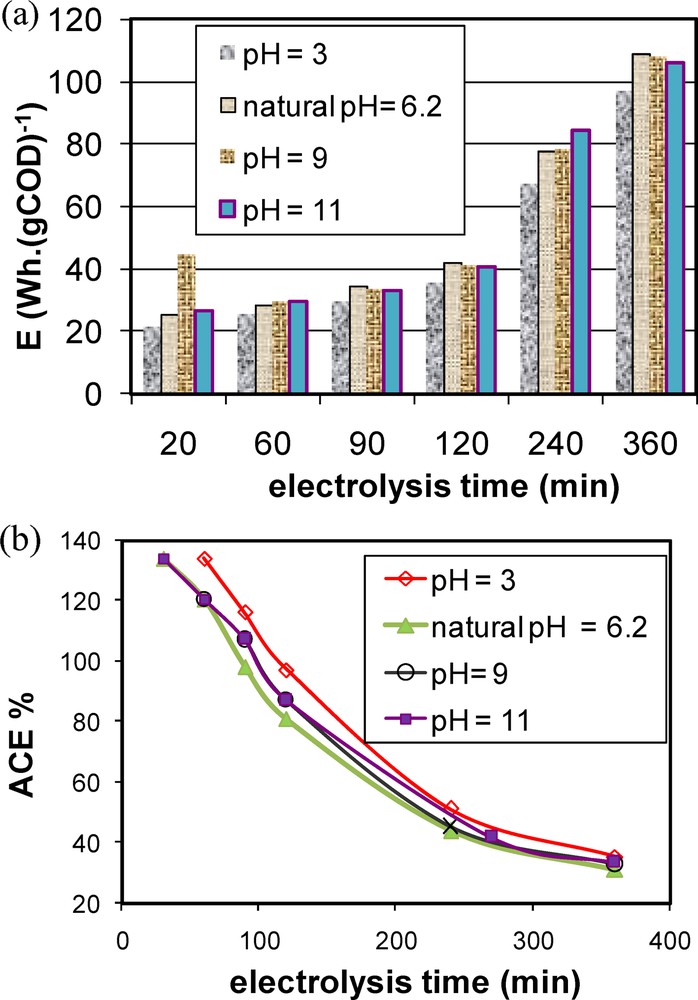
Effect of aqueous pH and operating time on (a) energy consumption, (b) average current efficiency “ACE” by AO process.
3.2 Methylene blue removal by adsorption onto sawdust
3.2.1 Methylene blue removal kinetics and isotherms
The experimental results regarding MB removal by sawdust for several initial concentrations and contact times showed that MB adsorption process is clearly time dependent (Fig. 4). The amount of adsorbed MB (Qt) increased rapidly for contact times lower than 20 min: at this time, it reaches about 61% and 38% of the totally adsorbed amount for initial aqueous MB concentrations of 30 and 100 mg·L−1. For periods greater than 20 min, the uptake is further increased, but with a much slower rate. The equilibrium state, characterized by approximate constant adsorbed MB amounts was reached at about 1, 4 and 12 h for 10, 50, and 100 mg·L−1 respectively.
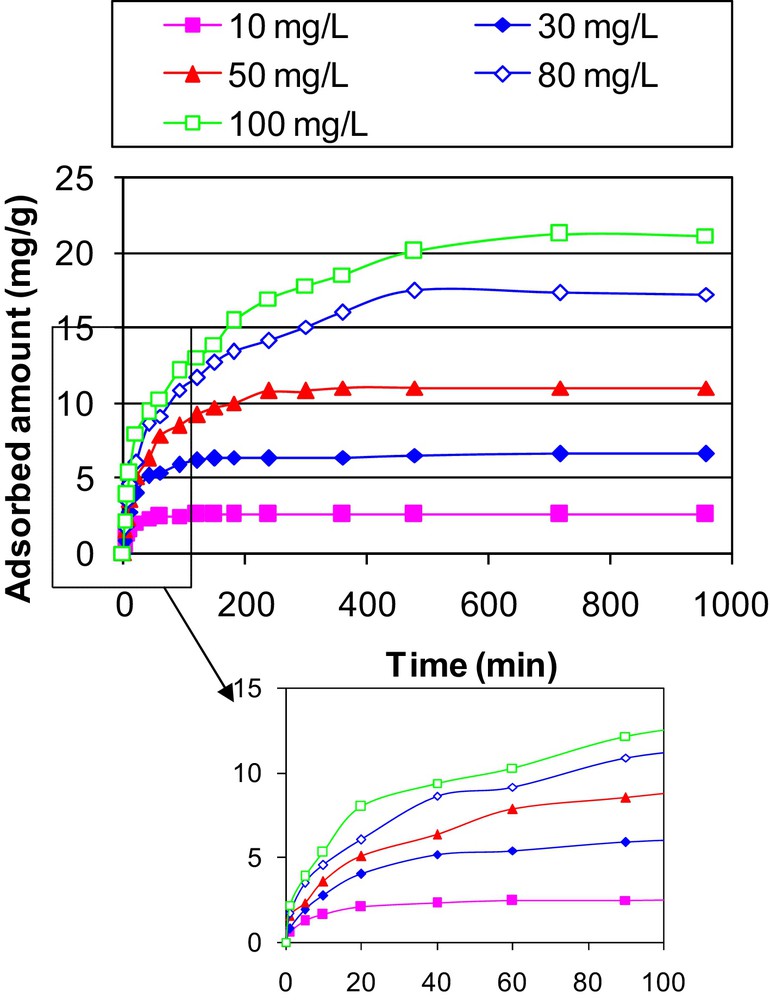
Effect of contact time and initial aqueous concentrations on the adsorption of MB onto sawdust (adsorbent dosage = 4 g·L−1, initial solution pH = 6.2, T = 20 ± 2 °C).
At the beginning of the adsorption experiments, the adsorption rate was relatively fast, because the MB cations were mainly adsorbed at the exterior surfaces of the sawdust particles. When the adsorption of the exterior surfaces of sawdust attained saturation, the MB ions entered the sawdust and were adsorbed by the interior surface of the particles. This finding is similar to previous studies, where the MB adsorption reaches an equilibrium state at about 200 min for garlic peels [42] and 360 min for rejected tea [43].
The parameters of the three used models (pseudo-first-order, pseudo-second-order and intraparticle diffusion models) are presented in Table 4. The correlation coefficients for the Lagergren irreversible first-order model obtained for all studied initial MB concentrations were relatively low (between 0.805 and 0.982), and the theoretical adsorbed masses (QeI), were significantly different from the experimental ones. Therefore, the reaction involved in the present adsorption system was not fitted by the first-order kinetic model. In contrast, the pseudo-second-order model showed the best fit to the experimental data with very high squared correlation coefficients (R2 > 0.992). In addition, as it can be seen from Table 4, the calculated adsorbed masses at equilibrium (QeII) according to the pseudo-second-order model are in good agreement with the experimental ones: the maximum difference did not exceed 4.8% for C0 = 100 mg·L−1. Thus, these results suggest that the pseudo-second-order model, based on the assumption that the rate-limiting step might be chemical adsorption involving valency forces through sharing or exchange of electrons between adsorbent and adsorbate, is the most appropriate one under the studied experimental conditions. Similar findings have been registered for MB adsorption on cedar sawdust and crushed brick [25], agricultural biomass [42], and wheat shells [44].
Kinetic rate constants related to the adsorption of MB onto sawdust at different initial aqueous concentrations.
Initial MB aqueous concentration (mg·L−1) | |||||
10 | 30 | 50 | 80 | 100 | |
Experimental Qe (mg·g−1) | 2.556 | 6.600 | 11.025 | 17.301 | 21.204 |
Irreversible first-order model | |||||
k1 (min−1) | 0.030 | 0.009 | 0.015 | 0.006 | 0.006 |
Calculated QeI (mg g−1) | 1.378 | 2.761 | 10.010 | 13.400 | 16.726 |
R2 | 0.943 | 0.805 | 0.937 | 0.972 | 0.982 |
Pseudo-second-order model | |||||
k2 (g·mg−1·min−1) | 0.110 | 0.013 | 0.004 | 0.001 | 0.0007 |
Calculated QeII (mg·g−1) | 2.570 | 6.680 | 11.364 | 18.116 | 22.222 |
R2 | 1.000 | 0.999 | 0.999 | 0.995 | 0.992 |
Film and intraparticle diffusion models | |||||
Df (10−10 m2·s−1) | 3.320 | 1.506 | 0.845 | 0.585 | 0.526 |
R2 | 0.984 | 0.994 | 0 .981 | 0.993 | 0.972 |
Dip (10−10 m2·s−1) | 3.494 | 1.520 | 1.003 | 0.602 | 0.559 |
R2 | 0.999 | 0.987 | 0.997 | 0.982 | 0.988 |
The analysis of the adsorption of MB onto sawdust particles with film and intraparticle diffusion models showed that the depicted two-phase plot suggests that the adsorption process proceeds by surface adsorption at the earlier stages and by intraparticle diffusion at later stages (see Fig. 4). The values of diffusion coefficients given in Table 4 indicated that for all the initial aqueous concentrations, the intraparticle diffusion coefficients are slightly greater than those of the film diffusion through the boundary layer. This finding confirms that in the case of sawdust, intraparticle and especially film diffusion process control the rate of adsorption of MB ions. This result is consistent with studies related to MB removal by Mansonia sawdust [45] and hazelnut shell [46].
On the other hand, MB removal increased with increasing the initial MB concentrations. Indeed, increasing the initial MB concentration from 10 to 100 mg·L−1 permits the sawdust particles to improve their adsorption capacities from 2.6 to 21.2 mg·g−1. This behavior is due to the fact that the higher ones are the initial aqueous MB concentrations; the more important is the concentration gradient between the aqueous solution and the sawdust particles, which results in more significant diffusion rates. Furthermore, for higher initial aqueous concentrations, the contact probability between MB species contained in the aqueous phase and the adsorbent should be more privileged [47].
The Langmuir, Freundlich and Temkin adsorption constants estimated from the isotherms at 20 °C and the corresponding correlation coefficients are presented in Table 5. The highest regression correlation coefficient (0.992) was observed for the Freundlich model, followed by the Langmuir (0.982) and Temkin (0.953) models. This indicates that the three models are suitable for describing the adsorption equilibrium of MB onto sawdust. The maximum adsorption capacity (Qm) determined from the Langmuir isotherm was estimated to be about 33.6 mg·g−1 at pH 6.2. This calculated value proves that the used sawdust efficiently removes MB from aqueous solutions compared to several other natural materials such as raw beach sawdust [24], orange peel [48] and wheat shells [44]. On the other hand, the calculated value of Freundlich exponent n = 1.477 is in the range of 1–2, which indicates a moderate adsorption of MB onto sawdust. Moreover, the Langmuir parameters, RL, for an initial aqueous concentration of 10 and 100 mg·L−1 were estimated to 0.548 and 0.108 respectively. These values are lower than 1, which confirms that the used adsorbent favors MB adsorption.
Adsorption isotherms constants for MB adsorption onto sawdust.
Isotherm | Freundlich | Langmuir | Temkin | ||||||
n | KF | R2 | Qm (mg·g−1) | kl (L·mg−1) | R2 | A (L·g−1) | B | R2 | |
Sawdust | 1.477 | 2.851 | 0.992 | 33.573 | 0.082 | 0.982 | 1.338 | 5.95 3 | 0.953 |
3.2.2 Effect of aqueous pH and sawdust doses on methylene blue removal
Color removal from synthetic solutions containing MB at an initial concentration of 58 mg·L−1 by adsorption onto sawdust is very dependent on both the aqueous pH values and the adsorbent dosage (Fig. 5a). Indeed, MB removal efficiencies increase when increasing the pH and the adsorbent doses. The worst removal efficiency (4.2%) was observed for the lowest tested initial pH (pH = 3) and adsorbent dose (1 g·L−1). This result is attributed to a conjugate effect of an excess of H+ ions in aqueous solutions, which could compete with the positively charged ions of MB, and also to the relatively low availability of adsorption sites [49]. Additionally, MB ions could also compete with Na+ (from Na2SO4 electrolyte). However, for initial pH values and adsorbent doses higher than 6.2 and 8 g·L−1, color removal efficiencies exceeded 97% (Fig. 5a). This behavior is due to the presence of abundant active sites that could react with MB ions and also to the fact that the pH values were higher than the pHZPC, which facilitates the adsorption of MB cations onto the negative charged surface area of the sawdust particles. It is worth mentioning that, for sawdust doses higher than 8 g·L−1, adsorption removal efficiencies of MB are important (higher than 95%), even for acidic pHs. This result confirms the importance of the presence of a large number of active sites in the MB removal from aqueous solutions [3]. This result is consistent with other MB adsorption studies using natural organic adsorbents such as hazelnut shell [46] and Meranti sawdust [30]. Thus, neutral to alkaline pH are recommended when using sawdust for the removal of MB from aqueous solutions. However, a sawdust dose of 8 g·L−1 is optimum, since an important discoloration efficiency was observed, even in acidic media.
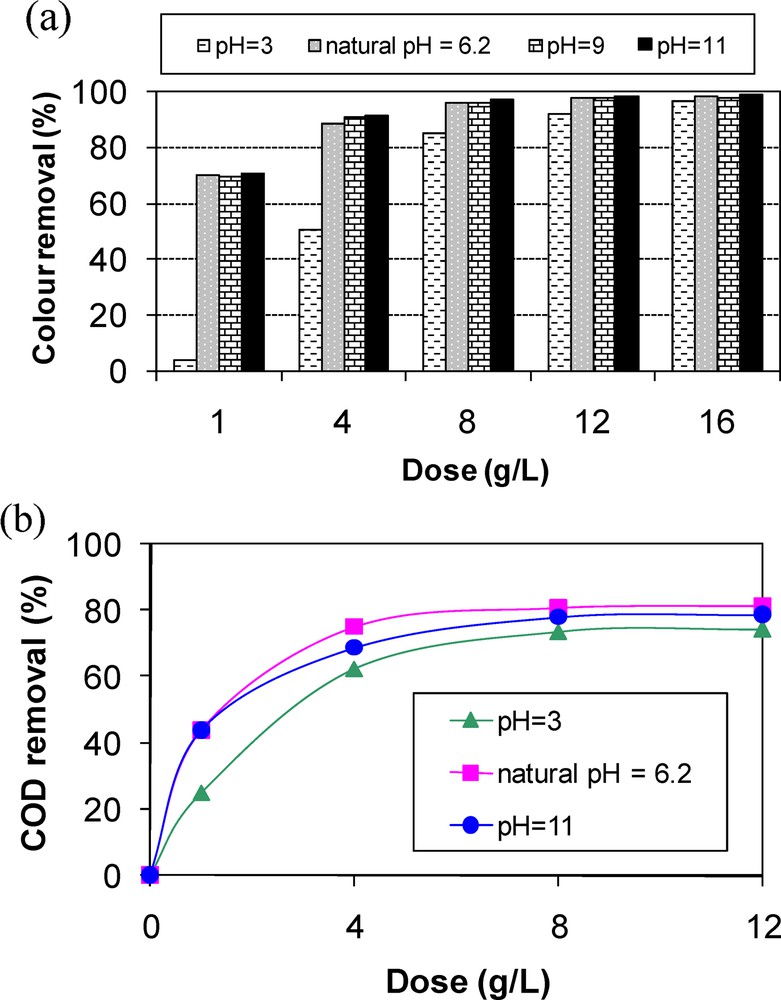
Effect of initial solutions pH and sawdust doses on methylene blue removal (C0 = 58 mg·L−1) (a) color removal; (b) COD removal.
Concerning COD removal, the experimental results (Fig. 5b) showed that, for the same reasons as those cited above for discoloration, its removal efficiency was relatively low for acidic pH values and small sawdust doses. For doses higher than 8 g·L−1, COD removal efficiency attains about 80% to 83% for pH values higher than 6.2. This is probably because of the resistance to mass transfer of MB molecules from bulk liquid to the surface of the sawdust particles, which becomes important at high adsorbent doses [50]. On the other hand, MB removal by chemisorption could generate the destruction of chemical bonds responsible for the blue color (chromophore group), and consequently the formation of probably non-colored by-products. These new molecules could be linked to the percentage of non-removed COD. This result proves that even if the synthetic solutions were quasi-completely discolored, some organic molecules are not adsorbed by the used sawdust.
The FTIR spectra of raw sawdust showed the presence of a large peak at 3422 cm−1 representing the –OH stretching of the phenol group of cellulose and lignin and important peaks at 2926 cm−1, 1722 and 1636 cm−1 that are attributed to –CH2 stretching of aliphatic compounds, CO stretching of aldehyde groups and CC stretching of phenol compounds respectively [26,30]. Other existing functional groups are presented in Table 6. To determine the main functional groups involved in MB adsorption onto the used sawdust, a comparison between FTIR spectra before and after MB was carried out. The related results showed shifts in some functional groups bands due to MB adsorption (Fig. 6 and Table 6). The main shifts corresponded to bonding hydroxyl OH, CO stretching, SO3 stretching, OH alcohols (primary and secondary) and aliphatic ethers at 3422, 1720, 1266 and 1162 cm−1, respectively. These registered changes suggested that these functional groups could be involved in MB adsorption onto the used sawdust.
Comparison between FTIR spectra before and after MB adsorption onto the used sawdust.
Peak number | Wave number | Differences | Assignment | |
Before adsorption | After adsorption | |||
1 | 3422 | 3436 | −14 | Bonded hydroxyl OH |
2 | 2926 | 2926 | – | CH2 stretching |
3 | 1720 | 1722 | −2 | CO stretching |
4 | 1636 | 1636 | – | CC stretching |
5 | 1444 | 1444 | – | CH2 deformation |
6 | 1384 | 1384 | – | COH bending |
7 | 1266 | 1264 | 2 | SO3 stretching |
8 | 1162 | 1160 | 2 | OH alcohols (primary and secondary) and aliphatic ethers |
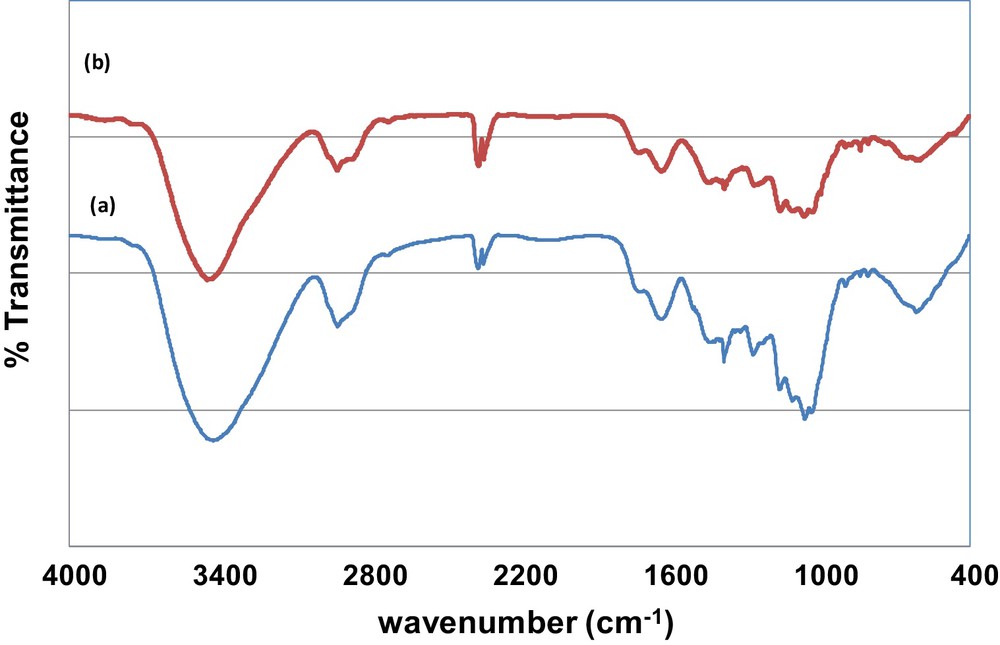
FTIR spectra of sawdust: (a) before MB adsorption, (b) after MB adsorption.
3.3 Methylene blue removal by combination of anodic oxidation and adsorption
As previously shown in Section 3.1, MB removal efficiencies by AO alone were relatively rapid at the beginning of the experiments for acidic to neutral aqueous solutions. However, these efficiencies increase, but with much slower rates, to attain a complete mineralization after 6 h. For example, for a natural pH (without adjustment), color and COD removal efficiencies exceed about 59% and 83% after 1 and 2 h of electrolysis, respectively. Besides, the energy consumption highly increased after 2 h of AO treatment. This means that this technology is economically suitable for wastewaters pretreatment. Thus, combining AO pretreatment with other technologies such as adsorption onto low-cost material should considerably decrease the cost of the overall treatment procedure. In this work, we studied the combination of MB adsorption onto different dosages of sawdust after 1 and 2 h of pretreatment with AO, respectively, for an initial pH of 6.2. The used experimental conditions are illustrated in Section 2.4.3.
The combination of MB anodic oxidation for 1 h and adsorption onto sawdust showed that this procedure could be considered as a very interesting option for the treatment of industrial wastewaters in terms of color removal (Fig. 7a). Indeed, after 1 h of AO pretreatment, about 97.7% of the color of the initial MB concentration (58 mg·L−1) was removed when using only 5g·L−1 of sawdust. This discoloration efficiency has been obtained with 12 g·L−1 when using adsorption alone. It is important to mention that after 1 h of AO pretreatment, a limited sawdust dosage of 0.5 g·L−1 permitted to remove 75.1% of color, which is a result to be compared with the one obtained using adsorption alone.
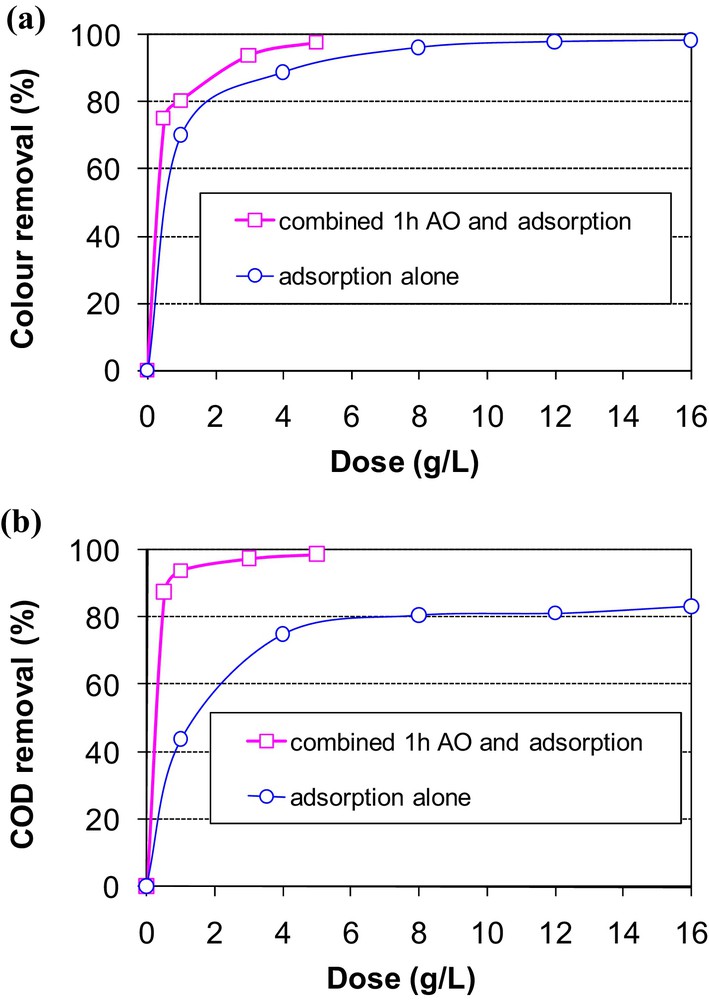
Effect of the sawdust dose on methylene blue removal from an aqueous solution pretreated with AO for 1 h (C0 = 58 mg·L−1) (a) color removal, (b) COD removal.
The effect of the combination of AO and adsorption was spectacular in terms of COD removal (Fig. 7b). In fact, after 1 h of AO pretreatment, only a sawdust dose of 0.5 g·L−1 has ensured a COD removal efficiency higher than 87%. However, when adsorption alone was used, the COD removal efficiency was evaluated at about 81%, even for sawdust doses higher than 8 g·L−1. Moreover, COD removal efficiency from the synthetic solutions pretreated with AO for 1 h increases when increasing the sawdust dose. A quasi-plateau has been observed for sawdust doses higher than 3 g·L−1. This quasi-equilibrium state corresponds to a COD removal efficiency of about 99%.
This result indicates that this sawdust's characteristic can be considered as an important added value to its intrinsic relatively high adsorption capacity of the MB molecule. In fact, relatively very low solid support doses could efficiently ensure both color and COD removal, suggesting that sawdust might present an important removal efficiency of not only the basic molecules of MB but also of the electrogenerated by-products formed by the AO pretreatment for 1 h. On the other hand, this gain in terms of adsorbent amount required for color and COD removal is accompanied by a gain on energy consumption. Indeed, a value of about 80 W·h·gCOD−1 was obtained when the AO time was reduced from 6 to 1 h (see Fig. 3a). Furthermore, the required sawdust amount was about 24 times lower than the one necessary when using adsorption alone.
Similarly, the combination of 2 h of AO followed by adsorption onto sawdust has significantly improved color and COD removal efficiencies (Fig. 8a and b). Only a dose of 0.125 g·L−1 allowed 97.0% and 95.0% of color and COD removal. These efficiencies were higher than the ones obtained with 16 g·L−1 of sawdust dosage when using adsorption alone. This MB removal enhancement is accompanied by an important energy consumption gain estimated to be about 67 W·h·gCOD−1.
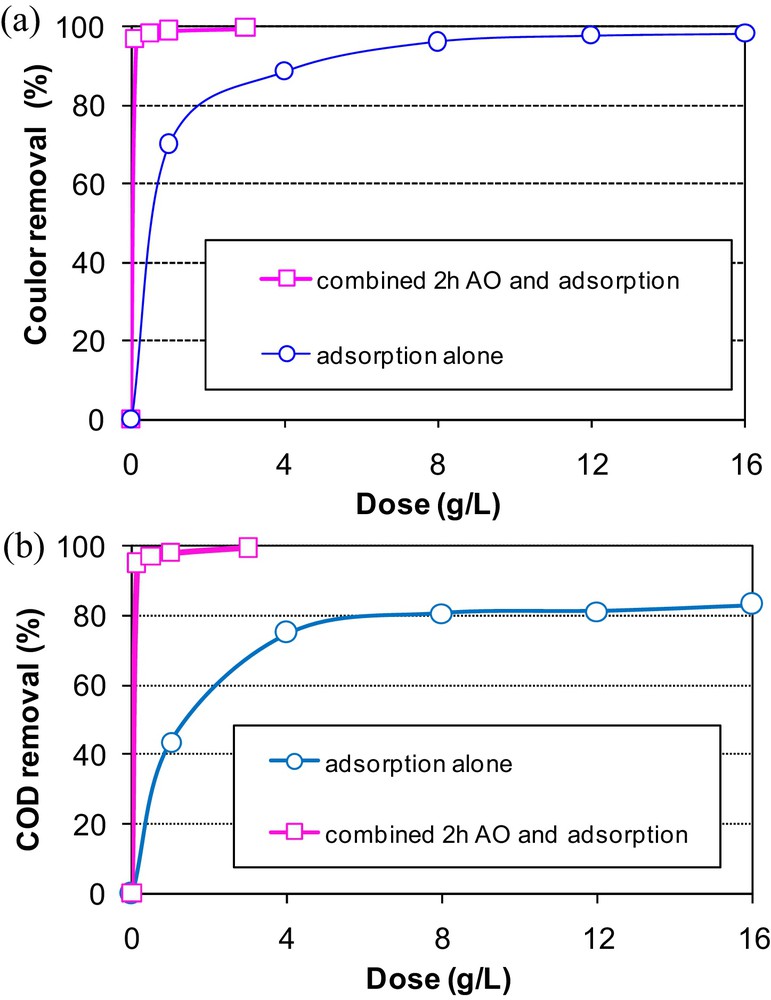
Effect of the sawdust dose on methylene blue removal from an aqueous solution pretreated with AO for 2 h (C0 = 58 mg·L−1) (a) color removal, (b) COD removal.
This enhancement of MB removal could be explained by the fact that:
- • AO converts MB molecules to low-molecular-weight intermediates that would be more easily adsorbed onto the sawdust small-size particles pores;
- • AO electrolysis could lead to the generation of negatively charged intermediates, which could be sorbed onto the positively charged sawdust sites.
4 Conclusions
The effects of anodic oxidation on BDD electrode, adsorption onto sawdust and combined anodic oxidation/adsorption on MB removal from synthetic solutions were investigated. Anodic oxidation, adsorption onto sawdust are both effective for removing methylene blue from aqueous solutions. For AO technology, a complete mineralization has been observed for a large pH interval after 6 h of operating time. The adsorption onto sawdust is dependent on both the pH and the dose of adsorbent. The maximum color and COD removal efficiencies were evaluated to 98% and 81%, respectively, and corresponded to a sawdust dose of 12 g·L−1. Combining AO for 1 or 2 h with adsorption onto sawdust leads to a significant improvement in color and essentially COD removals, which reflects the sorption of not only the MB molecules, but also of the electrogenerated species onto sawdust. Thus, AO followed by adsorption onto sawdust is an attractive, cost-effective, and promising process for treating methylene blue polluted wastewaters in order to produce effluents that can be reused for many purposes. Further investigations will be conducted regarding the identification of the formed by-products (LC-MS) and the application of the combined process for treating real industrial wastewaters.