1 Introduction
Nowadays, for considering green chemistry aspects, chemists are concerned with minimizing the environmental pollution caused by traditional solvents and try to establish methodologies based on solvent-free conditions. The drive for the development of dry media reactions are ease of purification, high reaction rates, environmentally friendly conditions, less energy requirement and considering economic and environmental demands [1].
The employment of a reusable solid supported heterogeneous catalyst for the efficient synthesis of heterocyclic compounds remains a challenge to chemists. Reactions with reagents that are immobilized on inorganic solid supports show several advantages over the conventional reactions in solution. Recently, the use of heterogeneous catalysts under solvent-free conditions has emerged as an eco-friendly alternative of great importance within organic synthesis [2–4].
As we have recently reported [5], nano titania-supported sulfonic acid (n-TSA) is a heterogeneous nano catalyst, which can be efficiently used for the promotion of the reactions that need the use of an acidic catalyst, because it acts as a Lewis and a Brønsted acid simultaneously. Simple work-up procedures, improved product yields, greater ease of purification, shorter reaction times, milder reaction conditions and recyclability of the catalyst are the main superiorities of n-TSA.
Xanthene—dibenzopyran—is a polyaromatic cyclic ether in which two benzene rings are fused to tetrahydropyran. Xanthene derivatives are very important heterocyclic compounds due to their broad spectrum of pharmacological and biological properties such as antibacterial, antidepressant, antimalarial, anti-inflammatory and antiviral activities [6]. Furthermore, they can be used as dyes, pH-sensitive fluorescent materials, in laser technologies and in photodynamic therapy [7–10]. Owing to their wide range of applications, their synthesis has gained a lot of attention in recent years.
The most common methodology for the synthesis of xanthene derivatives is the condensation of cyclohexan-1,3-diones or β-naphthol with aliphatic and aromatic aldehydes in the presence of different catalysts and solvents [11–14], but there are only two reports on cyclocondensation reaction between α,α′-bis(substituted-benzylidene)cycloalkanones and 1,3-diketones in the literature that has been published in 2013 [15,16]. However, these few studies suffer from significant limitations like using toxic solvents, tedious work-up, long reaction times, poor yields, harsh conditions and difficult separation methods. Hence, a simple, rapid, efficient and green procedure is still strongly desired for this type of reaction.
In this paper, we describe a facile and efficient method for the synthesis of (E)-5-benzylidene-3,3-dimethyl-9-phenyl-2,3,4,5,6,7,8,9-octahydro-1H-xanthen-1-one derivatives by using α,β-unsaturated cycloalkanones as starting materials (Scheme 1). To the best of our knowledge there are no literature reports on solvent-free conditions for this type of reaction.

Synthesis of xanthene derivatives.
2 Results and discussion
As part of our continuing efforts for the development of efficient green methodologies [17–19] and n-TSA-catalyzed organic transformations [5], herein, we report a new and simple synthesis of (E)-5-benzylidene-3,3-dimethyl-9-phenyl-2,3,4,5,6,7,8,9-octahydro-1H-xanthen-1-one derivatives (5). As we have mentioned earlier, there are only two reports about their synthesis meaning that probably the reaction must be difficult. On the other hand, we have reported n-TSA as a very efficient new nano catalyst in our last work for a vast variety of organic syntheses. So we have decided to investigate it for this type of condensation.
As α,α′-Bis(substituted-benzylidene)cycloalkanones (3) are not commercially sourced, so, first of all we have reported a simple and rapid method for their synthesis. α,α′-Bis(substituted-benzylidene)cycloalkanones (3) are prepared by cross-Aldol condensation of aromatic aldehydes (2) with cyclic ketones (1). We have commenced our experiments by examining the model reaction of cyclohexanone and benzaldehyde in the presence of n-TSA in various amounts of catalyst, temperatures and solvents. The results are summarized in Table 1.
Optimum conditions for the synthesis of α,α’-bis(Substituted-benzylidene)cycloalkanonesa.
Entry | Catalyst (mmol) | Solvent | Temperature (°C) | Yieldb (%) |
1 | 0 | – | 90 | 4 |
2 | n-TSA (0.03) | – | 90 | 42 |
3 | n-TSA (0.05) | – | 90 | 54 |
4 | n-TSA (0.10) | – | 90 | 78 |
5 | n-TSA (0.20) | – | 90 | 93 |
6 | n-TSA (0.30) | – | 90 | 92 |
7 | n-TSA (0.20) | – | Room temperature | Trace |
8 | n-TSA (0.20) | – | 50 | 63 |
9 | n-TSA (0.20) | – | 70 | 82 |
10 | n-TSA (0.20) | – | 110 | 90 |
11 | n-TSA (0.20) | EtOH | 90 | 41 |
12 | n-TSA (0.20) | H2O | 90 | 17 |
13 | n-TSA (0.20) | PEG | 90 | 36 |
14 | n-TSA (0.20) | CH3CN | 90 | 12 |
15 | Nano-TiO2 (0.20) | – | 90 | 0 |
16 | Bulk-TiO2 (0.20) | – | 90 | 0 |
17 | Bulk-TiO2-SO3H (0.20) | – | 90 | 75 |
a Reaction conditions: cyclohexanone (1 mmol), benzaldehyde (2 mmol), reaction time: 20 min.
b Isolated yields.
As Table 1 shows, the trial reaction gives the best yield under solvent-free conditions and proceeds smoothly at 90 °C (oil bath) with a catalytic amount of n-TSA to afford the desired product, α,α′-bisbenzylidenecyclohexanone (3), in 20 min (Table 1, entry 5). With other selected temperatures and solvents (Table 1, entries 7–14), the reaction gave rather lower yields within comparable reaction times. It is notable that no product formed within a reasonable time in the absence of catalyst (Table 1, entry 1) and other titanium dioxide base such as unmodified nano TiO2 and unmodified bulk TiO2 (Table 1, entries 15, 16). On the other hand, bulk-modified TiO2 provides lower yield in comparison to n-TSA as we expected (Table 1, entry 17) [5].
Using the optimized reaction conditions (Table 1, entry 5), we have explored the generality of this method with different aldehydes to prepare a series of benzylidenes (Table 2).
Synthesis of α,α’-bis(Substituted-benzylidene)cycloalkanone derivativesa.
Entry | 3 | Time (min) | Yieldb (%) | Melting point (°C) | |
Found | Reported [17,20,21] | ||||
1 | 25 | 90 | 187–190 | 188–189 | |
2 | 30 | 93 | 240–242 | 242–243 | |
3 | 15 | 95 | 153–156 | 154–156 | |
4 | 18 | 98 | 225–228 | 228–229 | |
5 | 20 | 96 | 205–208 | 204–205 | |
6 | 15 | 90 | 254–257 | 252–257 | |
7 | 20 | 93 | 118–121 | 116–117 | |
8 | 25 | 91 | 160–163 | 161–163 | |
9 | 30 | 90 | 165–168 | 164–165 | |
10 | 20 | 95 | 100–103 | 102–104 | |
11 | 18 | 98 | 148–150 | 147–148 | |
12 | 17 | 95 | 154–157 | 156–158 | |
13 | 15 | 90 | 161–164 | 163–164 | |
14 | 20 | 92 | 197–200 | 199–202 | |
15 | 25 | 92 | 137–140 | 137–138 | |
16 | 30 | 94 | 156–159 | 156–157 | |
17 | 20 | 98 | 175–178 | 177–178 | |
18 | 20 | 97c | 174–177 | Not reported | |
19 | 15 | 90c | 185–189 | Not reported |
a Reaction conditions: cycloalkanone (1 mmol), aldehyde (2 mmol), n-TSA (0.20 mmol), solvent-free, temp. 90 °C.
b Isolated yields.
c New compounds.
In all these cases, the corresponding products were obtained in excellent yields. The results (Table 2) indicated that substrates (2) bearing both electron-donating groups and electron-withdrawing groups can be involved in this reaction to afford the desired products (3) with high yields.
After this preliminary success, our study was followed by the synthesis of the xanthenes (5) and the reaction of α,α′-bisbenzylidenecyclohexanone (3) and dimedone (4) in the presence of n-TSA was chosen as a model reaction to determine the optimum conditions.
Initially, we performed the scope of the catalyst in order to find the required catalyst load. The results are listed in Table 3.
Optimum amount of catalyst on the synthesis of xanthenesa.
Entry | Catalyst (mmol) | Time (h) | Yieldb (%) |
1 | 0 | 3 | 20 |
2 | 0.045 | 3:30 | 60 |
3 | 0.060 | 3:45 | 70 |
4 | 0.075 | 2 | 80 |
5 | 0.090 | 2:45 | 75 |
a Reaction conditions: α,α′-bisbenzylidenecyclohexanone (1 mmol), dimedone (1 mmol), temperature 110 °C, solvent-free.
b Isolated yields.
As indicated in Table 3, the best yield was obtained in presence of 0.075 mmol of catalyst (Table 3, entry 4). In order to evaluate the effect of the catalyst particle size and acidity on the catalytic activity, the results were compared with those obtained using unmodified nano TiO2, unmodified bulk TiO2 and bulk-modified TiO2. The results are shown in Table 4.
TiO2-based catalyzed synthesis of xanthenesa.
Entry | Catalyst (0.075 mmol) | Time (h) | Yieldb (%) |
1 | Nano-TiO2 | 2 | 0 |
2 | Bulk-TiO2 | 2 | 0 |
3 | Bulk-TiO2-SO3H | 2 | 70 |
4 | Nano-TiO2- SO3H | 2 | 80 |
a Reaction conditions: α,α′-bisbenzylidenecyclohexanone (1 mmol), dimedone (1 mmol), temperature 110 °C, solvent-free.
b Isolated yields.
No activity was observed in the presence of nano TiO2 and unmodified bulk TiO2 (Table 4, entries 1, 2). Bulk-modified TiO2 was found to be effective but the yield of desired product was not satisfactory in comparison to modified nano TiO2 (Table 4, entries 3, 4).
In the next step the effect of temperatures were examined over the reaction.
As indicated in Table 5, temperatures under 90 °C did not provide remarkable yields (Table 5, entries 1–3). It was observed that fast reaction occurred when raising the temperature from 90 °C to 110 °C (Table 5, entries 3,4) and the yield of product increased significantly. The best yield was obtained at 130 °C, which was considered as the optimum temperature (Table 5, entry 5).
Optimization of temperature in the synthesis of xanthenesa.
Entry | Temperature (°C) | Time (h) | Yieldb (%) |
1 | Room temperature | 2 | Trace |
2 | 70 | 2 | 20 |
3 | 90 | 2 | 35 |
4 | 110 | 2 | 80 |
5 | 130 | 2 | 90 |
a Reaction conditions: α,α′-bisbenzylidenecyclohexanone (1 mmol), dimedone (1 mmol), n-TSA (0.075 mmol), solvent-free.
b Isolated yields.
We also investigated different solvents for the model reaction (Table 6).
Optimization of solvent in the synthesis of xanthenesa.
Entry | Solvent | Time (h) | Yieldb (%) |
1 | CH2Cl2 | 4 | 30 |
2 | CH3CN | 4 | 50 |
4 | H2O | 4 | 0 |
5 | PhCH3 | 4 | 70 |
6 | PEG-400 | 4 | 20 |
7 | – | 2 | 90 |
a Reaction conditions: α,α′-bisbenzylidenecyclohexanone (1 mmol), dimedone (1 mmol), n-TSA (0.075 mmol), temperature 130 °C.
b Isolated yields.
The results of Table 6 indicate that neither polar nor non-polar solvents are suitable for this reaction (Table 6, entries 1–6) and the best yield was obtained under solvent-free conditions (Table 6, entry 7).
We then investigated the reaction scope of this catalytic system and its tolerance of functional groups in the case of other α,α′-bis(substituted-benzylidene)cycloalkanones under the optimized reaction conditions. The results are shown in Table 7.
Synthesis of xanthene derivatives under optimized reaction conditionsa.
Entry | 5 | Time (h:min) | Yieldb (%) | Melting point (°C) | |
Found | Reported [15,16] | ||||
1 | 1:50 | 92 | 206–208 | 204–206 | |
2 | 2:10 | 60 | 228–231 | 230–232 | |
3 | 2 | 93 | 239–242 | 235–237 | |
4 | 2:10 | 90 | 233–236 | 233–234 | |
5 | 2:35 | 80 | 196–199 | 198–199 | |
6 | 2:25 | 94 | 249–252 | 250–252 | |
7 | 2 | 90 | 165–168 | 166–168 | |
8 | 1:45 | 82 | 157–160 | 158–160 | |
9 | 1:50 | 87 | 166–169 | 165–167 | |
10 | 1:35 | 90 | 157–160 | 156–159 | |
11 | 1:55 | 85 | 150–153 | 149–152 | |
12 | 1:55 | 95 | 165–168 | 165–167 | |
13 | 2:30 | 90c | 208–210 | Not reported | |
14 | 1:55 | 85c | 173–176 | Not reported | |
15 | 2:05 | 92c | 207–212 | Not reported | |
16 | 1:40 | 98c | 249–253 | Not reported | |
17 | 1:45 | 91c | 228–233 | Not reported | |
18 | 1:50 | 94c | 250–254 | Not reported |
a Reaction conditions: α,α′-bis(substituted-benzylidene)cycloalkanones (1 mmol), dimedone (1 mmol), n-TSA (0.075 mmol), temperature 130 °C, solvent-free.
b Isolated yields.
c New compounds.
As indicated in Table 7, the reaction works easily for a vast range of benzylidenes with electron-donating and electron-with drawing groups. This result justified once more the efficiency of n-TSA.
The reusability of the catalyst was checked by recovering n-TSA and reusing it for seven consecutive reactions; a yield of 88–90% was obtained. This indicates that n-TSA does not lose its activity and can be recyclable (Fig. 1).
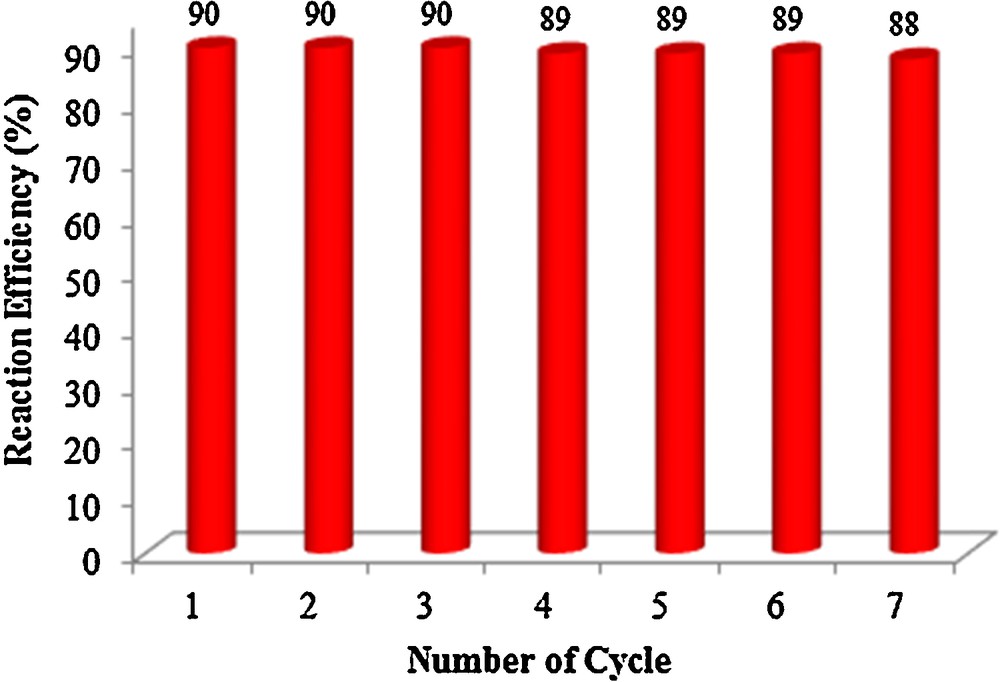
Reusability of n-TSA for the synthesis of octahydroxanthenes, Reaction conditions: α,α′-bisbenzylidenecyclohexanones (1 mmol), dimedone (1 mmol), n-TSA (0.075 mmol), reaction time: 2 h at 130 °C in solvent-free conditions.
The characterization by scanning electron microscopy of the nano-TiO2-SO3H before use and after it has been reused seven times showed the same particle size (Fig. 2).

SEM images of n-TSA before use (a) and after having reused it seven times (b).
3 Conclusion
In summary, an efficient and rapid route for the synthesis of α,α′-bis(substituted-benzylidene)cycloalkanones and their xanthene derivatives is reported under green conditions. Operational simplicity, neat conditions, short reaction time, simple work-up without column chromatographic purification, reusability of the catalyst, tolerance of various functional groups and high yields of products are the advantages of this protocol.
4 Experimental
Chemicals were purchased from the Merck chemical companies. Thin-Layer Chromatography (TLC) on commercial plates of silica gel 60 F254 was used to monitor the progress of reactions. The products were characterized by FT-IR spectra, 1H NMR, 13C NMR and X-ray. FT-IR spectra were recorded on Shimadzo FT-IR 8400 instrument. 1H and 13CNMR spectra were recorded on Bruker Avance 300 MHz and 75 MHz spectrometers, respectively, using CDCl3-d as a solvent. The chemical shifts are expressed in parts per million (ppm) and tetramethylsilane (TMS) was used as an internal reference. Melting points were recorded on a THERMO SCIENTIFIC 9100 apparatus.
4.1 General procedure for the synthesis of catalyst (n-TSA)
Chlorosulfonic acid (1 mL, 15 mmol) was added dropwise to a suspension of powdered nano TiO2 (4 g) in dry CH2Cl2 (20 mL) over a period of 30 min while the mixture was stirred slowly in an ice bath. The mixture was stirred for 30 min at room temperature until HCl evolution was seized. Then, the CH2Cl2 was removed under reduced pressure and the solid powder was washed with ethanol (10 mL) and dried at 70 °C. n-TSA was obtained as a white solid powder [5].
The number of millimoles of H+ per gram of catalyst was also evaluated. For this purpose, the surface acidic protons of nano-TiO2-SO3H (100 mg) were ion-exchanged with a saturated solution of NaCl (10 mL) by sonication. This process was repeated twice more, yielding 30 mL of proton-exchanged brine solution. Therefore, to determine the loading of acid sites on the synthesized catalyst the obtained solution was titrated by an NaOH (0.1 M) solution in the presence of phenol red indicator solution or a pH meter. The amounts of acidic protons found to be 4.5 mmol/g of n-TSA. This finding means the effective density of the acid sites.
The catalyst was already characterized by FT-IR spectroscopy, scanning electron microscopy (SEM), X-ray diffraction (XRD), thermal gravimetric analysis (TGA) and the acid strength of n-TSA was determined by the Hammett indicator method too [5].
4.2 General procedure for the synthesis of α,α′-bis(substituted-benzylidene)cycloalkanones (3)
A mixture of cycloalkanone (1 mmol), aromatic aldehyde (2 mmol) and 0.20 mmol (0.032 g) n-TSA was stirred at 90 °C under solvent-free conditions for an appropriate time. After completion of the reaction (monitored by TLC), the reaction mixture was cooled, eluted with hot ethanol (5 mL) and was centrifuged to filter the catalyst. The title compounds were obtained in their yellowish crystalline forms by recrystallization of ethanol solution.
4.3 Spectral data of new compounds
4.3.1 2,6-bis(4-flurobenzylidene)-4-(tert-butyl)cyclohexanone
Yellow crystal; m.p. = 174–177 °C; IR (KBr) (cm−1) νmax: 2956, 2869, 1664, 1602, 1508, 1155; 1H NMR (300 MHz, CDC13) 0.95 (s, 9H), 1.47 (tt, J = 12.7 Hz/2.8 Hz, 1H), 2.41 (m, 2H), 3.11 (dd, J = 15.6 Hz/2.3 Hz, 2H), 7.73 (s, 2H), 7.08–7.47 (m, 8H, ArH); 13C NMR (75 MHz, CDCl3): δ 27.23, 29.37, 32.49, 44.32, 115.45, 115.73, 132.02, 132.06, 132.11, 132.22, 135.65, 135.68, 135.78, 160.94, 164.26, 190.22 (Table 2, entry 18).
4.3.2 2,6-bis(4-bromobenzylidene)-4-(tert-butyl)cyclohexanone
Yellow crystal; m.p. = 161–164 °C; IR (KBr) (cm−1) νmax: 2958, 2867, 1662, 1604, 1487, 1074; 1H NMR (300 MHz, CDC13) 0.94 (s, 9H), 1.47 (tt, J = 12.8 Hz/3.2 Hz, 1H), 2.40 (m, 2H), 3.09 (dd, J = 15.5 Hz/2.3 Hz, 2H), 7.68 (s, 2H), 7.30–7.56 (m, 8H, ArH); 13C NMR (75 MHz, CDCl3): δ 27.22, 29.42, 32.51, 44.26, 122.89, 131.71, 134.73, 135.76, 136.48, 190.06 (Table 2, entry 19).
4.4 General procedure for the synthesis of xanthenes (5)
A mixture of α,α′-bis(substituted-benzylidene)cycloalkanones (1 mmol), dimedone (1 mmol) and 0.075 mmol (0.012 g) n-TSA was added in a test tube and heated in an oil bath at 130 °C under solvent-free condition for an appropriate time. After completion of the reaction (monitored by TLC), the reaction mixture was cooled, eluted with hot ethanol (5 mL) and was centrifuged to filter the catalyst. The products were obtained in white powder by recrystallization of ethanol solution.
4.5 Spectral data of new compounds
4.5.1 (E)-3,3-dimethyl-9-(naphthalen-2-yl)-5-(naphthalen-2-ylmethylene)-2,3,4,5,6,7,8,9-octahydro-1H-xanthen-1-one
White powder; m.p. = 208–210 °C; IR (KBr) (cm−1) νmax: 3421, 3051, 2941, 1622, 1660, 1220, 748; 1H NMR (300 MHz, CDC13) 1.04 (s, 3H), 1.15 (s, 3H), 1.65 (m, 2H), 2.17 (m, 4H), 2.66 (m, 3H), 2.86 (m, 1H), 4.42 (s, 1H), 7.16 (s, 1H), 7.39–7.84 (m, 14H, ArH); 13C NMR (75 MHz, CDCl3): δ 22.46, 27.31, 27.42, 27.75, 29.39, 32.18, 40.53, 41.39, 50.82, 112.42, 118.30, 122.20, 125.40, 125.83, 126.17, 126.54, 127.02, 127.53, 127.60, 127.92, 128.01, 128.08, 130.67, 132.12, 132.49, 133.27, 133.40, 134.90, 141.47, 142.01, 164.13, 197.22 (Table 7, entry 13).
4.5.2 (E)-5-benzylidene-7-(tert-butyl)-3,3-dimethyl-9-phenyl-2,3,4,5,6,7,8,9-octahydro-1H-xanthen-1-one
White powder; m.p. = 173–176 °C; IR (KBr) (cm−1) νmax: 3024, 2956, 1660, 1627, 1380, 1340, 1224, 769; 1H NMR (300 MHz, CDC13) 0.78 (s, 9H), 1.00 (s, 3H), 1.10 (s, 3H), 1.25 (m, 1H), 1.96 (m, 2H), 2.18 (m, 3H), 2.52 (m, 2H), 3.03 (dd, J = 14.70 Hz/2.76 Hz, 1H), 4.23 (s, 1H), 6.93 (s, 1H), 7.15–7.40 (m, 10H, ArH); 13C NMR (75 MHz, CDCl3): δ 27.14, 27.23, 27.33, 27.91, 28.22, 29.33, 32.15, 32.18, 39.69, 41.36, 43.86, 50.82, 112.65, 118.39, 121.80, 126.46, 126.50, 128.19, 128.42, 129.21, 130.95, 137.43, 142.18, 143.97, 164.30, 197.19 (Table 7, entry 14).
4.5.3 (E)-7-(tert-butyl)-3,3-dimethyl-5-(4-methylbenzylidene)-9-(p-tolyl)-2,3,4,5,6,7,8,9-octahydro-1H-xanthen-1-one
White powder; m.p. = 207–212 °C; IR (KBr) (cm−1) νmax: 3020, 2952, 1662, 1629, 1379, 1365, 1110, 833; 1H NMR (300 MHz, CDC13) 0.78 (s, 9H), 1.00 (s, 3H), 1.10 (s, 3H), 1.25 (m, 1H), 1.99 (m, 2H), 2.20 (m, 3H), 2.26 (s, 3H), 2.37 (s, 3H), 2.53 (m, 2H), 3.04 (d, J = 6.3 Hz, 1H), 4.18 (s, 1H), 6.89 (s, 1H), 7.04–7.26 (m, 8H, ArH); 13C NMR (75 MHz, CDCl3): δ 21.08, 21.21, 27.18, 27.27, 27.38, 27.47, 27.86, 28.24, 29.33, 32.15, 32.21, 39.24, 40.56, 41.37, 43.76, 50.85, 112.78, 117.80, 118.16, 121.58, 127.96, 128.26, 128.87, 128.92, 129.01, 129.14, 130.34, 130.59, 134.56, 135.81, 135.91, 136.20, 141.01, 141.14, 163.74, 164.24, 197.23 (Table 7, entry 15).
4.5.4 (E)-7-(tert-butyl)-5-(4-chlorobenzylidene)-9-(4-chlorophenyl)-3,3-dimethyl-2,3,4,5,6,7,8,9-octahydro-1H-xanthen-1-one
White powder; m.p. = 249–253 °C; IR (KBr) (cm−1) νmax: 2960, 1662, 1627, 1379, 1089; 1H NMR (300 MHz, CDC13) 0.77 (s, 9H), 0.98 (s, 3H), 1.10 (s, 3H), 1.25 (m, 1H), 1.98 (m, 2H), 2.20 (m, 3H), 2.53 (m, 2H), 2.96 (dd, J = 14.65 Hz/2.78 Hz, 1H), 4.21 (s, 1H), 6.86 (s, 1H), 7.23–7.35 (m, 8H, ArH); 13C NMR (75 MHz, CDCl3): δ 27.12, 27.20, 27.28, 27.91, 28.14, 29.30, 32.16, 32.18, 39.16, 41.32, 43.88, 50.75, 112.33, 118.19, 120.94, 128.40, 128.51, 129.48, 129.77, 130.43, 131.35, 132.18, 132.30, 135.75, 142.27, 142.44, 163.81, 164.33, 197.11 (Table 7, entry 16).
4.5.5 (E)-7-(tert-butyl)-5-(4-flurobenzylidene)-9-(4-fluorophenyl)-3,3-dimethyl-2,3,4,5,6,7,8,9-octahydro-1H-xanthen-1-one
White powder; m.p. = 228–233 °C; IR (KBr) (cm−1) νmax: 2958, 1652, 1600, 1380, 1222, 1157; 1H NMR (300 MHz, CDC13) 0.77 (s, 9H), 0.99 (s, 3H), 1.11 (s, 3H), 1.24 (m, 1H), 1.95 (m, 2H), 2.18 (m, 3H), 2.52 (m, 2H), 2.96 (dd, J = 14.63 Hz/2.83 Hz, 1H), 4.22 (s, 1H), 6.88 (s, 1H), 6.91–7.30 (m, 8H, ArH); 13C NMR (75 MHz, CDCl3): δ 27.10, 27.27, 27.86, 28.12, 29.29, 32.16, 38.94, 41.33, 43.95, 50.78, 112.55, 114.84, 115.02, 115.13, 115.31, 118.09, 120.93, 129.80, 129.90, 130.67, 130.70, 130.77, 133.32, 133.37, 139.67, 139.71, 142.20, 159.81, 159.89, 163.08, 164.22, 197.17 (Table 7, entry 17).
4.5.6 (E)-7-(tert-butyl)-5-(4-bromobenzylidene)-9-(4-bromophenyl)-3,3-dimethyl-2,3,4,5,6,7,8,9-octahydro-1H-xanthen-1-one
White powder; m.p. = 250–254 °C; IR (KBr) (cm−1) νmax: 3056, 2866, 1658, 1625, 1380, 1064; 1H NMR (300 MHz, CDC13) 0.79 (s, 9H), 0.98 (s, 3H), 1.12 (s, 3H), 1.26 (m, 1H), 2.03 (m, 2H), 2.18 (m, 3H), 2.53 (m, 2H), 2.80 (m, 1H), 4.20 (s, 1H), 6.95 (s, 1H), 7.16–7.35 (m, 8H, ArH); 13C NMR (75 MHz, CDCl3): δ 26.60, 27.05, 28.04, 30.40, 32.31, 33.04, 39.59, 41.93, 43.93, 50.77, 113.57, 118.04, 121.13, 128.32, 128.43, 129.72, 130.48, 130.53, 132.27, 132.37, 135.67, 141.93, 142.60, 165.75, 197.33 (Table 7, entry 18).
Acknowledgements
We thank the Department of Chemistry of Semnan University for supporting this work.