1 Introduction
The non-molecular compounds consisting of metal ions and organic ligands, whose assembly leads to one-, two-, and three-dimensional networks, are commonly known as coordination polymers [1]. In recent years, there has been a tremendous interest in the design and synthesis of coordination polymers that have different metal ions or metal clusters connected through supramolecular contacts, such as hydrogen bonding, π···π or CH···π stacking, van der Waals contacts or their combinations [2–5]. Generally, the synthetic strategy in building such networks rests on the basis of appropriate bridging ligands containing O- or N-donor atoms that can bind metal ions in different ways of coordination and provide a likely way to achieve the desired structures, which depend both on the geometry of the ligand and the coordination trend of the metal ions [6]. 1,10-Phenanthroline and some of its derivatives such as 1,10-phenanthrolone-5,6 diamine, 1,10-phenenthroline-5,6-dione (phendione) and 1,10-phenanthroline-5,6-dioxime can play important roles as molecular frameworks [7–9]. However, a major challenge in the preparation of these compounds is the predictability of the polymeric structures because many factors such as coordination geometry of the metals, solvent system, nature of the ligand, metal-to-ligand ratio, pH value, counter ions and temperature will affect framework construction [10–13]. Stable, inert complexes containing an extended aromatic heterocyclic ligand, which can intercalate into DNA, are extremely valuable as probes of biological systems. Recently, the novel ligand tetrapyrido [3,2-a: 2’, 3’-c: 3”, 2”-h: 2”’, 3”’-j] phenazine (tpphz) was synthesized (Scheme 1) [14,15]. Studies on its complexes are mainly focused on their intense metal-to-ligand charge transfer, their photo-initiated energy transfer, sensitization processes, and water splitting devices. However, tpphz is suitable for being used as an intercalating ligand owing to its flat, large surface area and special geometry, which permit overlapping between the aromatic ring of the intercalating ligand and the base pairs in DNA. Tpphz is the first example of a rod-like polypyridine bridging ligand, has large defined length, conjugated character, good stability and fully rigid aromatic structure for the assembly of coordination polymers [16]. It may serve as a terminal or planar bridging ligand in the construction of the versatile complexes; however, the very low solubility of these long polyaromatic ligands, due to strong π···π interactions, can hinder their synthesis and the preparation of their complexes [17]. It has been recently used in the design and synthesis of a variety of dyads [18,19] and tetrads [16] suitable for the study of intramolecular photo-induced energy or electron transfer. Related more extended aromatic bridging ligands have also been developed [20]. Recently, we have reported coordination polymers constructed from N-donor ligands and one of the most interesting one was prepared using tpphz [21]. Here we continue our research by preparing and characterizing the tpphz ligand with the anion, which, to the best of our knowledge, has not been reported yet and polymers were generally mononuclear and binuclear complexes of Ru (II) and Os (II) [22–25]. However much to our surprise, in our synthesis we got the tpphz polymeric compound in nanocrystalline form with as the counter ion, which has not been reported so far [24]. The structural analysis of this nanocrystalline compound has been carefully done.

Schematic representation of the tpphz ligand.
2 Experimental
2.1 Materials and methods
1,10-phenanthroline-5,6-dione (phendione) as a precursor for the synthesis of tpphz and tpphz were prepared according to the literature methods [25,26] and all other chemicals and solvents were purchased from Merck Company. Elemental analyses(C, H and N) were carried out with a PerkinElmer elemental analyzer. FT–IR spectra were recorded with a Bruker Equinox-55 spectrometer equipped with a single reflection diamond ATR system in the range from 400 to 4000 cm−1. Scanning electron microscopy (SEM) was performed with an Oxford LEO 1445 V device.
2.2 Synthesis
The tpphz ligand was prepared in accordance with the literature method. A mixture of 1,10-phenanthroline-5,6-dione (phendione) (1.05 g, 4.8 mmol), ammonium acetate (5.43 g, 70.44 mmol), and sodium hydrosulfite (0.12 g, 0.62 mmol) was heated to 180 °C for 2 h under argon atmosphere. After addition of water (100 ml), the precipitate was added to the refluxing ethanol followed by hot filtration.
In order to prepare the compound, a solution of (0.129, 1 mmol) in water (5 ml) was combined with a solution of tpphz (0.19 mg, 0.5 mmol) in hydrochloric acid (37%, 10 ml) and stirred for some minutes. The reaction mixture was transferred into a 25-ml Teflon-lined stainless steel autoclave and maintained at 170 °C for 96 h under autogenous pressure. While cooled to room temperature at the rate of 10 °C·h−1, green needle-liked crystals were obtained. Yield: 60%. Anal. calc. for C16H9.33Cl2.67Co0.67N4 (Mw = 391.43 g/mol): C, 49.05; H, 2.30; N, 14.3. Found: C, 48.90; H, 2.22; N, 13.94. IR (cm−1): 3041.48(br), 2332.18(w), 1612.02(m), 1577.24(m), 1525.93(m), 1461.38(m), 1314.24(m).
2.3 X-ray analysis
The compound's crystallographic data and structure refinement parameters are summarized in Table 1. Data were collected using Mo Kα X-ray radiation by means of an Oxford Instrument Xcalibur 3 with a Sapphire CCD detector for the current compound. The CrysAlis program packages were used for indexing and integrating the single-crystal reflections. The structure was solved by direct methods and refined using full-matrix least squares on F2 using SHELXS97 and SHELXL97 [27]. Non-hydrogen atoms were treated anisotropically. All the aromatic hydrogen atoms in the compound were introduced at the calculated positions except for NH, which was located on the electron density map and constrained to ride on the parent nitrogen atom with Uiso (H) = 1.2 Ueq. The crystallographic data for the structure have been deposited with the Cambridge Crystallographic Data Centre, CCDC 866087. This number contains the supplementary crystallographic data for this paper. These data can be obtained free of charge from The Cambridge Crystallographic Data Centre via www.ccdc.cam.ac.uk/data_request/cif (Fig. 1).
Crystal data and structure refinement for the studied compound.
Empirical formula | C16H9.33Cl2.67Co0.67N4 |
Formula weight | 391.43 |
Temperature (K) | 293 (2) |
Crystal system | Monoclinic |
Space group | C 1 2/c 1 (No. 15) |
a (Å) | 9.5916 (19) |
b (Å) | 22.957 (5) |
c (Å) | 10.583 (2) |
α (°) | 90.00 |
β (°) | 87.88 (1) |
γ (°) | 100.20 (3) |
Volume (Å3) | 90.00 |
Z | 6 |
Dc (g/cm3) | 1.700 |
θ range for data collection | 2.33° to 30.04° |
μ (mm−1) | 1.243 |
F (000) | 1180 |
Range of h, k, l | –13/13, –32/32, –14/14 |
Reflection measured/unique | 13,254/3366 |
Goodness-of-fit on F2 | 1.082 |
Data/restraints/parameters | 1496/3/187 |
Final R indices [I > 2σ(I)] | R1 = 0.0304, wR2 = 0.0869 R1 = 0.0282, wR2 = 0.0849 |
R indices (all data) |
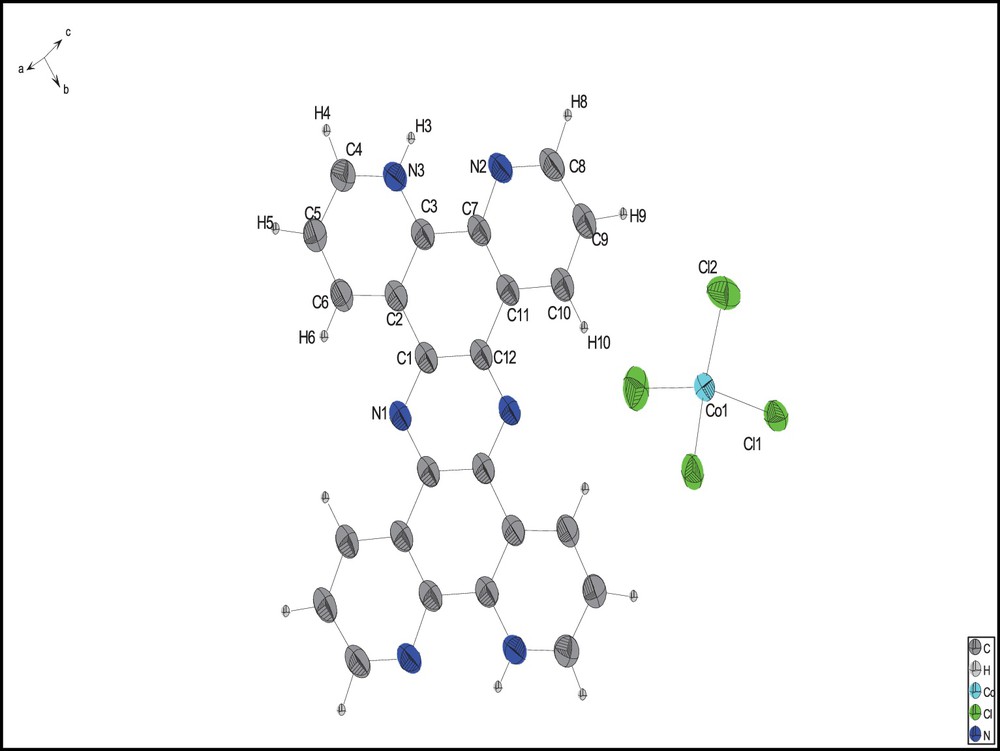
(Color online.) Molecular structure of the studied compound with 50% probability displacement ellipsoids. H atoms are shown as circles of arbitrary radii.
2.4 Computational details
Calculations were carried out using Guassian 09 package program [28]. In the computational model, the anion was ignored and the di-cationic compound was taken into account. The optimized geometry of [H2tpphz]2+ was verified by performing a frequency calculation. Density functional theory (DFT) was carried out on the gas phase of compound using the becke three-parameter hybrid exchange [29] and the Lee–Yang–Parr correlation hybrid functional [30] (B3LYP) and 6–31 + G (d, p) basis set used for all atoms. The vibrations in the calculated vibrational spectrum were real, thus the optimized geometry of [H2tpphz]2+ corresponds to a true energy minimum.
3 Result and discussions
3.1 IR spectra
The{[H2tpphz][CoCl4]}n compound has been obtained in the reaction of the cobalt(II) chloride with tetrapyrido[3,2-a:2’,3’-c:3”,2”-h:2”’,3”’-j] phenazine (tpphz). Due to the low solubility of tpphz, this reaction was performed under hydro/solvothermal condition. The FT–IR spectrum of {[H2tpphz][CoCl4]}n clearly shows the characteristic bands of the C = C and C = N stretching modes of tetrapyrido[3,2-a:2’,3’-c:3”,2”-h:2”’,3”’-j] phenazine (tpphz) in the range from 1520 to 1650 cm−1 [31]. The calculated (non-scaled) vibrational spectrum of {[H2tpphz][CoCl4]}n stays in good agreement with experimental data, as shown in Fig. 2. These values are higher than the experimental ones by ∼ 3%, which is a usual feature for this approach. Similarly, the calculated absorptions due to the m(C = N), m(C = C) modes of the tpphz, appearing at 1510-1650 cm−1, fall in the experimental range. The band at 3300 cm−1 is consistent with the N–H stretching vibrations of the aromatic rings, respectively [32].
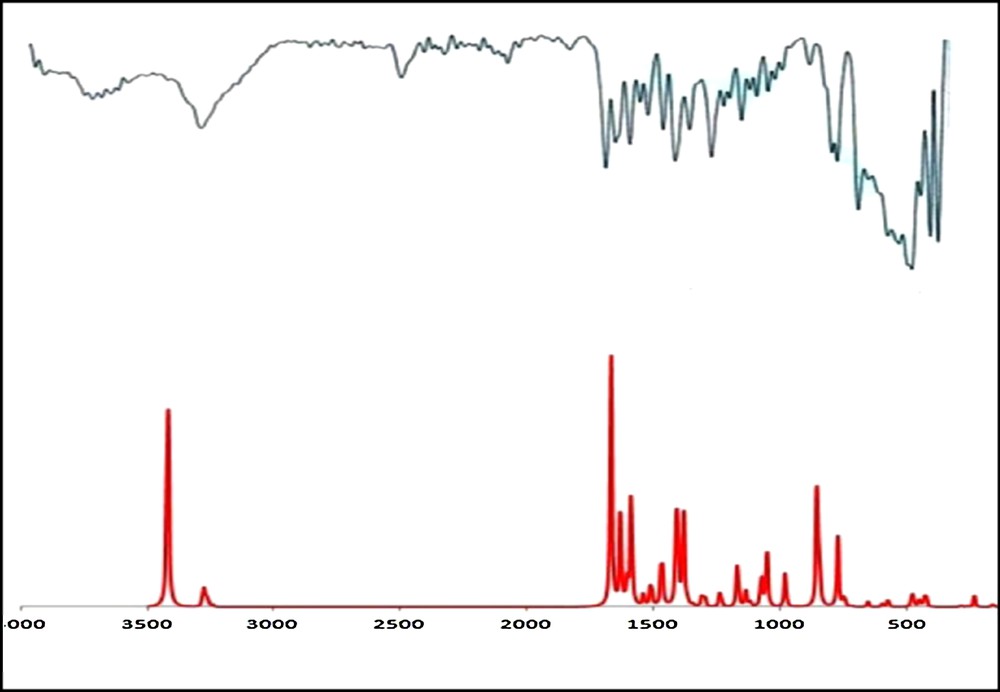
(Color online.) Experimental and calculated (non-scaled) vibrational spectra of [H2tpphz]2+.
3.2 Description of the crystal structure
The compound crystallizes in the monoclinic system with C 1 2/c 1 (No. 15) space group, with Z = 6. The presence of large amounts of H+ in the initial solution used for the synthesis may be considered as a reason for the protonation and as providing the di-protonated cation together with as a counter ion. Selected crystallographic and experimental details are summarized in Table 1 and the selected bond lengths and angles are presented in Table 2. The molecular structure and the atom-numbering scheme are shown in Fig. 1. N-protonation causes neither planar nor angular deviation from the theoretical planes and angles compared to the non-protonated ligand. Each tetrahedron forms four hydrogen bonds that not only stabilized the assembled layers of H2tpphz2+ ligand, but also maintain two H2tpphz2+ units nearby in one layer (Fig. 3). Hydrogen bond distances for interactions within layers are 2.313 and 2.792 Å and are 2.792 and 3.004 Å for interactions between layers. It is worth mentioning that there are also π···π interactions between centroid centers of the H2tpphz2+ rings, with distances of 3.503 and 3.890 Å. The mentioned interactions are responsible for the layered structure in which tetrahedra are arrayed between the layers (Fig. 4).
The selected experimental and optimized bond lengths (Å) and angles (°) for the compound.
Bond distance (Å) | Bond angles (°) | ||||
Experimental | Optimized | Experimental | Optimized | ||
C2—C3 | 1.395 (14) | 1.4056 | C2–C3–C7 | 122.35 (12) | 120.162 |
C2—C1 | 1.456 (2) | 1.4598 | C4–N3–C3 | 123.35 (14) | 123.26 |
N3–C4 | 1.336 (11) | 1.3508 | C1–C12–C11 | 120.44 (11) | 120.58 |
N3–C3 | 1.350 (2) | 1.3635 | N1–C1–C2 | 117.79 (12) | 118.75 |
C5–C6 | 1.377 (2) | 1.3974 | N2–C7–C3 | 116.89 (12) | 116.35 |
C5–C4 | 1.384 (15) | 1.3951 | C7–C11–C10 | 117.84 (13) | 117.04 |
N1–C1 | 1.337 (14) | 1.3460 | C8–N2–C7 | 116.42 (13) | 118.19 |
N1–C12 | 1.337 (2) | 1.3494 | N3–C4–C5 | 119.80 (15) | 119.22 |
C7–C11 | 1.399 (2) | 1.4105 | C5–C6–C2 | 120.23 (15) | 120.24 |
C7–C3 | 1.453 (13) | 1.4447 | H3–N3–C3 | 118.78 | 114.15 |
N3–H3 | 0.855 | 1.026 | C6–C2–C1 | 122.51 (13) | 123.90 |
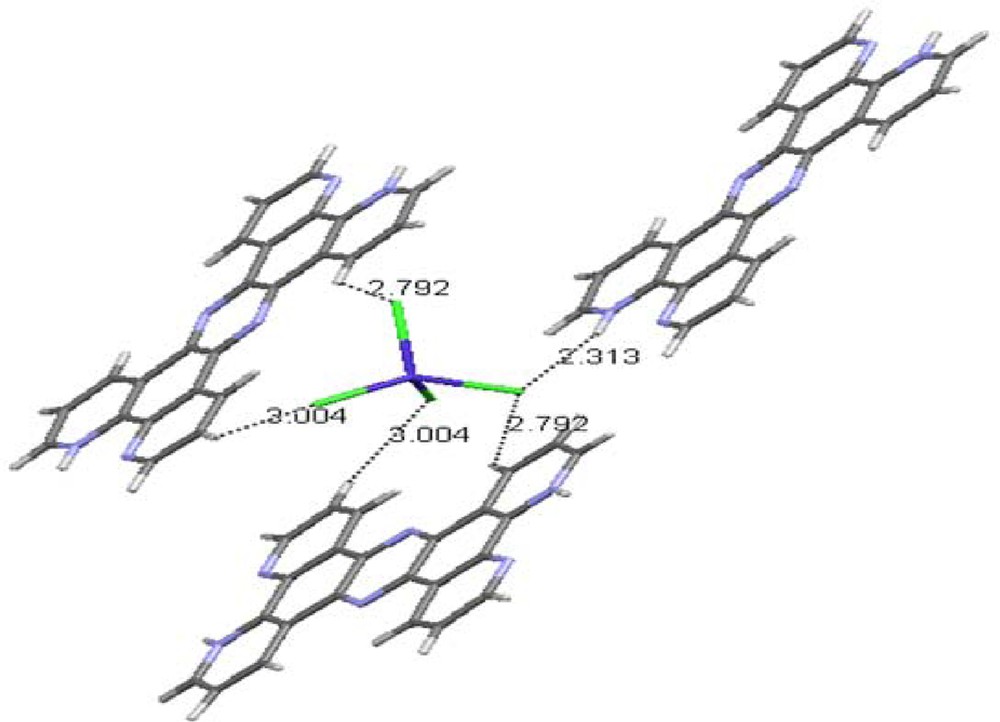
(Color online.) The hydrogen bonding that stabilizes between layers.
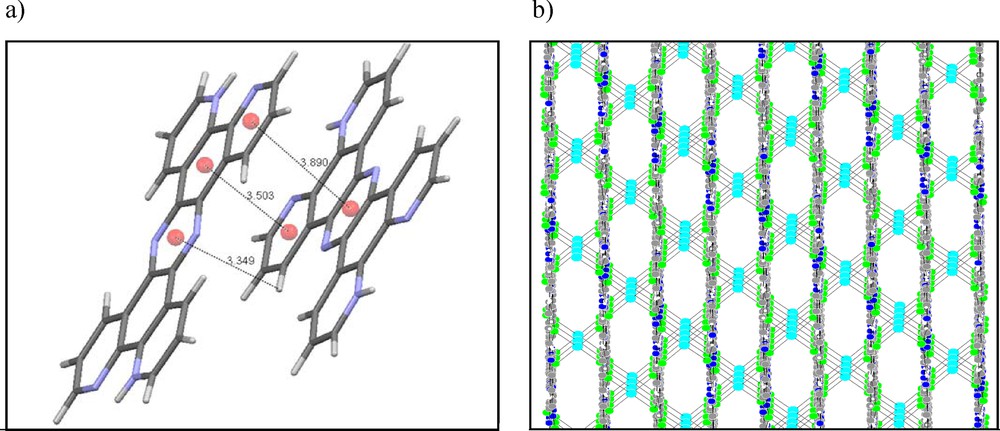
(Color online.) (a) π···π and C–H···π interactions, and (b) stacking arrays of layers along the b axis in the studied compound.
3.3 Scanning Electron Microscopy (SEM)
The compound's morphology, characterized by SEM, is shown in Fig. 5. Crystals of the compound were relatively uniform rectangular prisms without any agglomeration. The size of the crystals was about 80 nm in diameter. The large quantity of monodispersed rectangular prisms reveals the significance of our easy synthetic procedure to fabricate a nanosized polymeric compound.
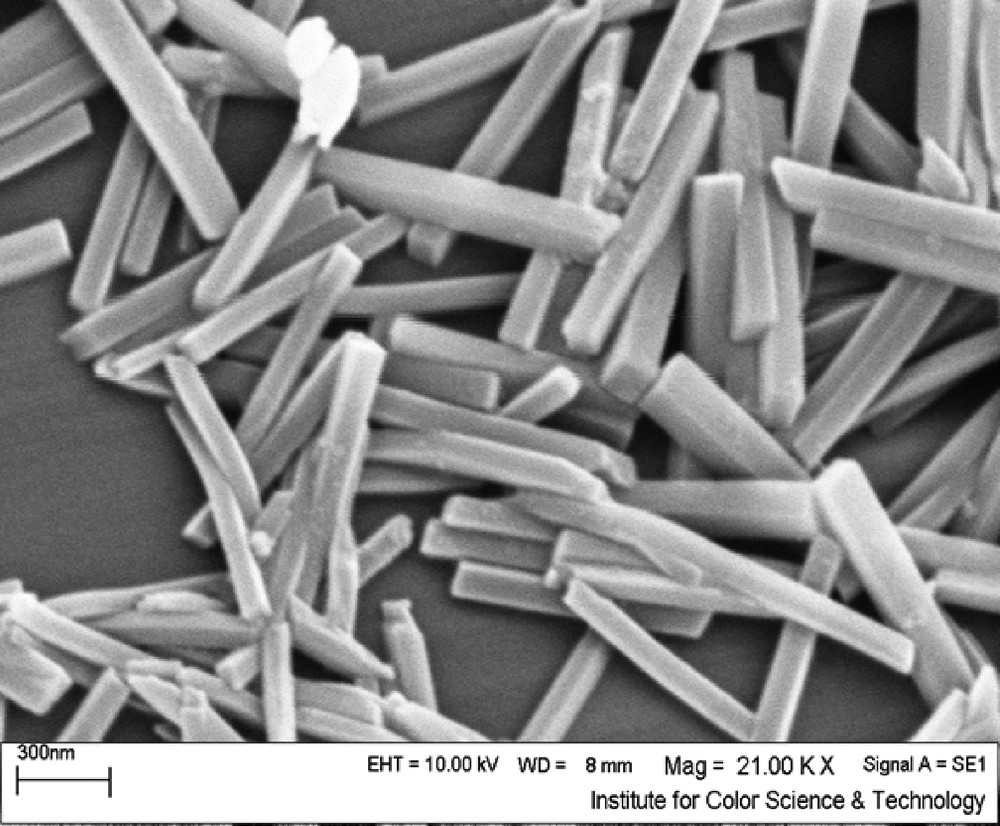
SEM image of the studied compound.
3.4 Topology
In view of topology, by considering all the weak H-bonding interaction, if we simplify the tpphz2+ ligand (pink ball) and ion (gray ball) as 6-connected nodes, the 3D architecture can be classified as the (6,6)-connected (413. 62)(48. 66. 8) network (Fig. 6a). The vertex symbol for this network is [4.4.4.4.43.43.43.43.62.62.62.62.820.820.832] [4.4.4.4.4.4.4.4.4.4.42.42.42.820.820]. However, by excluding the weak H-bonding, then the simplified structure will be turned to zigzag chains (Fig. 6b).
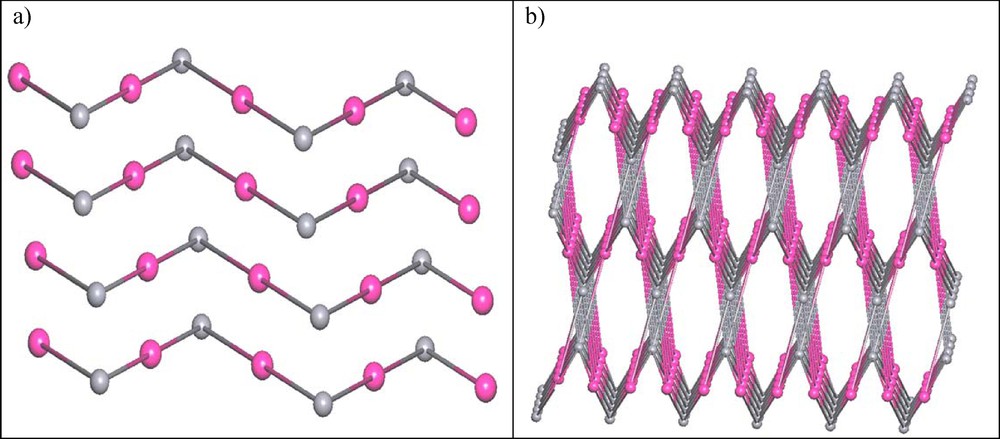
(Color online.) Topology view of the studied compound: (a) layered structure and (b) zigzag chain.
3.5 Geometry optimization
The geometry of [H2tpphz]2+ was optimized in a singlet state using the DFT method with the B3LYP functional in the gas phase. The calculated geometric parameters are gathered in Table 2. The optimized geometry of [H2tpphz]2+ displays a planar structure and general trends observed in the experimental data are satisfactorily reproduced in the calculations (Fig. 7).
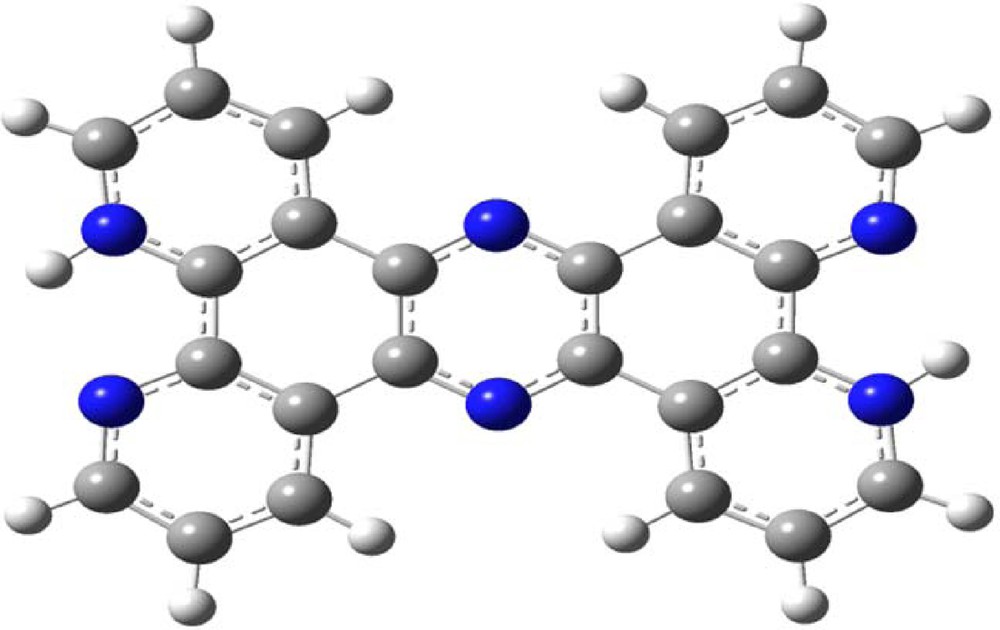
(Color online.) Optimized geometry of the studied compound.
A schematic illustration of the energy and character of the frontier orbitals of the cation [H2tpphz]2+ is shown in Fig. 8. For the compound, the energy between the Highest Occupied Molecular Orbital (HOMO) and the Lowest Unoccupied Molecular Orbital (LUMO) is about 3.5 eV. The highest MO presents a significant pz character (28% for the N atom and 72% for the other ones). LUMO presents a lesser pz character compared to HOMO.
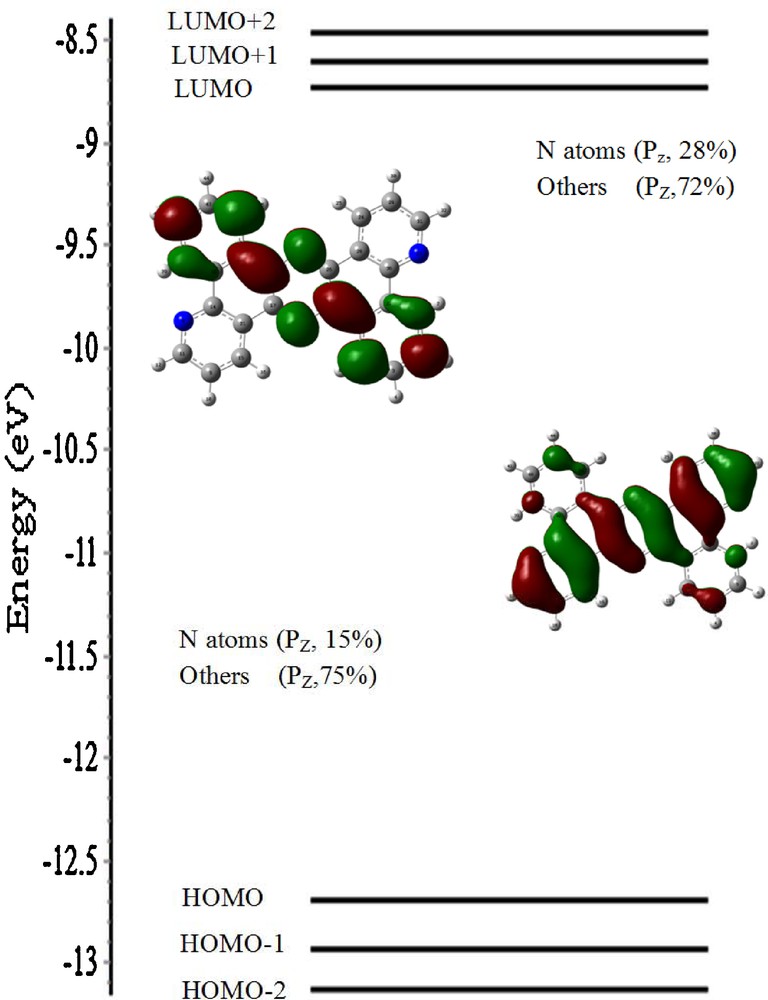
(Color online.) Energy (eV), character, and some contours of the unoccupied molecular orbitals of [H2tpphz]2+.
4 Conclusion
In summary, we have successfully synthesized new polymeric compound based on the H2tpphz2+ and CoII cation through a hydrothermal process. The compound crystallized in the monoclinic system, with C 1 2/c 1 (No. 15) space group. Various hydrogen bonding types and π···π and C–H···π interactions give this compound a layered structure. Computational studies with Density Functional Theory (DFT) have been done and show a good agreement between experimental and theoretical results. Scanning electron microscopy also showed that the compound is a nanosized polymeric compound, which is particularly wonderful for these types of material. The experimental and calculated IR spectra are pretty consistent with each other.
Acknowledgements
We gratefully acknowledge a grant from the University of Tehran and Dr. Nahid Hasani, from the University of Mazandaran, for her excellent guidance in computational sections. We are also grateful to Prof. Peter. H. M. Budzelaar and Mark Cooper from University of Manitoba for gathering the crystallographic data.