1 Initial descriptions by Alexander Randall and contemporaries
Alexander Randall, an American urologist, proposed in 1936 a new theory relative to “The origin and growth of renal calculi” [1,2]. Based upon extensive forensic studies performed between 1935 and 1938, he observed, at the tip of the renal papillae, lesions consisting of calcium phosphate and carbonate deposits accumulating in the kidney interstitium. These lesions were initially interstitial but their growth was likely to result in urothelium rupture and formation of plaque in contact with urine. He observed small kidney stones made of calcium oxalate in 65/1154 pairs of kidneys (2.8%). Overall, plaques were present in 19.6% of kidneys [2]. He also observed that calcium oxalate stones expelled by patients harboured in some cases a depression due to papilla imprinting and calcium phosphate residues, involving thereby plaques as the origin of stones (Fig. 1). Alexander Randall distinguished two ways for stone initiation: on the one hand, calcium phosphate deposits may form typical plaques in the renal interstitium leading to urothelium rupture in some cases (papillary lesion type 1); on the other hand, calcium phosphate plugs may be generated in the collecting duct, at the outlet of the tubule (papillary lesion type 2). In both scenarios, calcium phosphate initiates further a calcium oxalate stone heterogeneous nucleation process.
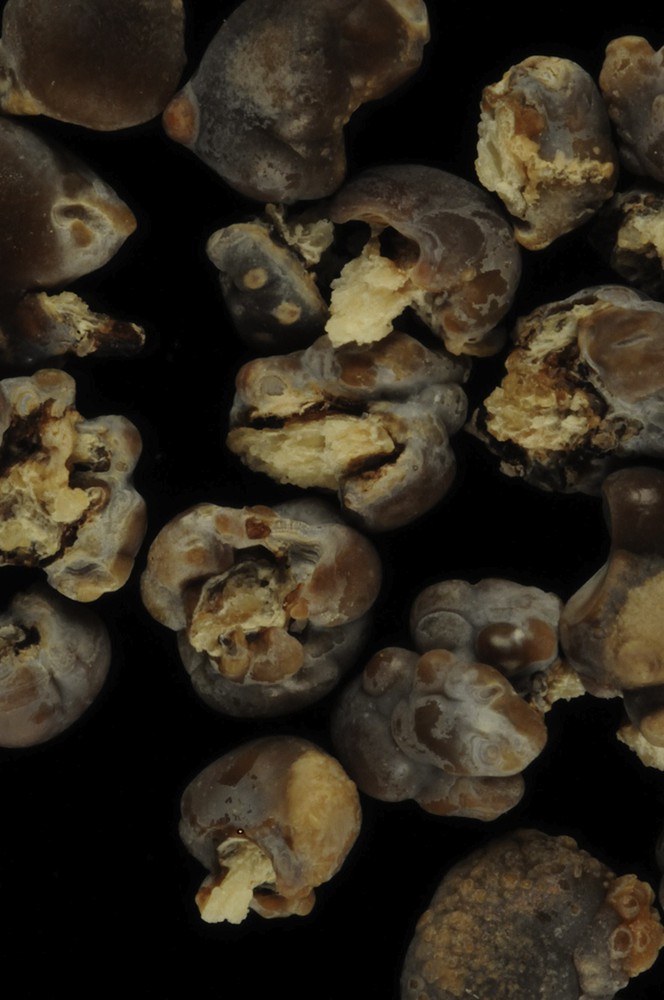
Typical monohydrate calcium oxalate stones (type Ia, brown structures) harbouring papillary umbilication and Randall’s plaque made of apatite (white structures).
Several authors were interested in kidney calcifications during the same period. Rosenow observed plaques in 22% of 239 kidneys and Vermooten in 17.2% of Caucasian patients' kidneys [3,4]. The calcifications were present in the interstitium but not in the tubule lumen [5]. Anderson found microscopic evidence for “calcareous” plaques or “tiny stones” in a vast majority of 148 surgically removed kidneys and 20 apparently normal autopsy kidneys [6]. He postulated that the calcareous material would originate from the high concentration of calcium and other ions in the interstitium. Phagocytic cells would in turn absorb this material and die, leading to the formation of calcareous “droplets”.
Despite these seminal studies, Randall’s plaque generated limited interest during the second part of the 20th century. One of the reasons underlying this loss of attention was the discrepancy between the clinical peak stone age and the peak of Randall’s plaque, assessed through forensic studies, respectively, before and after the fifth decade of life in those days. Nevertheless, Cifuentes-Delatte et al. reported in the eighties a typical umbilication in 142/500 stones, evidencing their papillary origin [7,8]. Eighty seven stones were studied in detail: 63 of these stones exhibited Randall’s plaque residues. Electron microscopy revealed that 13 of these 63 plaques exhibited calcified tubules, demonstrating their intrapapillary origin (Fig. 2).
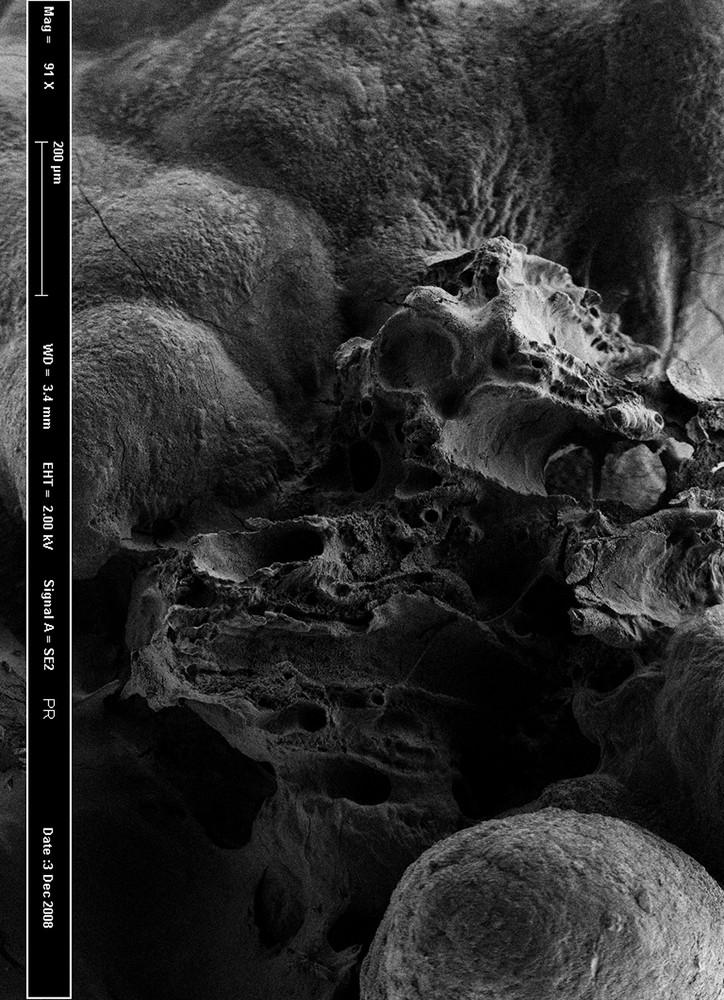
Scanning electron microscopy of a Randall’s plaque at the stone surface, showing tubular structure remnants.
2 Renewed interest in Randall’s plaque and recent advances
During the past two decades, interest in Randall’s plaque increased, probably as a result of two factors, the development of reno-ureteroscopy and a potential increase in the incidence of Randall’s plaque related stones. First, the development of new urological techniques allowed detailed examination of renal cavities and the direct visualization of Randall’s plaques. Low and Stoller reported in 1997 the presence of Randall’s plaques on one or more papillae in 74% of 57 patients who underwent ureteroscopic or percutaneous stone removal. In 7 patients who underwent reno-ureteroscopy for conditions unrelated to kidney stones, only 3 (43%) had papillary plaques [9]. In 2006, Matlaga et al found that 91% of papillae in 23 patients with idiopathic calcium oxalate nephrolithiasis contained plaques [10]. They evidenced that calcium oxalate stones were actually attached on Randall’s plaques. More recently, Linnes et al. reported that 99% of renal stone formers had Randall’s plaque on at least one papilla [11]. In France, prevalence of Randall’s plaque seems lower: Olivier Traxer found Randall’s plaques in 57% of 287 stone formers who underwent flexible uretero-renoscopy. Plaques were visible in only 27% of 173 non-stone forming patients [12,13].
Another matter of interest is that Randall’s plaque-related stone prevalence is likely to have increased during the past decades. Calcium oxalate stones are nowadays the main type of urolithiasis in western countries. Longitudinal studies performed in USA, Europe or Japan [14–17] report an increase in calcium oxalate stone prevalence during the last few decades. The risk factors involved in calcium oxalate stone formation are well documented: low diuresis and imbalance in the diet resulting in hypercalciuria, hyperoxaluria and/or hypocitraturia [18,19]. There is no longitudinal study taking into account Randall’s plaque related stones. Nevertheless, in France, the proportion of stones harbouring an umbilication and typical plaques was found to be three times more frequent in recent years than three decades ago, especially in young patients [20]. Children may form calcium oxalate stones from Randall’s plaque [21]. Taking into account the increased proportion of Randall’s plaque related stones and the increase in calcium oxalate stone prevalence, it may be hypothesized that Randall’s plaque-related stone prevalence may have increased during the past decades.
A major contribution in the field of Randall’s plaque dedicated research has been made by Evan et al. in 2003 [22]. They took advantage of kidney biopsies performed in renal stone formers during percutaneous nephrolithotomies. These biopsies were performed in upper, middle and lower poles of papillae. They observed Randall’s plaque at the tip of the renal papillae in the subepithelial position and characterized calcium-containing structure deposits surrounding Henle’s loop. These deposits were identified by infrared microspectroscopy to be made of apatite and carbonate. Electron microscopy evidenced that they were located in the basement membrane of Henle’s loop but not in the cell cytoplasm. These observations suggest that Randall’s plaque apatite droplets form in Henle’s loop thin limb basement membrane, spread in the interstitium and form plaques. By contrast, Evan et al. observed in patients affected by enteric hyperoxaluria due to jejuno-ileal bypass an initial crystallization site in collecting duct lumen, distinct from Randall’s plaque, confirming eighty years later the observations made by Alexander Randall.
The mechanisms underlying the formation of carbonated apatite deposits remain elusive. Asplin et al. have hypothesized that calcium phosphate supersaturation in the thin limb of Henle’s loop would promote calcium phosphate particle precipitation [23]. Some particles attached to epithelial cells may actually undergo an endocytosis process and precipitate in basement membranes [24]. The precipitation would be favoured by the presence of collagen and mucopolysaccharides. Once initiated, the interstitial calcification process would extend in the interstitium, forming plaques made of carbonated apatite at the contact of macromolecules such as osteopontin [25].
3 Unsolved questions and future challenges
3.1 Randall’s plaque epidemiology: does Randall’s plaque-related stone incidence increase?
Several longitudinal studies performed in western countries have shown that calcium oxalate stones are more and more frequent and represent in some series more than 90% of whole stones [14–17]. To date there is no longitudinal study specifically dedicated to Randall’s plaque-related stones. Several limitations make it difficult to perform such studies. Actually, few laboratories are able to detect and analyse the tiny calcifications within umbilication evidencing thereby that stones result from Randall’s plaque. Moreover, the increased use of fragmentation techniques such as shockwaves, ultrasounds or laser promotes the loss of Randall’s plaque fragments fixed to the stone. It becomes thereby impossible to identify the renal origin of these fragmented stones. However, the concomitant increased proportion of Randall’s plaque related intact stones suggested by some series and the observation that young patients and children expelled more and more frequently Randall’s plaque-related stones during the past years make us prompt to hypothesize that Randall’s plaque could explain in part the increased incidence of calcium oxalate stones [12]. There is therefore a need to assess which environmental factors may affect the urine composition and would affect Randall’s plaque formation.
3.2 Which are Randall’s plaque biological determinants?
Kuo et al. have shown that hypercalciuria, low diuresis and low pH correlated with the presence of Randall’s plaque in renal stone formers [26]. Worcester et al. accumulated further lines of evidence that Randall’s plaque growth was related to increased calcium concentration in distal tubular fluid, promoting calcium-phosphate supersaturation and precipitation [27]. Interestingly, Vermooten examined 1060 pairs of kidneys in South Africa during the middle of the past century [4]. He identified Randall’s plaques in 17.2% of Caucasians and only in 4.3% of Bantus, the local native Africans, who are known to have lower calciuria and to be less affected by calcium-related stones.
Hypercalciuria would therefore be responsible for a two-step process: Randall’s plaque formation and calcium oxalate stone formation once plaque has eroded the urothelium. The two main dietary determinants of hypercalciuria are salt and protein intakes [28]. As a consequence, our modern lifestyle and inappropriate diet enriched with fat, proteins and salt may theoretically favour Randall’s plaque formation and calcium oxalate stones as well. In addition, the widespread use of vitamin D supplementation in children during the past decades, especially in the USA but also in Europe raises some questions. Actually, some studies highlighted a relationship between vitamin D intakes and stone formation [29].
Further studies are required to analyze the diet, vitamin D intakes and serum levels, and urine composition in renal stone formers affected by Randall’s plaque.
3.3 What are Randall’s plaque and related stones made of?
Alexander Randall identified that plaques were made of calcium phosphate and subsequent studies reported plaques made of hydroxyapatite. Evan et al. described plaques made of apatite and amorphous calcium phosphate (ACP) deposits at the surface of the plaque in contact with urine [25]. Nevertheless, ACP formation is favoured by high CaP supersaturation and may occur in the interstitium. ACP is more important in Randall’s plaque than at its surface, advocating that ACP is the first mineral phase generated, evolving secondarily toward the more stable apatite crystalline phase [30]. Of notice, plaques are formed in the interstitium at physiological pH and apatite is highly carbonated, resulting in carbapatite rather than hydroxyapatite. The morphologic examination of the renal stone surface combined with Fourier transform infrared spectroscopy (FTIR) allows the identification of various crystalline phases in Randall’s plaques [31]. Although Randall’s plaque is made of carbapatite in most cases, and identified in more than 97% of plaques in our experience, other calcium phosphates such as ACP, brushite or whitlockite, and other chemical species have been identified within Randall’s plaque [31,32]. Compounds such as purines, particularly sodium urate, are not exceptional and raise questions about Randall’s plaque formation mechanisms.
Interestingly, the first layers of the stone surrounding Randall’s plaque are most often made of calcium oxalate monohydrate [32,33]. Calcium oxalate dihydrate and other crystalline phases may be present in Randall’s plaque related stones but not in direct contact with the plaque. The mechanisms underlying the specific affinity of calcium oxalate monohydrate for the plaque remain unknown (Fig. 3).
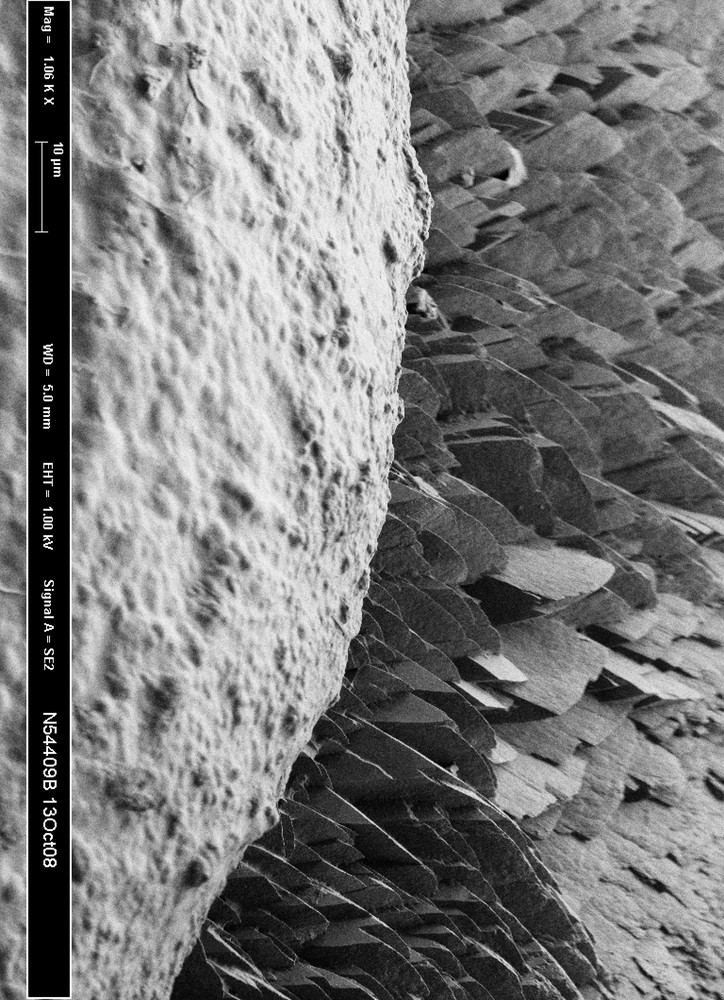
Scanning electron microscopy of the interface between Randall’s plaque made of apatite (foreground) and stone made of monohydrate calcium oxalate (background).
3.4 Does Randall’s plaque induce renal lesions and inflammatory processes?
Kim et al. have reported than stone episodes were proportional to the coverage of papilla by Randall’s plaques, suggesting a high risk of recurrence in these patients [34]. However, whether patients with Randall’s plaque have a more severe renal prognosis than other renal stone formers is unknown. Although the observation of massive plaques during reno-ureteroscopies is impressive, the evolution toward renal failure seems unlikely in most of the cases but data are missing to draw any conclusion.
The question whether Randall’s plaque may induce the inflammatory process in kidney tissues, and thereby chronic lesions and fibrosis, is a matter of debate [35]. Further studies based upon human papillary biopsies are required. Several lines of evidence suggest that Randall’s plaque may be associated with inflammation. The possible relationship between trace elements and the formation of the plaque has been obtained through μ-X-ray fluorescence and μ-X-ray diffraction [36]. These techniques allowed the identification of dramatic amounts of zinc in carbapatite of plaques in comparison to carbapatite in kidney stones. Zinc could be in such a context a biomarker of inflammation. In addition, we observed the presence of macrophages around some plaques but not in all kidney papillary biopsies (unpublished data). Finally, the presence of urate in some plaques could promote the NLRP3 inflammasome and activate innate immunity [37,38]. The relationships between plaque composition and inflammatory processes deserve further studies.
4 Conclusion
Calcium oxalate stone incidence has increased worldwide during the past decades and the observation that a large, and probably growing, number of these stones are related to Randall’s plaque is a warning signal for the medical community. The environmental determinants and the pathophysiological mechanisms responsible for Randall’s plaque formation remain elusive. There is therefore a need for further clinical studies in patients affected by Randall’s plaque related stones and for new studies based upon histopathology. The application of FTIR, μ-X-ray fluorescence and μ-X-ray to Randall’s plaque and kidney tissues allowing in-depth characterization of these plaques is a first step toward the identification of the underlying process at work. Investigating more in detail the interaction between cells and Randall’s plaque is another way to understand the mechanisms involved in such a lithogenic process, the occurrence of which has dramatically increased over the past decades in most of the industrialized countries.