1 Introduction
One of the main challenges for urology in the 21st century is to understand the biochemical mechanisms involved in the formation of Randall's plaque (RP) [1–4]. RP is a white deposit commonly made of calcium phosphate visible at the tip of papilla beneath the papillary epithelium. It was first reported by Alexander Randall in 1936, and it is considered today as a major factor for nucleation of calcium oxalate stones. From an epidemiologic point of view, a progression in the presence of RP at the tip of the papilla has been recently reported in industrialized countries [5–7]. More precisely, in US up to 80% of calcium stone formers present Randall's plaque at the tip of their papillae [8]. In France, more than 50% of stone formers exhibit Randall's plaque [9]. Stone formation is proportional to papillary surface coverage by RP, which may explain the significant increase of kidney stone prevalence observed over the past two decades in industrialized countries [10–14].
In the recent model proposed by A.P. Evan et al. [15,16], apatite plaque begins in the basement membrane of the thin loops of Henle, and spreads from there into the papillary interstitium. The starting point of our investigation is given by the observation that, if calcium phosphate apatite (CA) is the major component of most RP, other chemical phases can be found, such as amorphous carbonated calcium phosphate (ACCP) [17], whitlockite (WK), brushite, uric acid and sodium hydrogen urate monohydrate (NaUr) [18]. Even if NaUr is present in only 3.5% of RP [19], it represents a high number of patients, about 70000 subjects in France. Indeed, the occurrence of NaUr in urinary calculi is around 0.7%, i.e. 5 times less than in RP. Such chemical diversity of RP could indicate that RP begins not only in the basement membrane of the thin loops of Henle but in other parts of the kidney.
The aim of this study which follows our previous ones dedicated to RP [5,17,20,21] is to seek and discuss the presence of NaUr crystals in RP at the surface of kidney stones as well as in kidney tissues. Investigation regarding such pathological calcifications [22–25] calls for a set of in-lab techniques [26–39] or ones related to large scale instruments [40–49] capable of multi scale description [50,51]. Observations of the topology at the micrometer scale of RP present at the surface of calculi have been performed through an Environmental Scanning Electron Microscopy (ESEM) equipped with an energy-dispersive X-ray spectrometer (EDS) [52–54]. The chemical composition of such biological entities was obtained through classical Fourier Transform Infrared (FT-IR) spectroscopy [55]. Moreover, in order to obtain the spatial distribution of NaUr in kidney tissue slices, Synchrotron Radiation μFT-IR (SR-FTIR) data have been also collected [56–61].
2 Materials and methods
The biological samples including kidney tissue slices and kidney stones used in the present investigation came from Tenon hospital. As a referal center for stone analysis, the urolithiasis laboratory of AP-HP has had the opportunity to perform a morphoconstitutional analysis of more than 70,000 urinary stones over the past three decades coming from more than fifty hospitals in France. Such an analytical procedure based on the structure and the chemistry is of primary importance [62–64] and was previously described [65,66]. Because a number of stones are now removed from the urinary tract by various fragmentation techniques including ESWL, percutaneous nephrolithotomy or ureteroscopy, we selected only unfragmented stones, i.e. a cohort of 35,323 calculi.
Regarding ectopic calcifications, healthy parts of six kidneys removed by nephrectomy for cancer were used for seeking the presence of crystal deposits in the papillae.
All specimens were snap frozen and fixed with acetone. For light microscopy analysis, sections were stained with hematoxyline. For ESEM and SR-μFTIR analysis, four micron slices of the papillae’s tip were deposited on low-e microscope slides (MirrIR, Kevley Technologies, Tienta Sciences, Indianapolis). Consent was obtained from Tenon hospital urology team according to the French legislation and samples were immediately anonymized. No data about patients were collected.
An FEI/Philips XL40 Environmental Scanning Electron Microscopy (ESEM) equipped with an energy-dispersive X-ray spectrometer (EDS) was used for a precise description of the sample topology [22–25]. An important feature of the ESEM compared to a conventional SEM is the fact that non-conductive materials can be imaged without any conductive coating, which permits a direct observation with no damage to the sample. Imaging was performed with a gaseous secondary electron detector, an accelerating voltage of 20 kV and a water pressure of 0.4 torr in the chamber. This low pressure was used to maintain a high spatial resolution for the X-ray analysis by minimizing the scattering of the primary electron beam. Also, a Zeiss SUPRA55-VP type scanning electron microscope was used for microstructure observation. This field effect gun microscope operates at 0.5–30 kV. High resolution observations were obtained using two secondary electron detectors: an in lens SE detector and an Everhart-Thornley SE detector.
SR-μFTIR experiments were performed at the infrared beamline SMIS of the Soleil-Synchrotron (St Aubin-Gif sur Yvette) operating in top-up mode at 300 mA [67]. The IR spectra were collected in reflection mode using an infrared microscope (Continuum-Thermo Electron Corporation) coupled to an FTIR spectrometer (Thermo Nicolet Nicplan IR microscope 32X/NA0.6 objective). The IR microscope is equipped with a motorized sample stage (precision 0.5 μm) and a liquid nitrogen cooled mercury cadmium telluride (MCT) detector (50 μm size). Each spectrum was acquired after 100 accumulations (1 mn) at 4 cm−1 spectral resolution in the range of 650–4000 cm−1. The spatial resolution was 11 × 11 μm2. Data acquisition and processing were performed using Omnic software (Version 7.3, Thermo Electron Corporation).
3 Results
3.1 Epidemiologic investigation
From an epidemiologic point of view, among 35,323 unfragmented calculi referred to our stone laboratory over the past three decades, morphologic examination coupled with FTIR identified 10,462 (29.6%) umbilicated calculi initiated from a papillary deposit, namely a RP. Most of these stones (89.5%) were made of calcium oxalate monohydrate (COM) either pure or admixed with calcium oxalate dihydrate. Regarding the chemical composition of RP, we have already pointed out its chemical diversity [5,17,19–21]. Among 10,462 RP analyses, we found CA in 95.6%, ACCP in 7.2%, NaUr in 3.4%, and WK in 1% of cases. Other compounds were also identified such as brushite, bobierrite, opale, uric acid and some rare urates, overall representing less than 0.35% of cases. Of note, 16.9% of RP found in the stones appeared as mixtures of two or more crystalline phases irrespective of calcium oxalate. Of note, even if NaUr is present in 3.4% of the RP, it corresponds to a large number of patients. In 28% of cases, NaUr was the only crystalline phase identified in RP. In the other cases, it was mixed with either CA or ACCP. The presence of NaUr in RP appeared to be sex dependent since NaUr-containing RP was found in 4.3% of stones from male patients and in only 0.6% of cases in female patients (p < 0.00001).
3.2 Description of RP on kidney stones at the micrometer scale through ESEM and FTIR
The different RP containing NaUr displays a quite similar structure at the micrometer scale (Fig. 1a). On the ESEM photograph of a typical RP containing NaUr (Fig. 1b), a core (Area 1 in Fig. 1b) surrounded by needles (Area 2 in Fig. 1b) is observed. Such structural description is completed by FTIR spectroscopy in order to identify its chemical composition. The IR absorption band at 1037 cm−1 indicates that the core (Area 1 on Fig. 1b) is made of CA (Fig. 2a), while the needles (Area 2 on Fig. 1b) are composed of NaUr (its specific bands are positioned at 3600 and 1004 cm−1 on Fig. 2b). As shown in Fig. 2c, the stone layers surrounding RP (Area 3 in Fig. 1b) are made of whewellite.
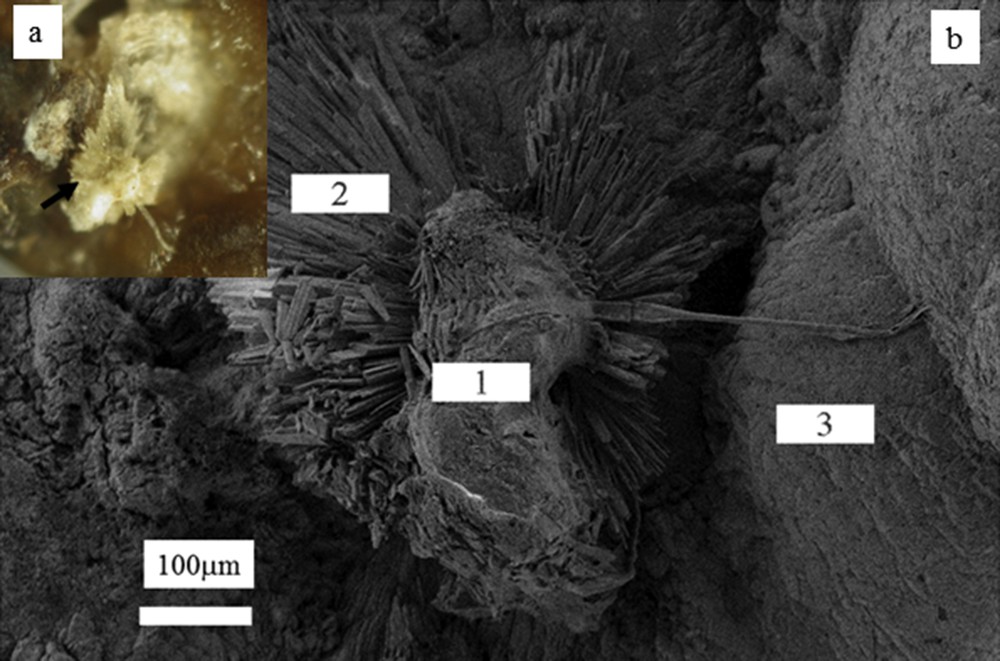
(a) Microscopic aspect of an RP made of a mixing of NaUr needles and carbapatite (black arrow) (b) RP (areas 1 and 2) attached on a calculus made of whewellite (area 3). NaUr needles of large dimension are visible on area 2.
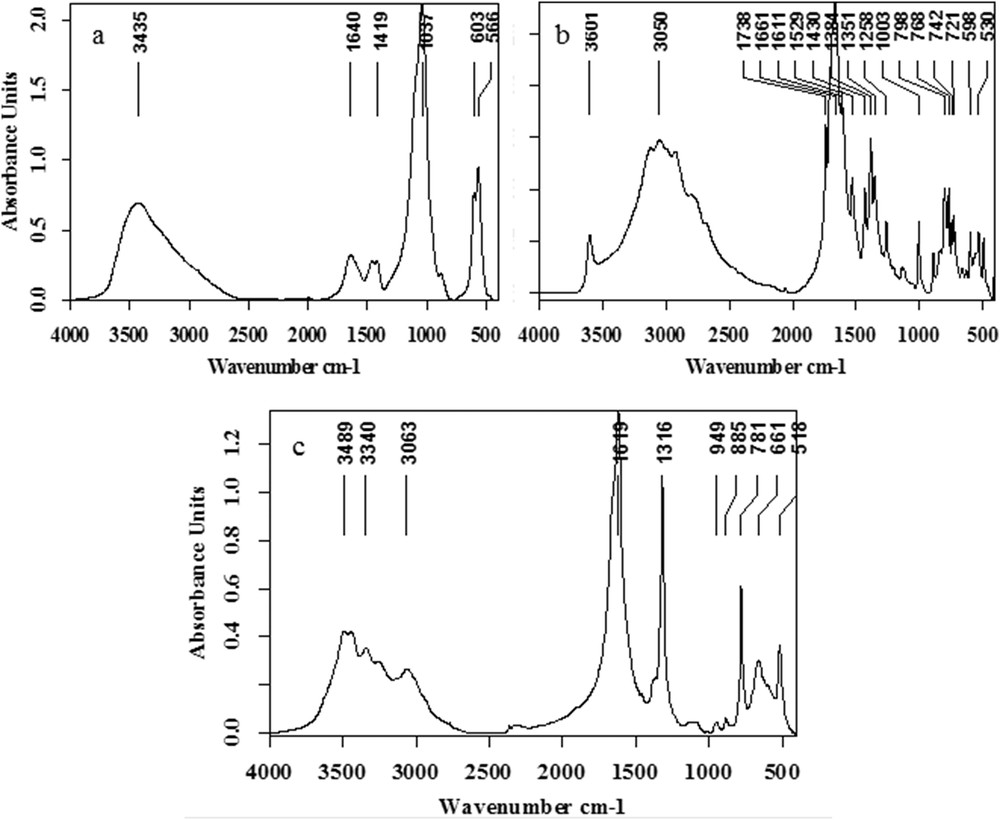
Infrared spectra of different parts of RP and of the first layers of the stone around RP: (a) calcium phosphate apatite (b) NaUr (c) whewellite.
At higher magnification, we can see through ESEM that the central part in Fig. 1b (area 1) is made of an intimate mixing of CA spherules [68–72] and NaUr acicular entities with the presence of small spherical entities at the extremity of the needles (Fig. 3a). Complementary information is given by EDS measurements regarding the elementary composition of these spherical and needle entities (Fig. 3b). EDS spectra indicate that spherical entities are made of Ca, P and O while needles are made of Na, C, O and N.
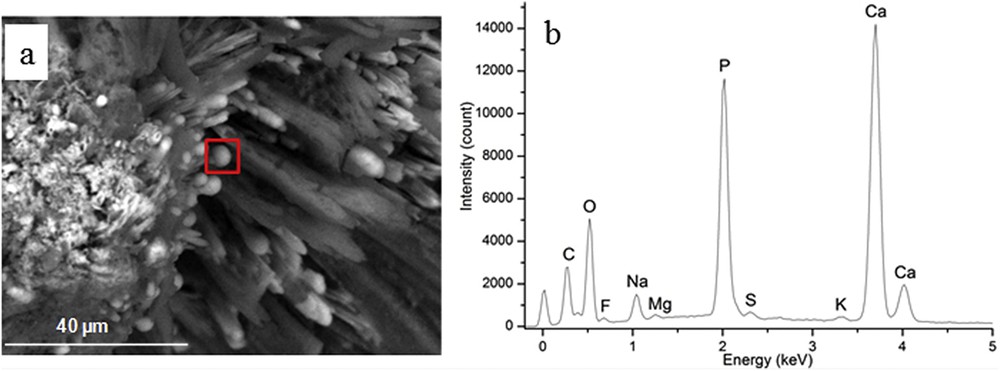
(a) At the extremity of some NaUr needles, white spherical entities are visible (b) Elementary composition through EDS of the white spherical entities show that these entities are made of Ca, P and O.
3.3 Shedding light on the presence of NaUr in kidney tissue
Regarding kidney tissue, we perform this investigation through polarizing microscopy, ESEM at the mesoscopic scale and SR-μFTIR analysis on the same samples. Six randomly selected kidneys were examined.
Among the papillae analyzed by SR-μFTIR, several from three different kidneys (50%) presented with incipient RP at the tip of the papillae. Polarized light microscopic examination of tissues revealed two types of tissular crystal aggregates in two of the six kidneys: unorganized and unpolarizing aggregates, closely associated with needle-shaped polarizing crystals (Fig. 4) while in one third kidney, only unorganized non-polarized aggregates were found. SR-μFTIR analysis of these incipient plaques revealed a mixed composition of NaUr (needle-shaped polarizing crystals) and CA (large non-polarized aggregates, Fig. 5a).
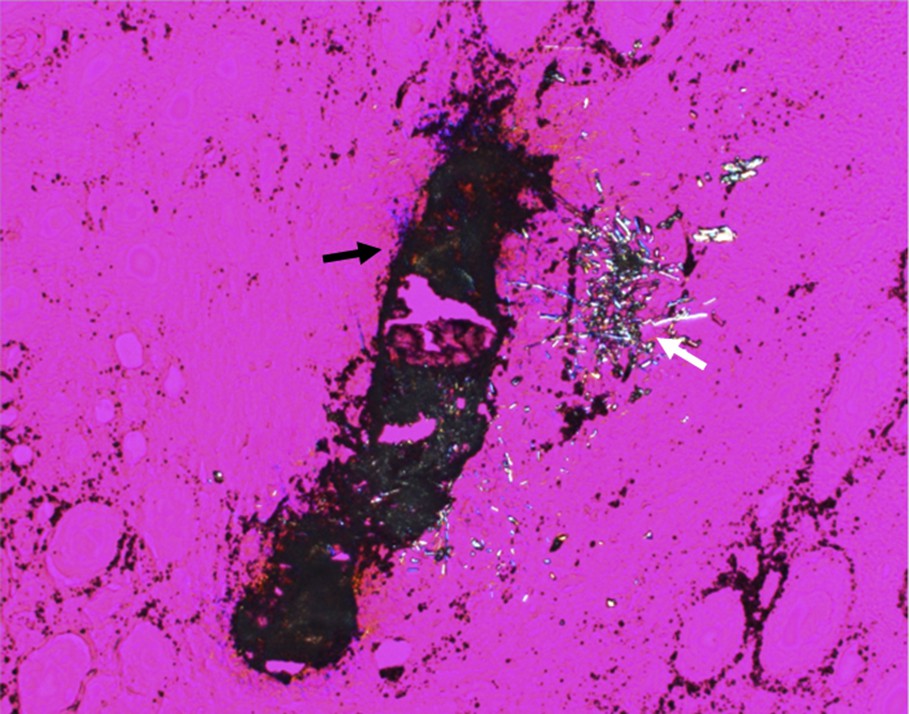
Crystal deposit observed by polarizing microscopy within the papilla of a kidney. Two types of crystals may be observed: CA (black arrow) and NaUr (white arrow).
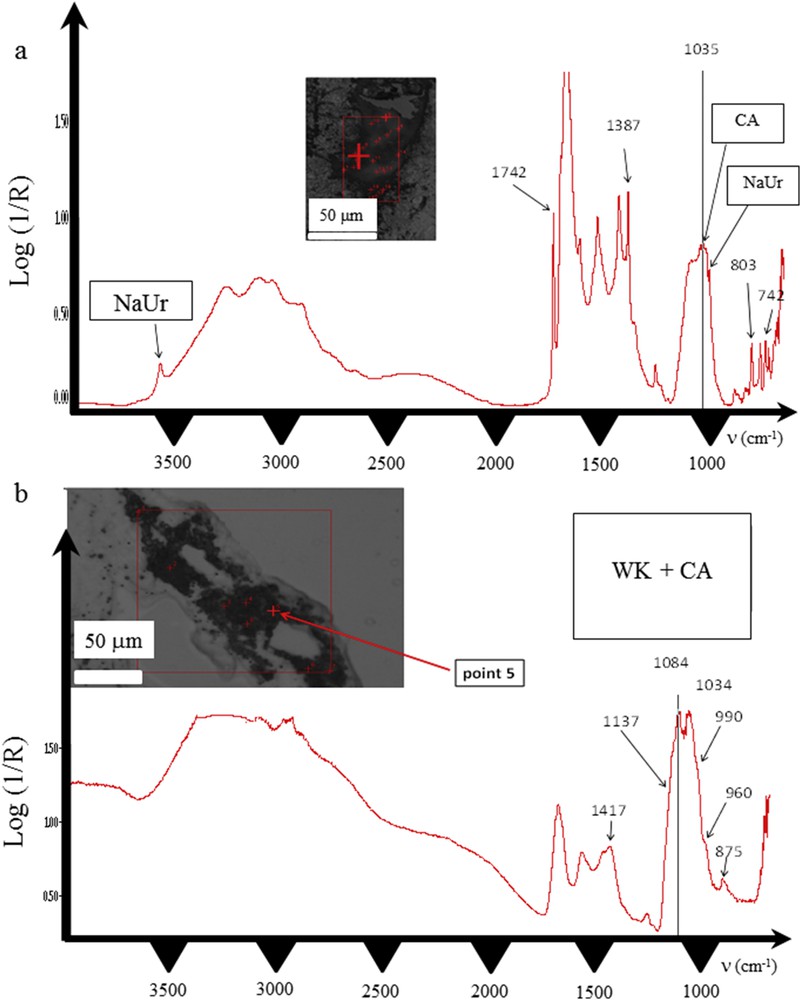
Infrared spectra of two different parts of the crystal deposits found in the papilla of the same kidney (a) spectrum of CA mixed with NaUr (b) spectrum of whitlockite mixed with CA.
S.R.-μFTIR analysis proved that needles were made of NaUr and spherules were made of CA. In some parts of the deposits, the two chemical species were mixed as shown in Fig. 5a. While CA deposit resembles a plug within a renal tubule, needle-shaped crystals of NaUr seem to be located both in the tubule and in the interstitium (Fig. 4). In addition, in another part of the same kidney sample, deposits within the papilla were located around the tubule. Infrared spectra evidenced the presence of whitlockite, another form of calcium phosphate containing Mg ions. Whitlockite was mixed with CA (Fig. 5b). NaUr crystal aggregates were sometimes surrounded by numerous cells as observed in Fig. 6, thus suggesting an inflammatory process not observed in other parts of the tissue where only CA or CA + WK mixture was found. ESEM photographs also confirm the close association between NaUr needles and CA spherules (Fig. 7).
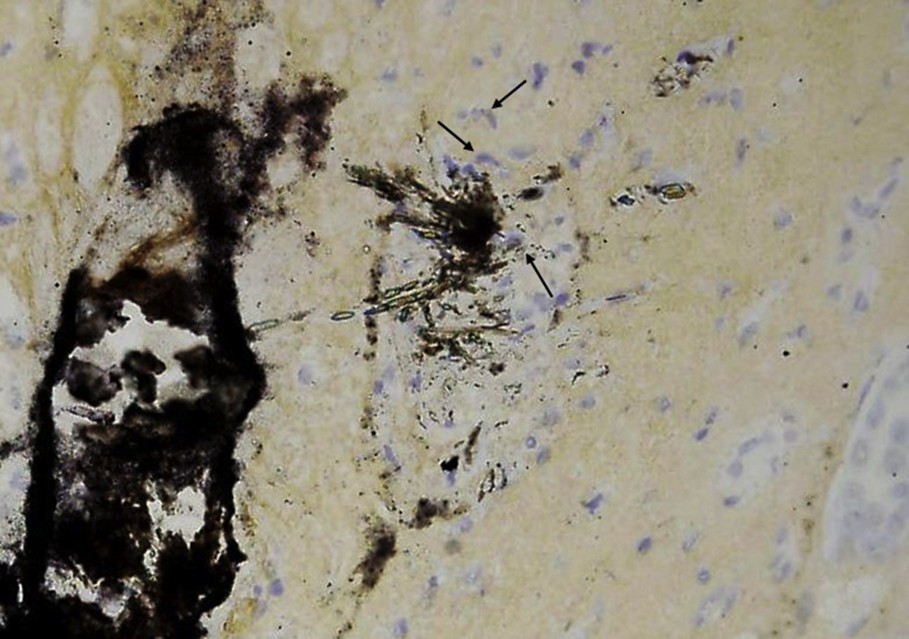
Magnification of the crystal deposit seen in Fig. 4. Note the presence of numerous cells (arrows) around the needle-shaped crystals made of NaUr monohydrate.
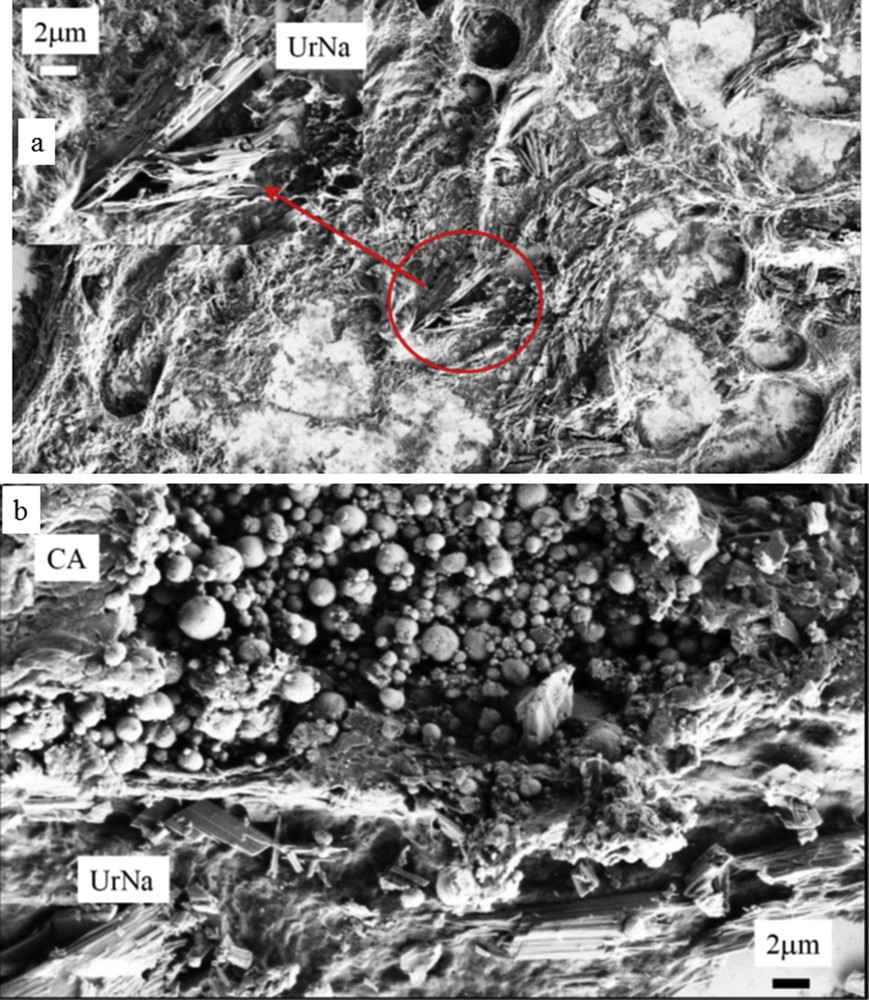
Observations through ESEM of a kidney slice show the presence of both NaUr needles and apatite spherical entities.
4 Discussion
From a medical point of view, ectopic calcification defined as inappropriate biomineralization occurring in soft tissue (which can be related to severe pathologies like breast or testicular cancer) and concretion defined as the deposition of crystalline materials in excretory ducts (KS, also gallstones, salivary stones and so on.) constitute two very different families of pathological calcifications [22]. Our study is dedicated to the growing process of a concretion on an ectopic calcification.
The fact that several epidemiologic studies point out Randall's plaque as a major public health problem have induced a number of investigations in order to understand precisely the biomechanims responsible for the formation of these ectopic calcifications [73–101]. Recently, S.R. Khan et al. have reported that RP starts as small, discrete spherical entities, perhaps as a result of mineral imbalance, but it grows by the addition of more crystals through the mineralization of membranous vesicles and collagen fibers [102]. Other authors have considered genetically engineered mice lacking Tamm–Horsfall protein as a possible animal model of Randall's plaque [103]. Their data show that the location, ultra-structure, chemical composition and lack of associated inflammation of the interstitial crystals in THP-null mice strongly resemble those of RP found in humans. Other authors advance the hypothesis that RP constitutes a truly ectopic biomineralization in the renal tissue, and that Henle's loop epithelial cells, or pericyte-like interstitial cells, or papillary stem cells differentiating along a bone lineage might be involved [104].
All these studies discuss only CA RP. The starting point of our investigation was based on the observation that different chemical phases have been identified in RP opening a discussion of the initial model in which only CA based RP have been identified [15,16]. First, observations at the micrometer scale through ESEM on a typical RP containing NaUr show clearly that large needles of NaUr are present around a central part made of an intimate mixing of CA spherules and NaUr needles. Such spatial description is far from the one we have observed previously for apatite RP [8,15,16]. As previously reported, it seems that apatite RP is the result of an agglomeration of empty and apatite filled cylindric channels which correspond both to calcified Bellini’s ducts and vessels and to collecting ducts filled by CA plugs [8,15,16]. Thus RP at the surface of the papilla epithelium could be the consequence of an agglomeration of CA spherules from both interstitium and tubular lumen as suggested by the very highly variable carbonation rate of CA [5]. These ESEM observations indicate clearly that different biochemical mechanisms are involved in the pathogenesis of RP. The simultaneous presence of CA and NaUr in 3.4% of RP suggests the involvement of urate salt in a number of patients, i.e. more than 70,000 subjects in France based on epidemiological data regarding the prevalence of kidney stones in the general population. Moreover, among the six kidney tissue samples analyzed for identifying possible ectopic crystals, we found NaUr in two cases, i.e. one third of the kidneys, which is a very high occurrence.
To address more deeply the presence of NaUr in RP, SR-FTIR spectroscopy, a technique which combines IR spectroscopy and microscopy for determining the distribution of molecular groups at the cell scale has been used [71,105–114]. Thanks to 2D imaging and the high signal to noise ratio, different publications have shown that such an approach is particularly suitable for tissues which are usually complex and heterogeneous [105–118]. Regarding the different kidney slices that we have considered in this investigation, SR-μFTIR experiments point out the presence of NaUr in the renal papilla as well as an intimate mixing of CA and NaUr. From a physicochemical point of view, NaUr and apatite may have epitaxial relationships. In 1976, Pak reported that NaUr was able to serve as a heterogeneous nucleator for hydroxyapatite and conversely [119].
Several studies have considered the relationship between NaUr and calcium oxalate stones. Some authors underline that particulate monosodium urate is likely to influence calcium oxalate stone formation by binding to and attenuating the potency of urinary inhibitors [120].
However, our results highlight that NaUr may also segregate with CA at the tip of renal papillae. Our data deserve further studies to identify whether urate crystals originate from tubular structures or grow within interstitium. The close association of urate and apatite in tissues, as well as in established plaques, is striking: these two crystalline phases usually do not coexist in renal stones. Moreover, the identification of urate in at least some incipient Randall's plaques may impact surrounding tissues. Actually, NaUr crystals have been identified a few years ago as a powerful danger signal, triggering NLRP3 inflammasome-dependent and independent pathways to generate interleukin-1 beta synthesis and innate immune cell recruitment [121]. Our observation of cells around NaUr crystal aggregates may advocate this pro-inflammatory role. This observation may be a clue to understand the discrepancy in the literature about the potential link between Randall's plaque and inflammation [122,123]. One may hypothesize that the inconstant presence of urate salts within plaque is the trigger of inflammatory cell recruitment. Our data are too sparse to draw any definitive conclusion on this topic but we did not identify any immune cell infiltrate surrounding the CA incipient Randall's plaque devoid of urate salts. Work is in progress to identify the cells observed around NaUr crystals.
5 Conclusion
The complete set of data shows that Randall's plaques are not always made of apatite, but may also contain other phosphate salts and oxypurines such as NaUr that modify significantly their morphology at the micrometer scale. Such a finding suggests that several pathways are involved in the RP formation. The latter phase is present in kidney tissue quite close to apatite, an experimental fact which has not been included in the biochemical mechanisms involved in the pathogenesis of Randall's plaque. As Coe et al. said, “No single theory of pathogenesis can properly account for all human kidney stones.” It seems that this sentence is also true for Randall's plaque [123]. Further investigations are needed to establish a significant relationship between such chemical and structural data and the clinical data of the patients.
Acknowledgments
This work was supported by the Physics and Chemistry Institutes of Centre national de la recherche scientifique and by contracts ANR-09-BLAN-0120-02, ANR-12-BS08-0022, ANR-13-JSV-10010-01, convergence UPMC CVG1205 and CORDDIM-2013-COD130042.