1 Introduction
Pharmaceuticals and personal care products (PPCPs), in many cases, are not significantly degraded/removed in wastewater treatment plants [1,2]. They are introduced into surface waters with the effluents of wastewater treatment plants. Some of them have adverse effects on surface water ecosystems [3]. Thus, it is important to explore, develop and improve technologies for the removal of such compounds to ensure a sustainable healthy natural environment [4–8]. Among all the existing approaches for the removal of pollutants from contaminated water, the processes of flocculation and coagulation, biological treatments, oxidation, reverse osmosis, ion exchange, electrodialysis and adsorption are currently the most popular.
Some advanced technologies have been evaluated with regard to their ability to decrease the xenobiotic discharge into water bodies, e.g., oxidative processes, activated carbon or membrane filtration. Generally, the high cost of these methods is the main disadvantage that limits their usage on a large scale. There is a need to find efficient, selective, inexpensive and eco-friendly low-cost adsorbents for water treatment. Inorganic chemical adsorbents, like clay minerals are low-cost adsorbents, are widely used to remove inorganic and organic pollutants from aqueous solutions. They have thus been studied widely, with the aim of providing an alternative to activated carbon in the treatment of surface and ground water and industrial effluents [9–13].
Removal by adsorption is generally considered to be an effective and economic purification method for lowering the concentrations of organic molecules in water and wastewater treatment [9]. Different adsorbents were identified for this purpose. Activated carbon, due to its high surface area, porous structure and special surface reactivity, proved efficient for the removal of organic molecules, especially hydrophobic compounds [14–16]. However, its relatively high cost and difficult regeneration processes have to be faced with. Activated carbon prepared from agricultural by-products (biochar) is being investigated [10,17] for this purpose. One alternative to activated carbon focuses around natural and modified clays that have been considered for the removal of organic compounds from water systems [13]. However, published information on the removal of PPCPs by adsorption onto mineral and carbon adsorbents is limited [11,12,18–20].
The objective of this study was to evaluate the sorption capacity of several natural and modified clays for the adsorption of six pharmaceuticals and personal care products. This work is a prelude to comprehensive research towards searching for an effective way to remove these types of contaminants from municipal wastewaters. The adsorbates were selected to be representative of a wide range of contaminants because of their physical properties and reported occurrence in surface waters [2,3,21,22]. Various organic and inorganic modification types of clays were tested. Additionally, under different pH conditions, both pharmaceuticals and clay materials undergo protonation/deprotonation reactions.
2 Experimental
2.1 Materials
The compounds included in this study were ketoprofen, ibuprofen, diclofenac, carbamazepine, bisphenol A, triclosan, and 1-hydroxypyrene as internal standards with purities of 98% and higher, all supplied by Sigma-Aldrich (Saint Louis, USA). The molecular structure, physical, and chemical properties of the compounds are summarized in Table 1. Solutions of the compounds, both singly and as mixtures, were prepared in methanol. A stock standard solution of 1000 μg/mL of each compound was used to prepare a standard mixture solution. Mixtures were used to spike water samples. Analytical grade reagents CaCl2, KH2PO4, NaOH, HCl, and acetonitrile (HPLC super gradient grade), methanol (HPLC grade) and ethyl acetate (HPLC grade) were obtained from POCH (Gliwice, Poland). Deionized water (<0.07 S/cm) was used to prepare sample solvents for the HPLC system and the solid-phase extraction (SPE) was obtained from the HLP5 pure water system (Hydrolab, Gdansk, Poland). Oasis HLB extraction cartridges, (3 cc/60 mg) for SPE were purchased from Waters (Wexford, Ireland). pH measurements were carried out using a multimeter from Crison Instruments (Barcelona, Spain).
Molecular structure and characteristics of the compounds.
Compounds | Molecular structure | Molecular weight, g/mol | pKa | Log KOW | Water solubilitya mg/L |
Carbamazepine (CBZ) | 236.27 | 13.90 | 2.45 | 17.7 | |
Diclofenac (DCF) | 296.16 | 4.15 | 4.51 | 2.37 | |
Ibuprofen (IBP) | 206.28 | 4.91 | 3.97 | 21 | |
Ketoprofen (KTP) | 254.28 | 4.45 | 3.12 | 51 | |
BisphenolA (BPA) | 228.29 | 9.59 | 2.20 | 120 | |
Triclosan (TCS) | 289.50 | 8.14 | 4.76 | 10 |
2.2 Sorbents characterization
Both commercial and modified layered clays were studied. The starting clays were montmorillonite (Mt) (Riedel-de Haën, Germany), vermiculite (VER) (Sigma-Aldrich, Steinheim, Germany), bentonite (B) (Sigma-Aldrich, Steinheim, Germany), kaolinite (K) (Merck, Darmstadt, Germany), commercial acid activated montmorillonites K10 and K30 (K10 and K30) (Sigma-Aldrich, Steinheim, Germany). The treatment of montmorillonite with mineral acids led to a notable increase in specific surface area, depending on the concentration of the acid and the duration of the activation process. According to Flessner et al. [23], acidic modifications change the density and type of acidic sites. K10 possesses a larger number of acidic sites per unit surface area of adsorbent than K30 [23]. K30 has the highest density of relatively strong Brønsted acid sites, but a very low content of Lewis acid ones, which is attributed to the extensive loss of Al.
Selected clays were modified according to the procedures shown in Scheme 1. This preparation gave carbonaceous-mineral nanocomposites. The initial material was commercial bentonite or montmorillonite. An optional step during this preparation was intercalation with aluminum polycations.
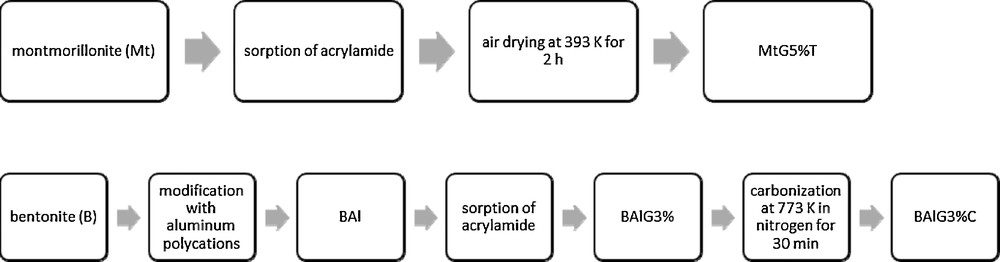
Synthesis of the organic modified clay minerals.
Intercalation was carried out with aluminum polycations (designation “Al”) with chlorhydrol according to the procedure described by Vaughan and Lussier [24].
The formula of polyacrylamide – commercially named Gigtar and used as a precursor of carbonaceous deposits – is presented in Table 2. The polymer was absorbed from aqueous 3 or 5% solutions for 24 h. Surface areas SBET and pore volumes were determined by nitrogen sorption at 77 K using the volumetric method (Micromeritics ASAP 2010, Norcross, USA). Prior to sorption, the residual gasses were removed at 200 °C for 16 h. The natural pH of the tested sorbents was measured in a 0.01 M CaCl2 solution, in which sorption experiments were conducted. Fractions of 0.500 mm of vermiculite and organic modified clay minerals and, in the case of other sorbents, fractions below 0.063 mm, were used in the experiments. Table 2 summarizes the specific surface area SBET and the natural pH of the studied sorbents.
Properties of sorbents used in the study and formula of polyacrylamide used as a precursor of carbonaceous deposits in organic modified clay minerals.
Sorbents | BET Surface area m2/g | pH (0.01 M CaCl2) | |
Unmodified clay minerals | Bentonite (B) | 37 | 8.4 |
Kaolinite (K) | 20 | 6.0 | |
Montmorillonite (Mt) | 40 | 8.4 | |
Vermiculite (VER) | 6 | 6.5 | |
Acidic modified clay minerals | Montmorillonite K10 (K10) | 269 | 3.9 |
Montmorillonite K30 (K30) | 330 | 3.6 | |
Organic modified clay minerals The formula of polyacrylamide | Nanocomposite (MtG5%T) | 37 | 6.8 |
Nanocomposite (BAlG3%C) | 90 | 5.4 |
Clays prepared in this manner can interact with the pharmaceuticals in several ways:
- • direct ionic interactions from, e.g., a negative surface charge of the clay to a positive (partial) charge in the organic molecule or vice versa;
- • hydrogen bonds from a negative surface charge to a negative (partial) charge in the molecule;
- • van der Waals interactions (organically modified clays);
- • calcium bridges similar to hydrogen bridges, but based on Ca.
2.3 Sorption experiments
Sorption experiments were performed according to OECD Guideline 106 (2000). Batch sorption experiments of selected compounds were conducted in 40-mL vials containing 200 mg of clay minerals in a 10-mL solution of 0.01 M CaCl2 to keep ionic strength constant. The procedure included two steps:
- • preconditioning of the clay/water suspension for 24 h with a background solution of 0.01 M CaCl2 including appropriate additions of NaOH or HCl (0.1 M solutions) for pH adjustment;
- • after preconditioning, the suspensions were spiked with the appropriate amounts of a multistandard solution to reach a concentration of 100 μg/L and were shaken horizontally for 24 h in the dark at 20 °C.
After equilibrium was reached, the solutions were filtered and analyzed. pH-dependent sorption was performed with selected pharmaceuticals at 100 μg/L at pH values close to 4 and 8.
Blank and control solutions were processed in parallel with each sample. The blank and control solutions were shaken and analyzed using the same procedure as all other samples. Blank solutions were prepared by adding the same amount of respective sorbents as to the real samples, but none of the tested compounds to the 10 mL of 0.01 M CaCl2 solution. Control: To estimate the losses of tested compounds due to the sorption on glass surface during 24-h-long experiments, control samples containing 10 mL of 0.01 M CaCl2 and the mixture of standard solutions at a concentration of 100 μg/L, but no clay materials, were used. The data show that the mass balances of all compounds were incomplete. After 24 h, the total mass of CBZ, TCS and BPA was reduced to approximately 80% in the aqueous solution. The total mass of DCF was 89% and for KTP it exceeded 92%. The absorbed fraction of each compound was calculated from the difference between the concentration in the aqueous phase and the corresponding control solution including appropriate recovery. All experiments were performed in duplicates; each solution was analyzed two times and the obtained values were averaged.
2.4 Analytical procedure
The supernatants obtained after filtration through a 0.2-μm glass fiber syringe were acidified to a pH of 2.0 and successively extracted by using 60-mg Oasis HLB cartridges. Chromatographic analyses were performed with a Varian HPLC system consisting of the 9012 pump, 9050 UV-Vis detector and 9100 auto-sampler. The separation was carried out using a LiChrospher® 100 RP-18 (125 mm × 4 mm; particle diameter = 5 μm) cartridge column (Merck, Darmstadt, Germany) protected by a LiChrospher® 100 RP-18 (4 mm × 4 mm; particle diameter = 5 μm) guard column (Merck). The mobile phases were:
- • 50 mM KH2PO4;
- • acetonitrile (ACN).
The HPLC method began with 10% ACN in the mobile phase and increased to 70% within 30 min. Afterwards, the column was equilibrated for 10 min using 10% ACN. The injection volume was 20 μL and the flow rate 1 mL/min. The quantification limits (LOQ) of the analytical procedure were 8 μg/L, 10 μg/L, 5 μg/L, 3 μg/L, 6 μg/L and 3 μg/L for carbamazepine, ketoprofen, bisphenol A, diclofenac, ibuprofen, and triclosan, respectively. The LOQs calculated as a signal-to-noise ratio were established by using the supernatants of control solutions. Samples for recovery calculations were prepared by adding 200 mg of the respective sorbents to 10 mL of the 0.01 M CaCl2 solution and after 24 h of preconditioning, the supernatants were spiked with the appropriate amounts of a multistandard solution to reach a concentration of 100 μg/L, and the extraction of the solid phase was conducted. The internal standards were spiked into the SPE eluate just before HPLC–UV/Vis analysis. Depending on the compound, the analytical recoveries ranged from 80% to 95% with Relative Standard Deviations (RSD) between 4.5% and 13%.
3 Results and discussion
3.1 Equilibrium partitioning of PPCPs between clay minerals and water
The results obtained in the experiments are displayed in the form of sorption constants of PPCPs onto the investigated clay minerals, after 24 h of sorption, at concentrations of 100 μg/L. The sorption constants Kd were obtained from the PPCPs concentration in the clay mineral Cs and the PPCPs concentration in the water solution, Cw, using the following equation (1):
(1) |
The resulting Kd values for PPCPs are shown in Table 3 for both neutral solutions (pH 6.0–8.4) and acidic solutions (pH 3.1–4.7). Sorption constants of PPCPs obtained for acidic solutions are different from those for neutral solutions. For neutral solutions, Kds ranged from 1.6 (ibuprofen) to 609 (triclosan). For acidic solutions, higher values of Kd were obtained and ranged from 14.3 (bisphenol A) to 696 (triclosan). Both the clay minerals and PPCPs can be protonated and deprotonated. The sorption results are thus the cross-correlation of the unknown protonation grade of the clay minerals and the protonation grade of PPCPs. While one system can easily be modelled, it is very difficult to model a two-component system. For almost all the tested clay minerals in acidic solutions, triclosan was found to have the highest Kd, followed by diclofenac. The exceptions are the Kds of ibuprofen and bisphenol A for organic modified bentonite (BAlG3%C). The highest Kd values of carbamazepine and ketoprofen were obtained for acid-modified montmorillonite (MtK30), for sorption in neutral solutions.
Sorption constants Kd [L·kg–1] values, including the measurement uncertainties as standard deviations as indicated by (±), of PPCPs between clay minerals and water under acidic and neutral pH conditions (in parentheses).
Unmodified clay minerals | Acidic modified clay minerals | Organic modified clay minerals | ||||||
B (3.8) | Mt (4.7) | K (3.1) | VER (3.1) | MtK10 (3.9) | MtK30 (3.6) | MtG5%T (3.1) | BAlG3%C (4.0) | |
CBZ | 26.5 ± 2.1 | 29.2 ± 1.1 | 26.1 ± 2.5 | 35.7 ± 2.9 | 25.5 ± 4.5 | 43.8 ± 2.2 | 33.2 ± 1.0 | 25.1 ± 2.5 |
KTP | 25.9 ± 1.9 | 37.2 ± 0.7 | 26.9 ± 2.0 | 32.2 ± 2.0 | 47.0 ± 8.2 | 57.1 ± 5.1 | 24.7 ± 0.5 | 15.5 ± 0.3 |
BPA | 24.4 ± 1.4 | 38.7 ± 2.1 | 28.1 ± 3.7 | 34.3 ± 2.1 | 14.3 ± 0.1 | 22.5 ± 1.1 | 29.0 ± 7.0 | 210.1 ± 4.8 |
DCF | 33.0 ± 0.7 | 73.5 ± 3.7 | 38.1 ± 5.1 | 62.4 ± 0.5 | 235.5 ± 7.8 | 139.9 ± 1.3 | 67.3 ± 27.8 | 76.2 ± 3.0 |
IBP | 26.0 ± 2.6 | 32.4 ± 2.4 | 23.2 ± 4.1 | 30.4 ± 0.4 | 58.1 ± 4.8 | 86.6 ± 7.8 | 24.8 ± 5.1 | 190.3 ± 15.9 |
TCS | 36.3 ± 0.4 | 235.7 ± 7.9 | 44.6 ± 4.7 | 92.9 ± 12.8 | 696.6 ± 52.4 | 106.1 ± 21.3 | 463.3 ± 8.0 | 88.2 ± 2.5 |
B (8.4) | Mt (8.4) | K (6.0) | VER (6.5) | MtK10 (8.3) | MtK30 (6.8) | MtG5%T (7.7) | BAlG3%C (7.7) | |
CBZ | 11.8 ± 0.9 | 14.0 ± 0.0 | 6.9 ± 0.2 | 34.9 ± 13.0 | 18.4 ± 0.6 | 55.1 ± 6.3 | 30.2 ± 2.5 | 20.6 ± 1.0 |
KTP | 7.5 ± 1.6 | 14.7 ± 0.3 | 6.4 ± 0.3 | 27.1 ± 4.9 | 5.8 ± 1.9 | 70.4 ± 2.7 | 32.1 ± 1.0 | 9.2 ± 2.5 |
BPA | 13.0 ± 0.7 | 18.9 ± 4.2 | 9.6 ± 2.8 | 35.4 ± 5.4 | 17.7 ± 0.4 | 18.2 ± 4.5 | 19.1 ± 4.1 | 15.1 ± 1.9 |
DCF | 5.8 ± 0.6 | 15.6 ± 0.7 | 6.6 ± 0.4 | 32.7 ± 6.8 | 9.1 ± 0.2 | 172.5 ± 7.9 | 17.9 ± 0.7 | 10.2 ± 0.2 |
IBP | 1.6 ± 0.2 | 6.3 ± 3.0 | 17.7 ± 1.1 | 32.5 ± 4.5 | 24.0 ± 1.2 | 101.3 ± 5.4 | 15.6 ± 2.5 | 37.7 ± 1.9 |
TCS | 21.5 ± 2.3 | 57.7 ± 2.4 | 19.9 ± 0.9 | 57.8 ± 3.1 | 609.1 ± 49.3 | 121.8 ± 12.5 | 467.7 ± 22.6 | 71.7 ± 3.9 |
3.2 Effect of pH on sorption
Fig. 1 shows the sorption of all tested compounds expressed as sorption percentages of PPCPs to sorbents depending on pH. The sorption of PPCPs onto bentonite (B), montmorillonite (Mt) and kaolinite (K) was strongly dependent on pH and was higher in acidic solutions. In the case of vermiculite (VER), the sorption of PPCPs was not significantly affected by pH. The acid-modified montmorillonite K30 exhibited high sorption of PPCPs independently of the pH solutions. Sorption of PPCPs onto montmorillonite K10 strongly depends on the solution's pH and was higher in the acidic solution.
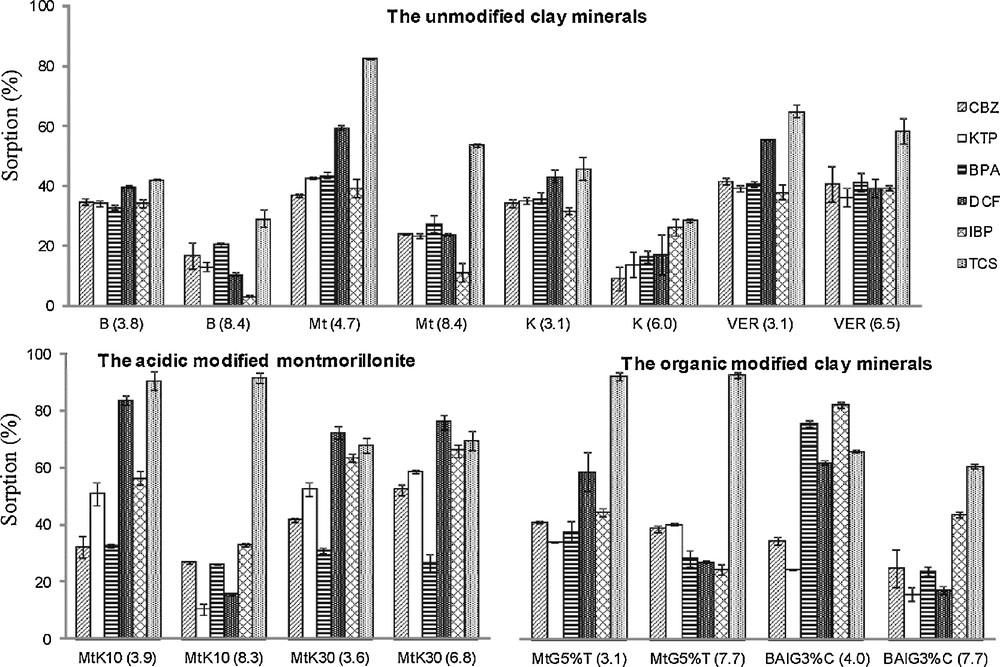
Sorption of carbamazepine (CBZ), ketoprofen (KTP), bisphenol A (BPA), diclofenac (DCF), ibuprofen (IBP) and triclosan (TCS) onto modified and unmodified clay minerals as a function of pH (in parentheses): bentonite (B), kaolinite (K), montmorillonite (Mt), vermiculite (VER), acid-modified montmorillonites (K10), (K30), nanocomposites: (MtG5%T) and (BAlG3%C).
Considering organically modified clays, the sorption of PPCPs depends on the pH of solutions, strongly for sorption of PPCPs to BAlG3%C, less for sorption onto MtG5%T. The highest sorption was observed for bisphenol A, diclofenac, ibuprofen and triclosan onto a carbonaceous sorbent (BAlG3%C), under acidic pH. Montmorillonite modified with a polymer (MtG5%T) was more efficient for the removal of carbamazepine, ketoprofen and triclosan, in spite of a lower specific surface area in comparison to BAlG3%C. Sorption of diclofenac was on the same level at acidic pHs for both organic modified sorbents (Fig. 1).
Generally, the increase in sorption with decreasing pH can be explained in relation to the speciation of the tested PPCPs. Sorption of both neutral and anionic species to clay minerals increases with their hydrophobicity expressed as log KOW [25,26]. Note that the hydrophobicity of PPCPs follows the order: triclosan > diclofenac > ibuprofen > ketoprofen > carbamazepine > bisphenol A (see Table 1). The results of sorption constants of tested PPCPs reveal that triclosan and diclofenac were absorbed to clay minerals to a significantly higher extent than bisphenol A. Moreover, sorption of acidic pharmaceuticals such as diclofenac, ibuprofen and ketoprofen in solutions at neutral pH significantly decreased due to the presence of ionic forms of compounds. According to Bui et al. [11], the sorption of carbamazepine and acidic pharmaceuticals (diclofenac, ketoprofen and ibuprofen) on silica characterized by a negatively charged surface was strongly pH dependent, similar to the case of minerals with negative surface polarity presented in this paper. The experimental results presented by Behera et al. [12] also showed that the sorption of triclosan onto montmorillonite and kaolinite is higher under acidic rather than alkaline conditions.
The high removal efficiencies of all compounds at acidic pHs suggest that the hydrophobic interaction may be considered the main mechanism of sorption for the tested natural and modified clay minerals. At acidic pHs, the negative net charge on the surface of the minerals is reduced, deprotonation of the functional groups of the acidic pharmaceuticals is minimized, and sorption is enhanced due to the decrease of electrostatic repulsion between them. Vermiculite has a smaller number of negatively charged sites, which could be confirmed by pH = 6.5 in 0.01 M CaCl2. As expected, sorption of all tested adsorbates to vermiculite is independent of the pH of the solution. Decreasing pH to 3 for vermiculite increased the sorption of diclofenac only from 40% to 55%, which is probably connected with the presence of diclofenac in its non-deprotonated form at pH < pKa.
3.3 Influence of organic modification of minerals towards sorption
Montmorillonite was modified with polymer and air dried at 393 K for 2 h (MtG5%T), while the organic phase on bentonite was subjected to carbonization at 773 K in a nitrogen atmosphere for 30 min (BAlG3%C). Although the specific surface area in organic-rich sorbents is decreased, the modification of the surface properties may explain the improvement in sorption with respect to some compounds [27–29]. This is probably connected with favorable conditions in the net charge on the surface of the sorbent and with interactions with anionic forms of acidic pharmaceuticals. Wen-Tien et al. [18] pointed out that a carbon adsorbent with a low surface polarity was more effective than a mineral adsorbent. The highest sorption onto MtG5%T regardless of the pH of solutions was observed for triclosan (89%). Sorption of carbamazepine (∼40%) and ketoprofen (∼30%) onto MtG5%T was higher than to BAlG3%C and slightly dependent on the pH. This trend of sorption onto MtG5%T could be correlated with non-electrostatic interactions. On the other hand, sorption of bisphenol A, diclofenac and ibuprofen was higher onto BAlG3%C and depends on pH (see Fig. 1). Functional group deprotonation of compounds and on the sorbent's surface is strongly limited at acidic pH. One may expect that the dependence could be correlated with the ionization state (pKa values) and to the changes of electron density on the bentonite surface, particularly when metal is added (aluminum promotion). In general, the observed sorption capacities of tested PPCPs on BAlG3%C seems to be comparable to those reported in the literature for organic and inorganic intercalated bentonite [19].
3.4 Correlation between properties of sorbents and compounds
Sorption constants (Kd) [L/kg] relative to specific surface areas (A) [m2/g], (Kd/A) [L/m2] were determined to assess the influence of the size of specific surface areas or other individual, chemical differences of the respective surfaces on sorption. The resulting Kd/A values for the sorption of the tested PPCPs at neutral pH onto natural and modified sorbents are shown in Fig. 2. It could be expected that Kd/A values should be similar in those cases where sorption depends largely on the size of the specific surface area. If, however, the obtained Kd/A values vary significantly as a function of compounds, the chemical properties of the materials should be predominant for the process.
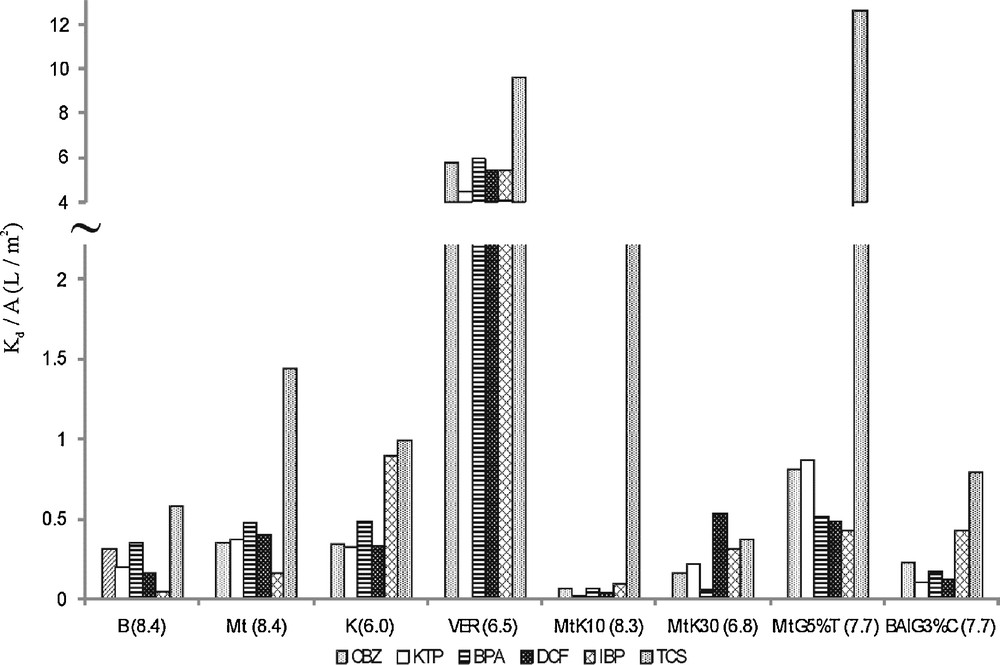
Equilibrium partition coefficients (Kd) relative to the specific surface are (A) of sorbents, Kd/A [L/m2] for carbamazepine (CBZ), ketoprofen (KTP), bisphenol A (BPA), diclofenac (DCF), ibuprofen (IBP) and triclosan (TCS): bentonite (B), kaolinite (K), montmorillonite (Mt), vermiculite (VER), acidic modified montmorillonites (K10), (K30), nanocomposites: (MtG5%T) and (BAlG3%C) – pH in parentheses.
For the studied compounds, the calculated Kd/A values were relatively high in the case of vermiculite (Fig. 2.). Vermiculite has the smallest specific surface area (6 m2/g), so it should be noticed that the individual and specific properties of the surface of vermiculite were of crucial importance for sorption. Similarly, Kd/A coefficients for kaolinite with 20 m2/g indicate that sorption could be attributed to specific surface properties. The Kd/A values for organic modified bentonite BAlG3%C (Fig. 2) showed also that specific surface properties could be relevant for sorption when considering this material. As can be seen from Fig. 2, Kd/A values for pharmaceuticals and bisphenol A, except triclosan, were significantly lower for all other sorbents. This might indicate that sorption onto these minerals depends on the size of the specific surface areas. It seems that the large specific surface areas of montmorillonites K10 and K30 were not enough to result in high sorption. Individual properties of each surface were significant.
The distinguishing Kd/A values for triclosan for most sorbents result from its hydrophobic properties, but also from the surface properties of the clays. Generally, the application of the Kd/A coefficient could be used to illustrate what kind of properties of sorbent and compound play the main role in the sorption process.
3.5 Proficiency test
Scenarios were calculated for a proficiency test by assessing how much sorbent would be needed to remove 50% of the PPCPs at equilibrium conditions, hypothesizing that partitioning would be the dominant mechanism. The amount of sorbent that is needed to remove 50% of the respective PPCP from 1 m3 of the aqueous solution is calculated considering for this model a 24-h contact of the respective amount of water with a sorbent. The resulting amounts of sorbents for this model were calculated and are shown in Table 4. Considering the worst absorbed compounds, at neutral pHs, solutions containing between 36.9 kg of vermiculite and 619.5 kg of bentonite were used to reduce the concentration of PPCPs by 50%. At acidic pHs, solutions containing between 34.2 kg of montmorillonite and 70.1 kg of montomorillonite K10 were used. The amounts of sorbents needed to reduce concentrations of PPCPs in aquatic solutions are much higher at neutral pH. The mass ratio of sorbent needed to reduce concentration of PPCPs by 50% at neutral pH and acidic pH close to 1 was obtained for vermiculite, montomorillonite K30, and organic modified montmorillonite MtG5%T. The mass ratios only for diclofenac are 1.9 and 3.8 for sorption onto vermiculite and MtG5%T, respectively. However, the lowest cost of vermiculite makes it one of the most promising adsorbents. One should remember that clay minerals can be regenerated by combustion without loss, due to the stability of their structure even at temperatures up to 850 °C.
Amounts of sorbents in kg needed to reduce concentration of PPCPs by 50% in acidic and neutral aquatic solutions.
B (3.8) | Mt (4.7) | K (3.1) | VER (3.1) | MtK10 (3.9) | MtK30 (3.6) | MtG5%T (3.1) | BAlG3%C (4.0) | |
CBZ | 37.7 | 34.2 | 38.4 | 28.0 | 39.2 | 22.8 | 30.1 | 39.9 |
KTP | 38.6 | 26.9 | 37.2 | 31.0 | 21.3 | 17.5 | 40.5 | 64.6 |
BPA | 40.9 | 25.9 | 35.6 | 29.2 | 70.1 | 44.4 | 34.5 | 4.8 |
DCF | 30.3 | 13.6 | 26.3 | 16.0 | 4.2 | 7.1 | 14.9 | 13.1 |
IBP | 38.5 | 30.9 | 43.2 | 32.9 | 17.2 | 11.6 | 40.3 | 5.3 |
TCS | 27.6 | 4.2 | 22.4 | 10.8 | 1.4 | 9.4 | 2.2 | 11.3 |
B (8.4) | Mt (8.4) | K (6.0) | VER (6.5) | MtK10 (8.3) | MtK30 (6.8) | MtG5%T (7.7) | BAlG3%C (7.7) | |
CBZ | 84.5 | 71.3 | 145.5 | 28.6 | 54.3 | 18.2 | 33.2 | 48.7 |
KTP | 132.6 | 68.1 | 155.4 | 36.9 | 172.7 | 14.2 | 31.2 | 108.9 |
BPA | 77.1 | 53.0 | 104.0 | 28.2 | 56.5 | 55.0 | 52.4 | 66.2 |
DCF | 171.8 | 64.3 | 152.0 | 30.6 | 110.2 | 5.8 | 56.0 | 98.4 |
IBP | 619.5 | 159.6 | 56.4 | 30.8 | 41.6 | 9.9 | 64.2 | 26.6 |
TCS | 46.4 | 17.3 | 50.2 | 17.3 | 1.6 | 8.2 | 2.1 | 14.0 |
4 Conclusions
The results of our study showed that unmodified montmorillonite, bentonite or kaolinite have poor capacity and are not versatile in absorbing different types of pollutants. Additionally, better sorption in acidic solutions makes their application difficult in the treatment of surface waters or wastewater of neutral pH. The results of sorption for the studied PPCPs showed the highest removal on carbonaceous sorbent BAlG3%C, vermiculite, and acid activated montmorillonites K10 and K30. The sorption capacities of vermiculite and montmorillonite K30 were practically independent of pH. This is of great importance for wastewater treatment plants, which generally operate at pH ∼8. Additionally, the low cost of vermiculite and its hydrophobicity make it very useful. In the case of K30, whose natural pH is 3.6, the increasing pH due to the interaction with weakly alkaline wastewaters should not affect its removal efficiencies. Finally, the removal capacities of the tested sorbents in real wastewater will be studied in the nearest future to determine the effects of combining pharmaceuticals properties, solution pH, and the nature of the sorbents.
Acknowledgements
This work was supported by AGH University grant No. 11.11.210.244.