1 Introduction
Ketoprofen, also known as [(RS)-2-(3-benzoylphenyl) propionic acid], is a commonly used pharmaceutical drug which possesses anti-inflammatory and analgesic activities because of its ability to inhibit cyclooxygenase enzymes that promote inflammation [1]. Ketoprofen is widely used in medical care because it is able to treat inflammatory diseases and musculoskeletal injury [2]. Because of the large quantity of ketoprofen consumed by humans, the compound is widely detected with other nonsteroidal anti-inflammatory drugs (NSAIDs) in wastewater and surface water [3–5]. Once consumed, 80% of ketoprofen is eliminated as unchanged drug and its degradation in wastewater treatment plants (WWTPs) depends on the biological treatment efficiency [6]. It has been previously reported that WWTPs are the primary source of pharmaceuticals in river water [5].
To date, many reports have emerged on the occurrence of NSAIDs such as ibuprofen, naproxen, and diclofenac in South African environment [7–13]. However, there are currently few studies that have reported on the presence of ketoprofen in South African aquatic conditions [11–13]. With the view of preserving the precious resource such as water, there is a need to understand the extent of all widely used pharmaceuticals in the environment. South Africa has a large number of WWTPs that are mainly used for domestic wastewater treatment and their potential for removal of pharmaceuticals such as ketoprofen is not known. There is currently a lack of data on the toxicity of ketoprofen in aquatic life. To understand the risk of aquatic life and water consumers from suffering the health effects caused by pollutant levels, it is necessary to fully evaluate the occurrence of ketoprofen in water resources.
To address this problem, the development of highly sensitive and selective methods for trace determination of compounds such as ketoprofen in complex wastewater matrix is required. One of the most suitable methods of ketoprofen analysis is the use of chromatographic methods that are equipped with a very sensitive mass spectrometry detector [14,15]. However, the operation, maintenance and cost, of mass spectrometry detector is expensive. Therefore, some laboratories had opted for the use of a cheap and readily available UV–visible detector. The sensitivity of UV–visible detector is usually improved by the use of solid-phase extraction (SPE) for cleanup and preconcentration of target compounds [16].
In SPE, the most widely used extraction media for ketoprofen are Strata X, C18, and Oasis hydrophilic lipophilic balanced (HLB) sorbents [17,18]. Although the application of these sorbents leads to the improvement of sensitivity, they often lack selectivity and their single use results in massive generation of solid waste. Nowadays, molecularly imprinted polymers (MIPs) are developed for SPE applications because of their properties that include high selectivity, reusability, mechanical strength, and resistance against acids, bases, and organic solvents [19]. The development and application of MIPs for the selective analysis of single NSAID such as ibuprofen and diclofenac is well documented [20,21]. The use of multitemplate MIPs for ketoprofen and several NSAIDs in wastewater analysis has been explored [22]. MIPs synthesized using the multitemplate approach are usually selective toward a group of compounds. However, these may not be useful in the analysis of a single analyte as it is important to obtain cleaner chromatograms which subsequently lead to more accurate measurements. Currently, there is a lack of available information for the synthesis of MIP that can selectively extract ketoprofen from aqueous samples.
Ketoprofen has been identified as one of the pharmaceutical drugs that contaminate Umgeni River which is found in the northern part of Durban city in South Africa [11,13]. With the exception of the work published by Madikizela et al. [12], there are currently no reports on the occurrence of ketoprofen in the southern region of Durban. Apart from these studies [11–13], there is currently no other South African study that has focused on the analysis of ketoprofen in water resources. Therefore, the aims of this study were to evaluate the occurrence and removal efficiency of ketoprofen in WWTPs located in the southern part of Durban city, South Africa. To achieve these aims, an MIP was synthesized, characterized, and applied in selective SPE of ketoprofen from wastewater before high-performance liquid chromatographic quantification.
2 Experimental section
2.1 Materials
Ketoprofen (≥98%), triclosan (≥97%), 2-vinylpyridine (97%), high-performance liquid chromatography (HPLC) grade methanol (≥99.9%), 1,1′-azobis-(cyclohexanecarbonitrile) (98%), ethylene glycol dimethacrylate (98%), and toluene (99.7%) were purchased from Sigma-Aldrich (Steinheim, Germany). HPLC grade acetonitrile (≥99.9%) and glacial acetic acid (100%) were purchased from Merck (Darmstadt, Germany). Formic acid (approximately 98%), fenoprofen (≥97%), and HPLC grade triethylamine (≥99%) were purchased from Fluka (Steinheim, Germany).
2.2 Synthesis of MIP
Published work was adopted with slight modifications for the synthesis of MIP for ketoprofen [23,24]. Synthesis was carried out by mixing 25 mg of ketoprofen with 54 μL of 2-vinylpyridine. The mixture was stirred at room temperature in a 250 mL round-bottomed flask containing 10 mL of a acetonitrile/toluene (1:9, v/v) porogenic mixture for 30 min. Thereafter, the reaction vessel was placed on ice to prevent unwanted polymerization. Ethylene glycol dimethacrylate (4.77 mL) and 100 mg of 1,1′-azobis-(cyclohexanecarbonitrile) were added. The mixture was purged with nitrogen gas for 10 min, sealed, and stirred in an oil bath at 60 °C for 16 h to initiate polymerization. After 16 h, the temperature was increased to 80 °C and maintained for 24 h to achieve a solid monolith polymer. The polymer was dried to constant mass at 80 °C followed by grinding and sieving. Particles ranging from 25 to 90 μm were collected and washed repeatedly with a mixture of acetic acid/acetonitrile (1:9; v/v) until ketoprofen could not be detected in high-performance liquid chromatographic system. Nonimprinted polymer (NIP) was synthesized and treated likewise with the omission of ketoprofen in the reaction mixture.
2.3 Apparatus
HPLC system consisting of an online mobile phase degasser unit (model DGU-20A5), 20 μL sample loop, pump (model LC-20AT), and UV–visible detector (model SPD-20A) obtained from Shimadzu Corporation (Kyoto, Japan) was used. The mobile phase conditions consisted of a mixture of acetonitrile and 0.2 formic acid in water (60:40, v/v) at a flow rate of 1 mL/min. Separation was performed on a Gemini C18 HPLC column of 150 × 4.6 mm × 5 μm obtained from Phenominex (California, USA). Shimadzu liquid chromatography (LC) solutions software was used for data collection and processing. Detector wavelength was set at 255 nm.
For characterization, thermal analysis was performed using thermogravimetric analysis/differential scanning calorimetry (TGA/DSC) 1 Stare system obtained from Mettler Toledo (Columbus, USA). Thermograms were recorded using a heating rate of 10 °C/min from 25 to 700 °C, under nitrogen atmosphere at a rate of 100 mL/min.
Diffraction patterns (10–90°) of the synthesized polymers were determined using an X-ray diffraction (XRD) equipped with XRD commander for data collection and Eva software for processing. XRD system was obtained from Bruker AXS (Karlsruhe, Germany). Cu Kα radiation source at a rate of 2°/min was used.
Elemental analysis was performed using Series II carbon, hydrogen, nitrogen, sulfur and oxygen (CHNS/O) analyzer 2400 from Perkin Elmer (Llantrisant, United Kingdom).
For SPE, manifold was purchased from Phenominex (California, USA), and it was connected into vacuum pump that was obtained from Merck Millipore (Massachusetts, USA).
2.4 Sampling
Samples analyzed consisted of raw influent collected after wastewater screening for solid removal and final effluent sampled after disinfection of treated water with chlorine. Wastewater samples were collected once per week in the month of May in 2016 from Amanzimtoti (Global positioning system (GPS), S30.00749° E30.91720°), Kingsburgh (GPS, S30.07445° E30.85687°), and Umbilo (GPS, S29.84556° E30.89103°) WWTPs located around the city of Durban in KwaZulu-Natal Province of South Africa. The samples were transported into the laboratory, where they were filtered through a 0.22 μm syringe filter; thereafter, pH was adjusted to 5. Samples were refrigerated at 4 °C until analysis.
2.5 Preparation and application of SPE
Empty SPE cartridges (1 mL) purchased from Supelco (Bellefonte, USA) were mounted into the manifold and washed with methanol before their use. Thereafter, 14 mg of MIP particles were packed in between two polypropylene frits.
Water samples were percolated through the packed cartridge using the preoptimized SPE conditions. This was achieved by conditioning the cartridge with 1 mL of methanol followed by equilibration with 1 mL of deionized water. Fifty milliliters of wastewater sample (pH 5) was loaded at 1 mL/min. The cartridge was vacuum dried for 10 min, followed by washing with 1 mL of 5% (v/v) triethylamine in water. The retained ketoprofen was eluted with 1 mL of methanol and injected into HPLC.
After each use, the cartridges were regenerated by washing with 3 mL of deionized water and 3 mL of methanol.
2.6 Quality assurance
A stock solution of 100 mg/L ketoprofen was prepared in acetonitrile. The stock solution was further diluted to prepare the working standards. The working standards were analyzed using HPLC. Calibration curve, limit of detection (LOD), and limit of quantification (LOQ) were computed. The accuracy and precision of analytical method were validated using deionized water and wastewater samples that were spiked with ketoprofen at concentration levels ranging from 5 to 1000 μg/L.
3 Results and discussion
3.1 Characterization of polymers
Thermogravimetric analysis was performed for the washed MIP and NIP (Fig. 1). At 40 °C, the washed polymers had a mass loss of approximately 4%. This was probably because of the adsorbed methanol used in the template removal step [25]. Further thermal decomposition of polymers was observed at 290 °C which was marked as the temperature where the polymer backbone collapses. In a separate study, the polymer backbone collapsed at 250 °C for an MIP that was synthesized for 1,3-diisopropylurea [25]. It was further observed that at 425 °C, there was 100% thermal decomposition for the NIP, whereas the corresponding mass loss was 90% for the MIP. The difference might have been caused by structural variations that could have happened during template removal process.
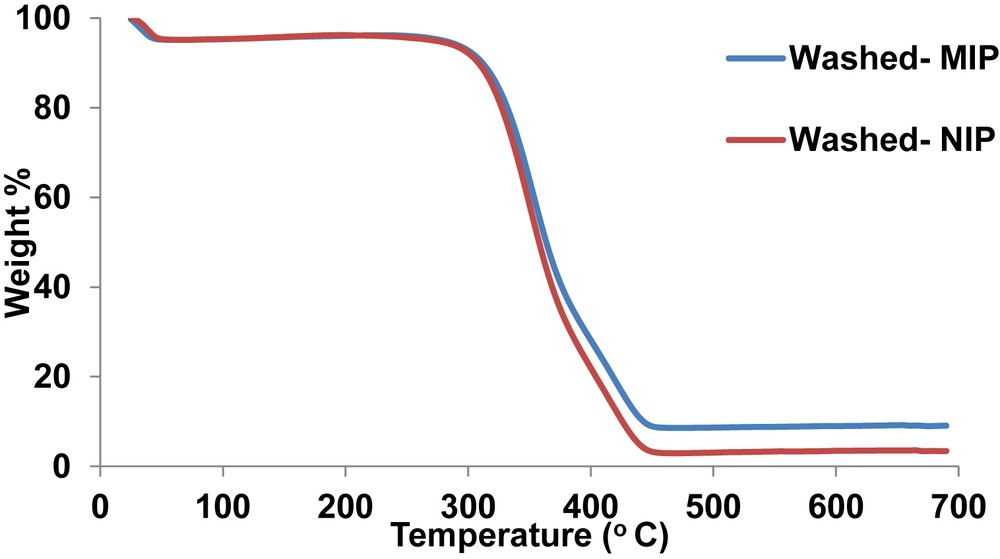
Thermogravimetric analysis of the synthesized polymers.
Differential scanning calorimetry thermograms (Fig. 2) of the MIP and NIP were similar with endothermic peak at 355 °C which is associated with the temperature where there is complete thermal decomposition of the polymers. Similarities in the thermograms could be because of similar structural arrangements of the MIP and NIP. Structural similarities were further confirmed with X-ray diffractograms (Fig. S1). The lack of peaks in diffractograms is an indication of amorphous polymers.
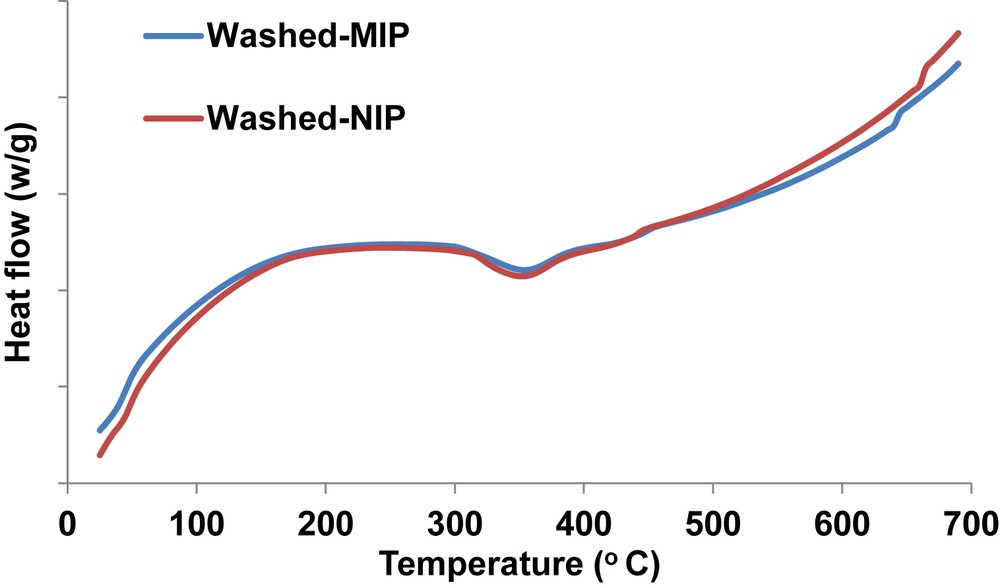
DSC curves for the polymers.
The synthesized polymers were analyzed in terms of their carbon, nitrogen, and hydrogen contents using an elemental analyzer. As indicated in Table 1, the carbon and hydrogen contents were identical because of similar conditions in the synthesis. Also, this is an indication of a successful template removal as the presence of ketoprofen in the MIP could result in higher carbon and hydrogen contents. The sources of nitrogen in the polymers are the initiator and functional monomer used in synthesis. The differences in the nitrogen contents of polymers can be explained by the possible disruptions in the chemical structure of the MIP during excess washing while removing ketoprofen. This was evident in the chromatograms obtained during template removal where there were unknown peaks recorded for the MIP solutions. Oxygen is the only other element (not quantified) known to be present in both polymers. The sources of oxygen in polymers are cross-linker and template used in polymerization.
Measured percentage by mass of carbon, hydrogen, and nitrogen of the polymers using CHN analyzer.
Polymer | Carbon (%) | Hydrogen (%) | Nitrogen (%) |
Washed MIP | 58.28 | 7.39 | 0.33 |
Washed NIP | 59.68 | 7.33 | 1.0 |
3.2 SPE studies
3.2.1 Optimization
Optimization was carried out by using deionized water spiked with 1 mg/L of ketoprofen as the sample. SPE parameters investigated include sample pH, sorbent mass, sample volume, and washing solvents. Because the target compound is polar, methanol was used for its elution from the SPE cartridge. During optimization, only one parameter was changed at a time whereas keeping other parameters constant. Each experiment was conducted in triplicate, where average results are discussed.
For the adsorption of acidic pharmaceuticals, it has been reported that the extraction is based on hydrogen binding of the target compound and functional monomer (2-vinylpyridine) [26]. In this context, pH of the water solutions was adjusted to promote the monomer–template interactions. pH was investigated in the range of 3–7, where the highest recovery was obtained at pH 5 (Fig. 3). Therefore, pH 5 was selected as optimum and used in all subsequent experiments. At selected pH, the recovery for ketoprofen using NIP was 69.5% which could be because of the adsorption based on nonspecific interactions, whereas for the corresponding MIP, 88% was obtained because of molecular recognition. pH values greater than 7 were not investigated because of the deprotonation of ketoprofen which could lead to unavailability of adsorption sites. This behavior is somewhat similar to the behavior of other acidic pharmaceuticals on the MIP [27].
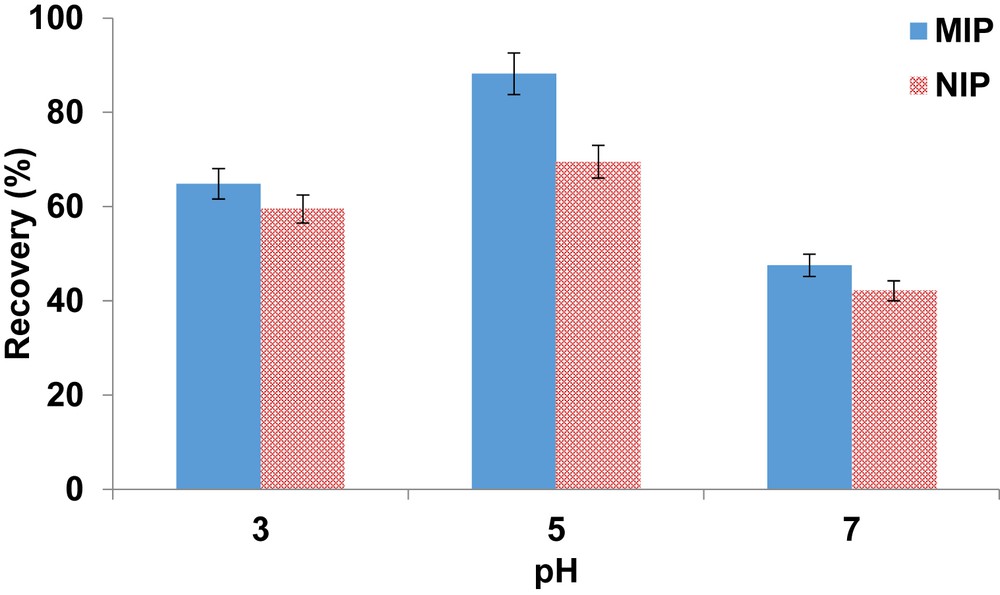
Effect of pH on the recovery of ketoprofen from the polymers. Extraction conditions were as follows: sample volume, 20 mL; washing solvent, 1 mL of deionized water; and elution solvent, 1 mL methanol.
The quantity of the polymer for the optimum extraction of ketoprofen was investigated. It was discovered that low mass of the polymers resulted in poor recoveries which could be a result of maximum occupation of the binding sites. However, the accepted recovery was achieved by using 14 mg of the polymer (Fig. 4). It should be noted that this work was investigated using 1 mg/L of ketoprofen which is much higher than the concentrations expected in wastewater samples. Therefore, the amounts of polymers beyond 14 mg could not be investigated.
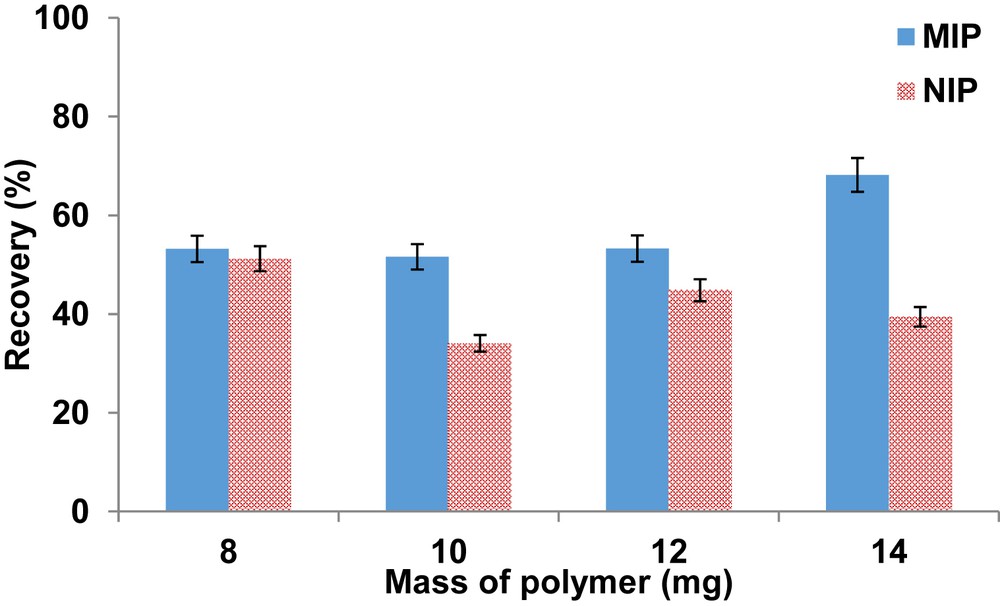
Effect of polymer amount on the recovery of ketoprofen. Extraction conditions were as follows: sample volume, 50 mL; washing solvent, 1 mL of deionized water; elution solvent, 1 mL methanol; and pH, 5.
High sample volumes are often required in environmental analysis as they tend to lead to high preconcentration factors which in turn produce better sensitivity. The results in Fig. 5 show that the recovery increased from the sample volume of 10–20 mL for both polymers because of limited analyte interaction with the polymer as not enough volume was available to percolate the whole sample through the sorbent. Accepted recoveries (>70%) were achieved in the sample volumes of 20 and 50 mL for the MIP. However, 50 mL was selected as optimum as it leads to higher preconcentration factor. Recoveries decreased beyond the sample volume of 50 mL for the MIP which could be because of the capacity of the polymers being exceeded. Because of limited binding sites in the NIP, maximum recovery was obtained when the sample volume was 20 mL.
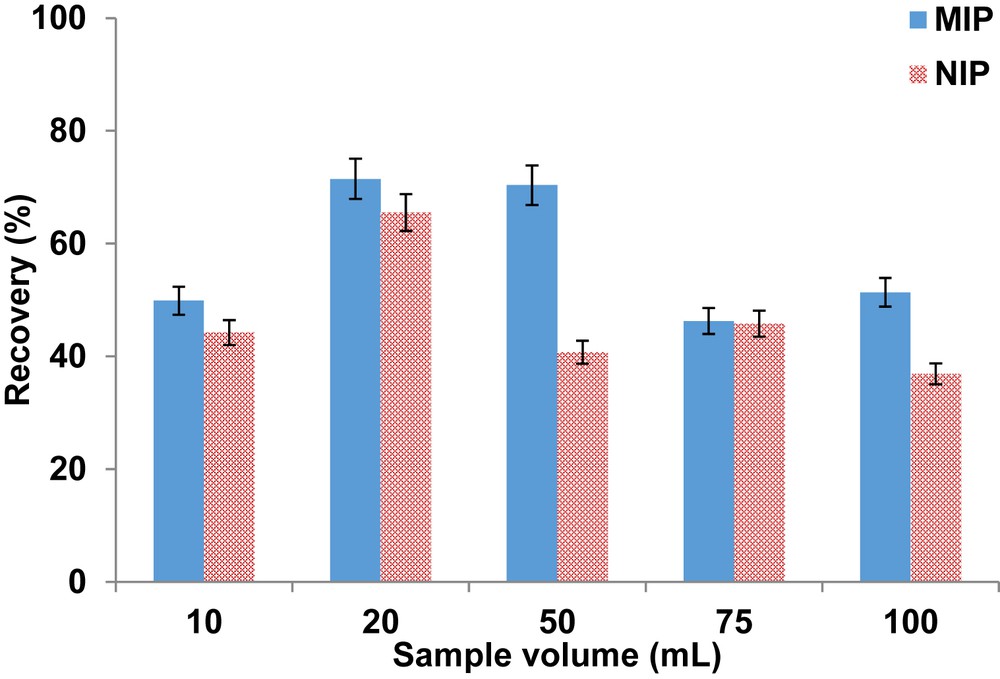
Effect of sample volume on the recovery of ketoprofen. Extraction conditions were as follows: washing solvent, 1 mL of deionized water; elution solvent, 1 mL methanol; sorbent mass, 14 mg; and pH, 5.
3.2.2 Selectivity
The selectivity of the polymers was investigated using deionized water that was spiked with ketoprofen, fenoprofen, gemfibrozil, and triclosan. Fenoprofen and gemfibrozil are acidic pharmaceuticals that contain similar functional groups as ketoprofen (Table 2). These two pharmaceuticals are usually present in wastewater solutions that contain ketoprofen [28,29]. Triclosan is an antibacterial compound usually detected in wastewater with ketoprofen [12,30]. These competitors were expected to be adsorbed by the MIP through the formation of hydrogen bonds because of the presence of hydroxyl groups in their molecules.
Molecular structures of ketoprofen and competitors.
Compound | Molecular structure |
Ketoprofen | |
Triclosan | |
Gemfibrozil | |
Fenoprofen |
The results show low recoveries (<20%) for all competitors which may be because of differences in their molecular shapes and sizes compared to ketoprofen. Therefore, the prepared polymers were highly selective toward ketoprofen (Tables S1 and 3). However, high ketoprofen recovery in the NIP could indicate a nonspecific adsorption which could be problematic considering the complex wastewater matrix. Therefore, selective washing was investigated in this case. Ten percent was recovered for triclosan when the MIP cartridge was washed with water, 17% was obtained for the corresponding NIP. Further washing solvents were investigated for selective removal of competitors which could result in cleaner chromatograms when the method is applied in wastewater analysis. Addition of organic solvents in the washing solutions resulted in elution of ketoprofen together with competitors for the NIP cartridges. This is a result of nonspecific binding. Therefore, the cartridges packed with NIP were discarded because of poor selectivity. However, in the case of the MIP, only competitors were removed during washing as a result of molecular imprinting. The introduction of acetonitrile in the washing solvent resulted in the reduction of ketoprofen recovery in the case of the MIP as well.
Effect of washing solvent in SPE of ketoprofen using the MIP.
Compound | % Recovery | ||||
H2O | MeOH/H2O (5:95, v/v) | TEA/ACN (1:99, v/v) | TEA/H2O (1:99, v/v) | TEA/H2O (5:95, v/v) | |
Ketoprofen | 69.06 | 67.61 | 33.39 | 107.85 | 103.98 |
Fenoprofen | 5.81 | 1.98 | 2.49 | 1.17 | 2.68 |
Gemfibrozil | 0.96 | 1.03 | 0.59 | 1.85 | 0.15 |
Triclosan | 9.93 | 9.56 | 2.31 | 13.76 | 12.64 |
3.3 Validation of the analytical method
The chromatographic analysis of ketoprofen was achieved on a reverse-phase stationary phase (Fig. S2). The performance of the proposed analytical method that uses MIP as a selective sorbent for SPE was validated based on sensitivity, accuracy, and precision. A linear plot with R2 greater than 0.99 was achieved for a seven-point calibration curve in the concentration range of 0.001–10 mg/L. On the basis of LOD and LOQ results shown in Table 4, the developed method is highly sensitive. LOQ and LOD are defined as concentrations that gave the signal-to-noise ratio of 10 and 3, respectively. Greater preconcentration factor in the proposed method led to better method sensitivity as compared with the data in the literature [31]. The results in Table 4 show that the analytical method was highly accurate as indicated with recoveries in the range of 68–114%. The relative standard deviation (RSD) given as ± values indicate that the developed method is precise.
LOD, LOQ, recovery (%), and RSD (%) values (n=3) achieved for the deionized water and wastewater influent and effluent spiked with ketoprofen.
Spiked sample | LOD (μg/L) | LOQ (μg/L) | Recovery (%) ± RSD (%) | ||
1000 μg/L | 25 μg/L | 5 μg/L | |||
Deionized water | 0.09 | 0.30 | 68 ± 3 | 80 ± 17 | 114 ± 16 |
Influent | 0.23 | 0.78 | – | – | 68 ± 10 |
Effluent | 0.17 | 0.55 | – | – | 68 ± 15 |
3.4 Wastewater analysis
The developed procedure was applied in the SPE of ketoprofen from wastewater before high-performance liquid chromatographic quantification. Ketoprofen was detected in all wastewater samples (Table 5) with concentrations greater than 2 μg/L in the effluent, whereas the levels were much higher in the influent. Chromatograms were cleaner for the effluent when compared with influent samples because of effective wastewater treatment process (Fig. 6). Detected levels of ketoprofen were compared with previous concentrations reported locally and in other countries. Previous work [12] reported the concentration range of 1–6 μg/L in Amanzimtoti WWTP influent, whereas the present study reports the average concentration of 28.4 μg/L. The differences could be because of seasonal variations as the samples were collected from August to October in the previous work [12], whereas the samples of the present study were collected in May. Also, the variations in population dynamics might be another factor to be considered. Overall, the concentrations of ketoprofen detected in these plants were higher than the levels reported in South Africa and the rest of the world (Table S2) because of differences in WWTP designs and consumption of the drug in foreign countries [32–36]. Most recently, ketoprofen is one of the pharmaceuticals that have been detected in wastewater and surface water from several countries including Algeria and China [6,37,38].
Removal rates and concentrations (μg/L) ± standard deviations (n = 3) of ketoprofen in wastewater samples.
WWTP | Influent | Effluent | %Removal |
Amanzimtoti | 28.4 ± 1.1 | 3.50 ± 2.4 | 88 |
Kingsburgh | 28.2 ± 12 | 3.40 ± 3.1 | 88 |
Umbilo | 27.3 ± 0.57 | 2.90 ± 2.5 | 90 |
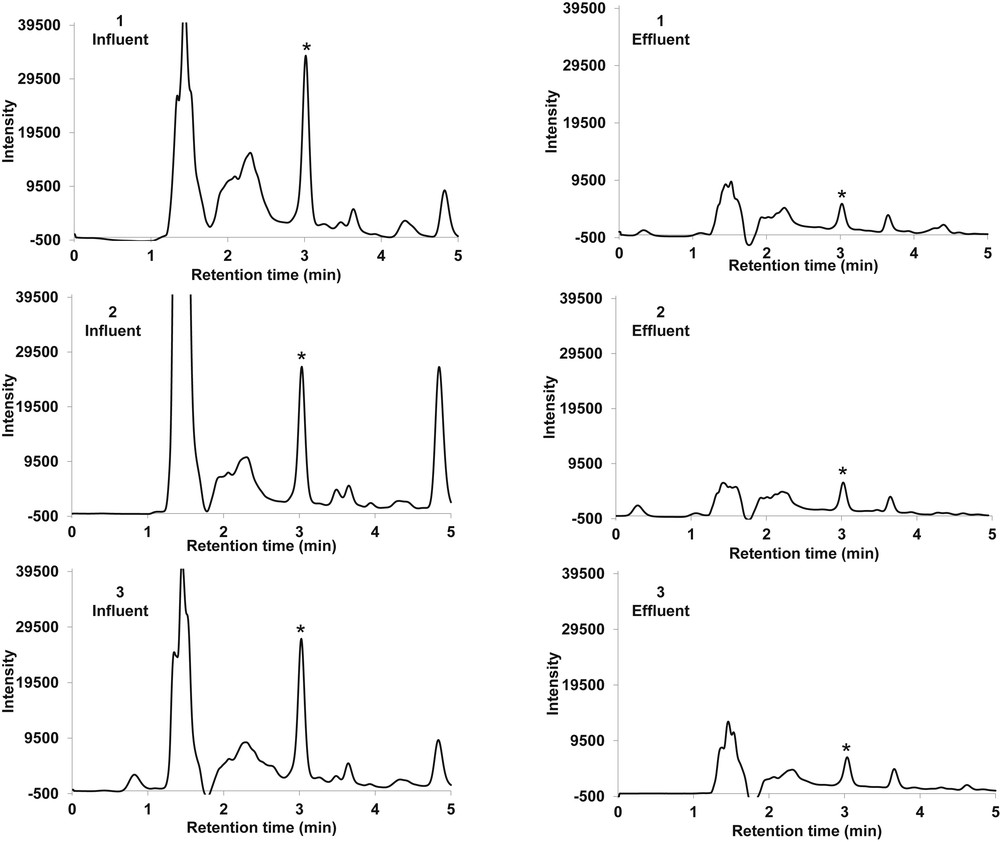
Typical chromatograms obtained for wastewater influent and effluent collected from Kingsburgh (1), Umbilo (2), and Amanzimtoti (3) WWTPs. ∗Peak for the target compound (ketoprofen).
To the best of our knowledge, this is the first detailed study based on the occurrence and removal rate of ketoprofen in Amanzimtoti, Kingsburgh, and Umbilo WWTPs. Removal rates were determined based on the concentrations present in both influent and final effluent. Therefore, the performance of these WWTPs in terms of reducing ketoprofen during treatment process was compared with various plants in the world (Table S3). It was observed that the sampled WWTPs perform in a similar manner and sometimes better when compared with various plants for the reduction of ketoprofen during the wastewater treatment [30,39–41].
4 Conclusions
For the first time, a selective MIP for ketoprofen was synthesized and applied as the SPE sorbent. SPE technique was optimized and used with HPLC for the quantitative determination of ketoprofen in Umbilo, Amanzimtoti, and Kingsburgh WWTPs. For wastewater analysis, ketoprofen was detected in all samples. Concentrations of ketoprofen found in this study were higher when compared with what has been reported for WWTPs located in Europe. The removal rate of ketoprofen during domestic wastewater treatment was in the range of 88–90%. These results call for a detailed screening of ketoprofen in other South African water bodies including river and dam water. The analytical methodology used for wastewater analysis was fast, highly accurate, sensitive, and selective and gave results with good precision.
Acknowledgments
This work was supported financially by the National Research Foundation (NRF) of South Africa, grant numbers TTK 150618119659 and TTK14042666625 and Eskom through Tertiary Education Support Program. Durban University of Technology and University of Witwatersrand are thanked for allowing us to use their research/teaching facilities. EThekwini Municipality and WWTPs are acknowledged for sample collection arrangements.