1 Introduction
There is a growing interest in natural and unnatural antioxidants as a protective strategy against various diseases such as artherosclerosis, ischemia-reperfusion injury and inflammatory injury by blockage or removal of oxidative stresses [1]. Ascorbic acid, vitamin E and polyphenols are the major antioxidants used as dietary supplements for the prevention of such diseases. Phenolic compounds have many industrial applications as natural additives with antimicrobial and/or antioxidant properties that are important in protecting the nutritive value and increasing the shelf life of cosmetic and food products. In addition, they are considered as one of the bioactive compound groups which are responsible for beneficial health effects [2,3]. From a chemical point of view, phenolic compounds can be classified in different ways because they are constituted by a large number of heterogeneous structures that range from simple molecules to highly polymerized compounds [4]. The antioxidant activity of phenolic compounds depends largely on the chemical structure of these substances [5] with flavonoids, tannins, chalcones and coumarins as well as phenolic acids, being the most highlighted ones. For instance, antioxidant activity of phenolic acids, especially hydroxycinnamic acids and hydroxybenzoic acids, is usually determined by the number of hydroxyl groups found in the molecule thereof [6].
Although a great amount of phenolic compounds can be found in plants, it could be very interesting to easily synthesize them with competitive prices, so that industrial investigation of their biological properties can occur more frequently. Accordingly, many different routes were published for total synthesis of phenolic antioxidant compounds. Among useful transformations, hydroxylation of aromatic compounds stands out as a fundamental reaction due to its many uses in the manufacture of high-added-value compounds, like orthodiphenol compounds such as hydroxytyrosol (3,4-dihydroxyphenylethanol) and 3,4-dihydroxyphenyl acetic acid [7].
In the course of the development of an efficient synthetic route of a potent antioxidant, we can describe herein a new alternative method of a two-step-synthesis of 3,4-dihydroxyphenyl acetic acid using the advanced oxidation of phenyl acetic acid under mild conditions. Actually, this synthetic route is based on literature analogies used for the synthesis of hydroxytyrosol [8]. Though the difference with the latter is the use of acid-activated clay instead of (Al–Fe) pillared one without ultra-violet light. As a matter of fact, acid-activated clays are one of the most widely studied solid acid catalysts for many organic transformations due to their environmental compatibility, low cost, high selectivity and thermal stability [9].
2 Materials and methods
2.1 Reagents
Phenylacetic acid (PAA) was obtained from Sigma-Aldrich (St. Louis, MO). para-hydroxyphenyl acetic (p-HPAA) acid and 3,4-dihydroxyphenyl acetic (3,4-DHPAA) used as standards for chromatographic calibration were also purchased from Sigma-Aldrich. Hydrogen peroxide (30% solution in water) was obtained from CePharma (Tunisia). Deionized water was used throughout the experiments and in the HPLC mobile phase. Pure HPLC solvents were used in all cases.
2.2 Catalysts
The commercial clays (Montmorillonite KSF/0 clay, Montmorillonite KSF clay and Montmorillonite K10 clay) used as catalysts in this study were purchased from Fluka and used without further treatment. In fact, the relevant physico-chemical properties of the catalysts used are given in Table 1 [10]. The total acidity of the catalysts was determined by NH3 adsorption. Following a 2 h pretreatment at 300 °C, NH3 was adsorbed at ambient temperature. After evacuating, the amount of the chemisorbed NH3 was measured applying 2 °C/min heating rate till 500 °C.
Some characteristic data of the investigated catalysts.
Catalyst | Textural characteristics | Analysis (%) | Acidity | ||||
Specific surface area (m2/g) | Average pore diameter (Å) | Al | Fe | pH | IR spectra (AU) | ||
Brønsted | Lewis | ||||||
KSF/0 | 117 | 74 | 6.16 | 1.37 | 1.3 | 1.03 | 0.20 |
KSF | 30 | 50 | 8.62 | 3.17 | 1.5 | 0.59 | 0.15 |
K10 | 249 | 56 | 7.71 | 1.99 | 4.5 | 0.33 | 0.29 |
2.3 Phenyl acetic acid conversion
Phenylacetic acid oxidation was carried out in a 100 ml Pyrex reactor equipped with a magnetic stirrer. The solid catalyst was introduced into 50 mL of PAA aqueous solution (500 mg L−1), under continuous stirring. The desired quantity of H2O2 (0.02 M) was added indicating the start of the reaction. During the experiments, aliquots were withdrawn at regular intervals with the purpose of monitoring the conversion of PAA to p-HPAA and then to 3,4-DHPAA after being immediately filtered to completely remove catalyst particles.
2.4 HPLC analysis
Monomeric phenols (PAA, p-HPAA and 3,4-DHPAA) were analysed on a high performance liquid chromatograph (Dionex HPLC) equipped with a C18 column (4.6 mm × 250 mm; Shimpack VPODS).
2.5 LC–MS/MS analysis
LC-UV-MS/MS analyses were performed as described by Kite et al. [11] on a Thermo Scientific System consisting of an Accela U-HPLC unit with a photodiode array detector and an LTQ Orbitrap XL mass spectrometer fitted with an electrospray source. Chromatography was performed with 5 μl sample injections onto a 150 × 3 mm i.d., 3 μm, Luna C18(2) column (Phenomenex) using a 400 μl/min linear mobile phase with a methanol/water/acetonitrile + 1% gradient. Formic acid changes from 0:90:10 to 90:0:10 over 20 min followed by an isocratic phase for 5 min afterwards, a column washing phase for equilibrium per-pauses was performed during 3 min before the next injection. The ESI source of the mass spectrometer was operated in both positive and negative modes under the recommended manufacturers’ conditions for the mobile phase parameters. An orbitrap mass analyser was set to scan in a range of m/z 200–2000 at 30,000 resolutions in one polarity, while the linear ion-trap analysis was performed. Analyses of MS were due on the most abundant ions in both polarities using an ion isolated window of +2 m/z and relative collision energy of 35%. For accurate mass analyses of product ions generated by MS2 in the ion trap, were scanned by the orbitrap at 7000 resolutions. Negative mode ESI was used since the phenolic compounds in question ionize better in this mode, as described by other authors about these species [12]. Acidification of the LC mobile phase allows the best separation, since the hydroxyl groups in the compounds are kept in their acidic form, thereby increasing their retention on the column and decreasing peak broadening.
2.6 TOC removal and leaching-off of Fe ions
The total organic carbon TOC of the solution (in mg of carbon per litre of solution) was measured with a Shimadzu Model 5050 TOC-analyzer. Iron leaching was evaluated by measuring iron concentrations at the end of the conversion reaction using an atomic absorption analyzer, a PerkinElmer 1101 B, with a hollow cathode lamp having 8 mA current.
2.7 Antioxidant activity
Evaluation of samples' antioxidant activity withdrawn at regular intervals was assessed by the DPPH (1,1-diphenyl-2-picryl hydrazyl) radical scavenging assay [13]. Aliquots (50 μL) were added to 5 mL of methanolic solution containing DPPH radicals (6 × 10−6 mol L−1). After 30 min of incubation period under room temperature and in the dark, the absorbance was read at 520 nm using a Shimadzu UV-160 A spectrophotometer. DPPH radical scavenging activity was calculated as follows:
A lower absorbance of the reaction mixture indicates a higher DPPH radical-scavenging activity. Butylated hydroxyanisole (BHA) was used as a standard. The test was carried out in triplicate.
2.8 Statistical analysis
Results were expressed as mean ± standard deviation (mean ± SD). The whole analysis was carried out with GraphPad Prism 4.02 for Windows (GraphPad Software, San Diego, CA). Significant differences for comparison of the yield of PAA conversion were determined by one-way ANOVA, followed by Tukey's post-hoc test for multiple comparisons with a statistical significance of p ≤ 0.05.
3 Results and discussion
3.1 Phenyl acetic acid conversion: preliminary experiments
A variety of montmorillonite catalysts KSF, KSF/0 and K10 were investigated in the PAA acid conversion. Montmorillonite is a member of the smectite clay family and has a 2:1 structure i.e. an octahedral layer of Al is sandwiched between two tetrahedral layers of silicon coordinated with oxygen (TOT) exhibiting overall weak acidity. The crystalline sheets of negatively charged aluminosilicates are balanced by hydrated cations (Na+, K+ or Ca2+) in the interlayer spaces of montmorillonite. The most interesting features of the smectite clay are their intercalation, swelling and cation exchange capacity which improve the catalytic properties of smectite clay [14]. Fig. 1 shows the conversion profiles of the reaction times at the room temperature over the studied catalysts in the presence of hydrogen peroxide. Blank controls made without the catalyst were also analysed, and no significant (p > 0.05) PAA conversion was observed. However, it can be noted that the reaction rate increased (p < 0.05) when adding acid-activated catalyst clays according to the following order: K10 < KSF ≈ KSF/0. The enhancement of PAA conversion is due to the increase in hydroxyl radicals by the combination of the oxidant hydrogen peroxide and the redox process in the presence of iron ions.
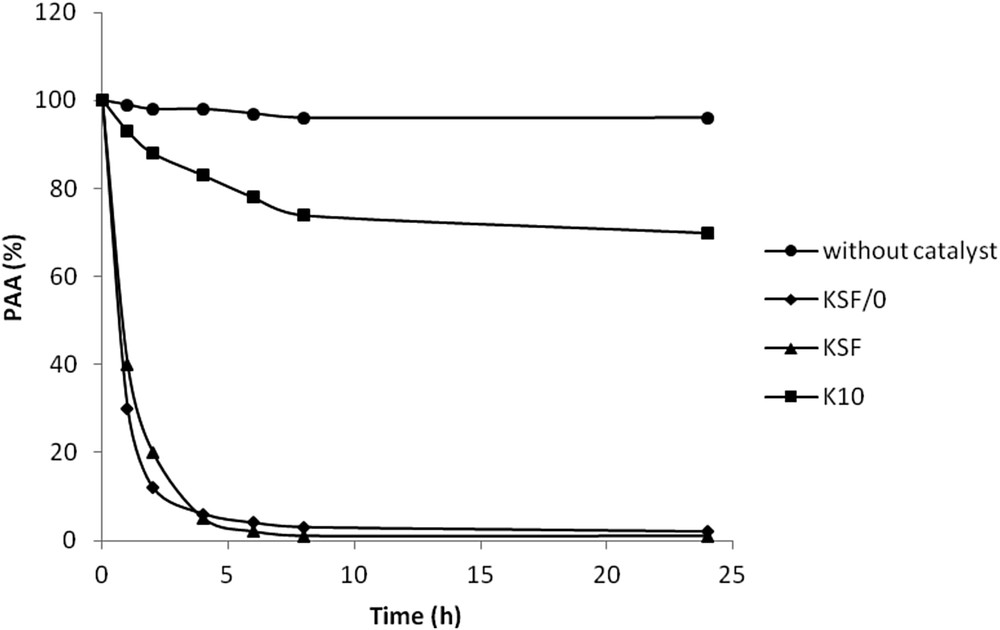
PAA oxidation by: H2O2, H2O2/KSF/0, H2O2/KSF, H2O2/K10. [PAA] = 500 mg/L, T = 298 K, [H2O2] = 2 × 10−2 M, and [catalyst] = 0.5 g/L.
Acid treatment in commercial-type montmorillonite KSF/0, KSF and K10 is performed using H2SO4.This behaviour promoted an increase in the number of acid sites of moderate strength compared to natural clay [15]. The acid strength of the catalysts can be characterized by the pH value of their 10% water suspension, while the number of sites by the intensities of the Brønsted- and Lewis-characteristic bands in the IR spectra after pyridine adsorption as previously described (Table 1) [10]. The acid strength increases from K10 to KSF and KSF/0. Based on the data shown in Table 1, no correlation was found between either the surface area or average pore diameter with the catalytic activity. Indeed, K10, which recorded the highest surface area (249 m2/g), led to the slowest reaction rate. The increase of the catalytic conversion could be correlated to the total acidity surface of the catalyst. Besides, lower pH clay values resulted in a significant increase (p < 0.05) of the reaction rate. Both KSF and KSF/0 with initial pH of 1.5 and 1.3, respectively, led to total PAA conversion, where only 30% PAA conversion yield was observed for the K10 catalyst at initial pH 4.5. KSF/0 and KSF are more active in the conversion of PAA because of their stronger acidity. So the catalytic activity of these K-catalysts is controlled by their acidity.
On the other hand, higher catalytic activity of KSF could be attributed to its high iron content. As a matter of fact, hydroxyl radicals can be easily produced through a redox process known as the Fenton reaction in the presence of iron ions [16]. They are generally schematized as follows:
Fe2+ + H2O2 → Fe3+ + OH− + °OH |
Fe2+ + °OH → Fe3+ + OH− |
RH + °OH → H2O + °R |
°R + Fe3+ → R+ + Fe2+ |
Even with its lower iron content compared to KSF's one, the KSF/0 catalyst yielded high PAA conversion similar to KSF. This result could be attributed to the high acidic pH (2.97) of the aqueous phenolic solution mixed with KSF/0 particles (Table 2). Such acidic pH values (around 3) have been reported to be optimum for homogenous Fenton processes. The latter corresponds to the maximum concentration of the active Fe2+ species and the lowest rate of H2O2 parasitic decomposition. By increasing the pH, the precipitation of the insoluble ferric hydroxides takes place and the decomposition of H2O2 becomes preponderant [17].
pH Values, TOC removal and leaching-off of Fe ions throughout the catalytic reaction run.
Catalyst | Time (h) | pH | TOC | Fe leaching (ppm) |
KSF | 0 | 3.5 | 359 | 0 |
2 | 2.97 | 342 | – | |
4 | 2.95 | 230 | – | |
6 | 2.94 | 323 | – | |
8 | 2.91 | 325 | – | |
24 | 2.58 | 326 | 4.37 | |
KSF/0 | 0 | 2.97 | 359 | 0 |
2 | 2.94 | 341 | – | |
4 | 2.92 | 335 | – | |
6 | 2.91 | 325 | – | |
8 | 2.87 | 330 | – | |
24 | 2.67 | 329 | 1.57 |
3.2 Phenylacetic acid conversion: identification of reaction products
A typical catalytic reaction behaviour is shown in Fig. 2a and b. After a short induction period, PAA was rapidly converted to new phenolic reaction intermediates until complete disappearing. Throughout the reaction course, an interesting observation was noted. The initial transparent colour of the aromatic solution has been changed from colourless to yellow to brown and the intensity of the latter increased with time (Fig. 3).
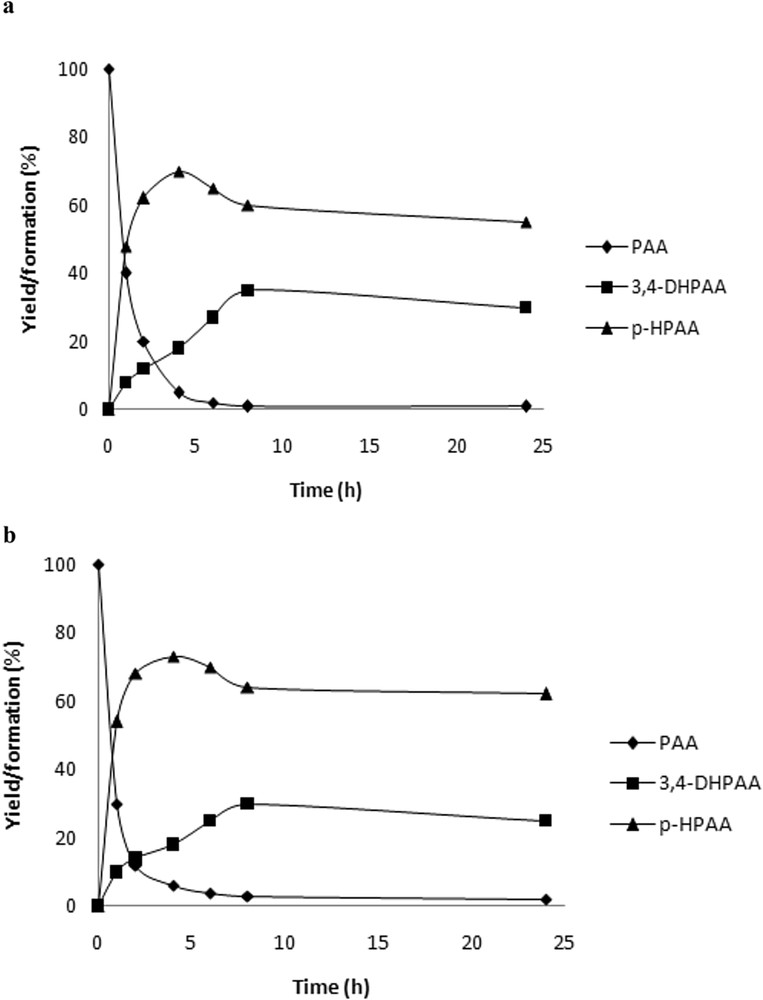
a. PAA oxidation by H2O2/KSF. [PAA] = 500 mg/L, T = 298 K, [H2O2] = 2 × 10−2 M, and [catalyst] = 0.5 g/L. b. PAA oxidation by H2O2/KSF/0. [PAA] = 500 mg/L, T = 298 K, [H2O2] = 2 × 10−2 M, and [catalyst] = 0.5 g/L.
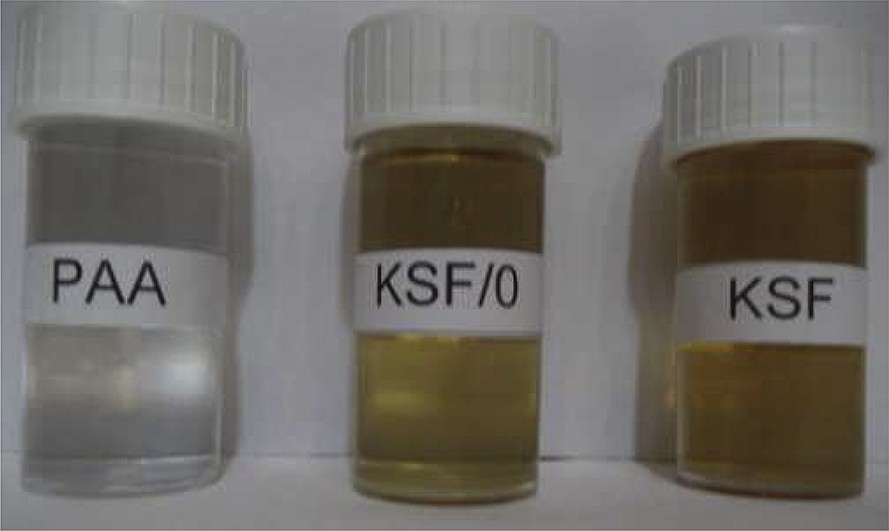
Colour aqueous solution before and after the PAA conversion with KSF and KSF/0 clay.
Newly appearing phenolic compounds were identified by LC-MS/MS, equipped with an electrospray mass spectrometer in the negative mode, was carried out by comparing retention times and mass spectra of the unknown peaks with the standards'. The structure assignment of phenolic compounds was based on UV absorbance spectra with a systematic search for molecular ions, using extracted ion mass chromatograms. The recorded MS/MS spectra are then compared to those reported by the literature [18,19].
Compound 1 was identified as being 3,4-dihydroxyphenyl acetic acid retained at 7.16 min (λmax, 268) (Fig. 4). The MS1 spectrum (Fig. 4a) exhibited a molecular ion at m/z 167 [M−H]−. The MS2 spectrum obtained by fragmentation of the ion m/z 197 presented the following m/z values: 167, 150 and 149. The pseudo molecular ion [M−H]− fragmented at m/z 167 yielded a fragment at m/z 149 by the loss of 18 mass units of [M−H2O−H]−. The structure of these compounds was confirmed with those of an authentic standard [20].
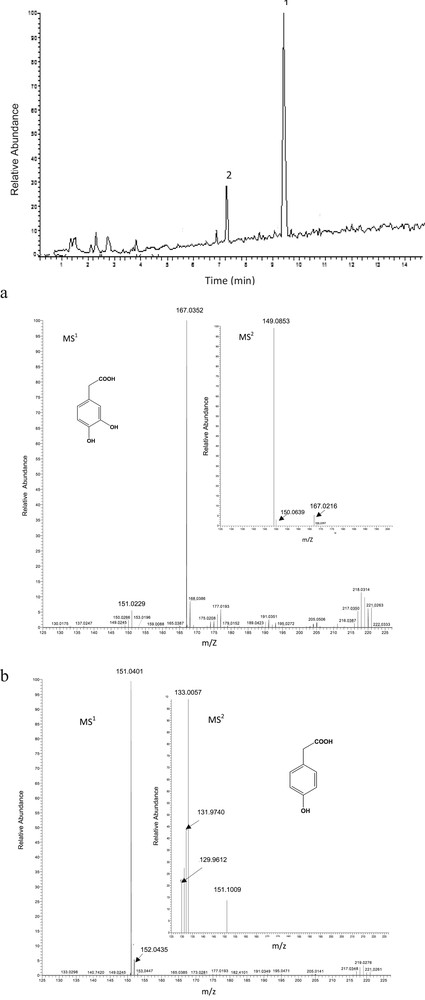
Detection by LC of para-hydroxyphenyl acetic acid and 3,4-dihydroxyphenyl acetic acid. a. LC-MS/MS spectrum (MS1 and MS2) of 3,4-dihydroxyphenyl acetic acid. b. LC-MS/MS spectrum (MS1 and MS2) of para-hydroxyphenyl acetic acid.
Compound 2 (Rt, 9.31 min; λmax, 270 nm) (Fig. 4 and b) was identified as being para-hydroxyphenyl acetic acid, based on chromatography analysis with an authentic standard and a mass spectrum with a [M−H]− at 151 and prominent MS2 fragments at m/z 151, 133. The ion m/z 133 obtained by the loss (−18) of a H2O molecule provided an anion of [M−H−H2O]−.
Taking into account the LC-MS/MS finding, it is possible to presume that the PAA conversion behaviour would be as follows: PAA led to the p-HPAA which itself was converted to the high-added value 3,4-DHPAA. Despite the complete PAA conversion after the 4 h reaction, an accumulation of 3,4-DHPAA was continuously observed.
3.3 pH, TOC and Fe leaching-off evolution
Table 2 presents the pH value, TOC removal and Fe ions' leaching-off throughout the catalytic reaction conducted by the most efficient catalysts (KSF and KSF/0). It was observed that the pH decrease was more significant (p < 0.05) for KSF (from 3.5 to 2.5) than KSF/0 catalysts (from 2.97 to 2.67). The latter were associated with less Fe leaching-off varying between 4.37 ppm and 1.57 ppm for KSF and KSF/0, respectively. This finding is of a great importance since the leaching content of iron species was very small in spite of the initial acidic pH of the used clays, as it was reported in the literature [21,22]. Thus, PAA conversion under our catalytic system could be regarded as an ecofriendly process for the synthesis of new compounds with high biologically added values.
Unexpectedly, TOC values remained quite constant during the catalytic PAA conversion using either the KSF or KSF/0 catalyst, which indicates a low mineralization phenomenon (<10%).
3.4 Antioxidant activity evaluation of the newly synthesized p-HPAA and 3,4-DHPAA mixture
In order to evaluate the antioxidant properties of the newly synthesized phenolic compounds, we first examined the DPPH radical scavenging assay performed on reaction aliquots withdrawn at regular intervals (Fig. 5). Samples prepared by both KSF or KSF/0 showed similar antioxidant activities, which exceeded BHA activity (94.7%). Regarding the hydroxyl substituent type, hydroxyl groups at the ortho-position of the PAA ring have considerably enhanced the radical scavenging efficiency of the mixture reaction, corresponding to high yield conversion of PAA to 3,4-DHPAA. This newly synthesized ortho-diphenolic compound has been largely described for its biological activities such as antioxidant and antimicrobial ones [19]. The higher antioxidant activity observed even after 2 h of the reaction may be explained by a synergistic effect resulting from the interaction of p-HPAA and 3,4-DHPAA.
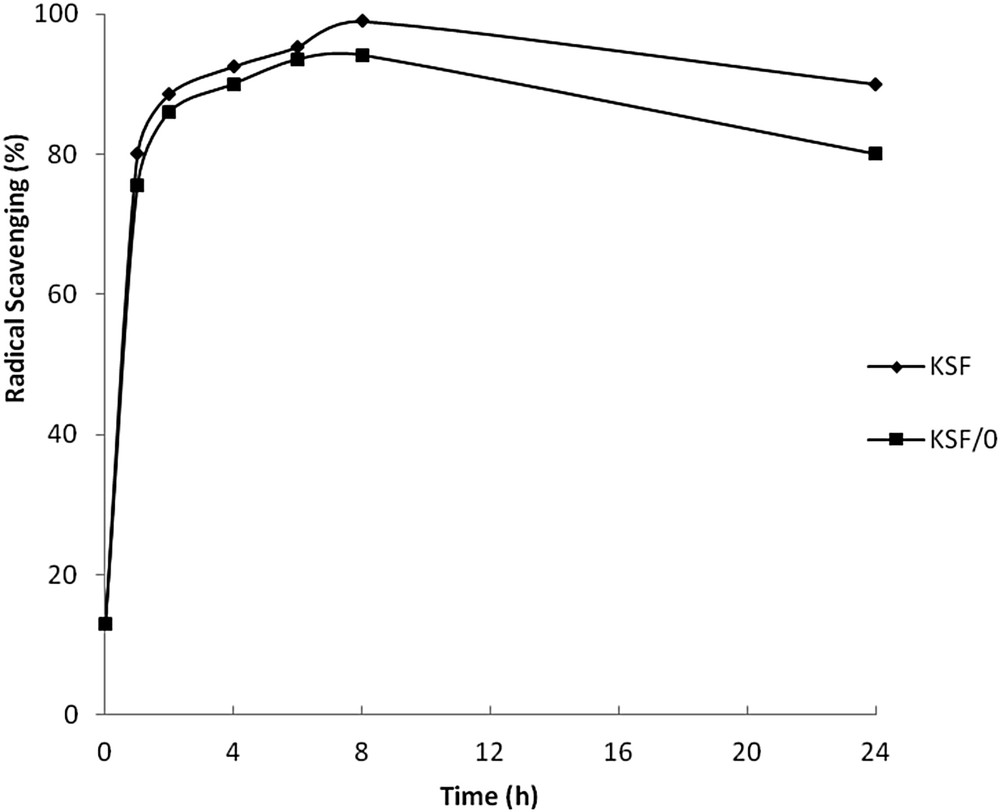
Free radical scavenging activity of the ortho-diphenolic compound during PAA oxidation.
4 Conclusion
As a conclusion, the method described here for the chemical synthesis of p-hydroxyphenyl acetic acid and 3,4-dihydroxyphenyl acetic acid exhibited a promising alternative to other previously published methods dealing with unnatural antioxidant invention. To our knowledge, this is the first study showing the ability to synthesize 3,4-dihydroxyphenyl acetic acid, a high-added-value phenolic compound, by hydrogen peroxide catalytic oxidation of phenylacetic acid using acid-activated clays. This is a very simple, mild, cheap and fast method for high amounts of 3,4-DHPAA (35%) recovery with no mineralization taking-off. Besides, it could be considered as an ecofriendly chemical approach since it does not use toxic reagents without significant iron catalyst leaching. At the end, catalyst particles can be removed by simple filtration and the target compound could be purified to obtain pure antioxidant in huge amounts. Finally, this process may prove its usefulness not only for laboratory applications but also for potential industrial ones, such as cosmetic and food industries.
Acknowledgement
The authors would like to thank the Ministry of Higher Education and Scientific Research of Tunisia (Contract programme LR14ES08), for the support of this research and for giving us the opportunity to work on it. We would like to appreciate Dr Mourad Jridi's help on the antioxidant activity.