1 Introduction
Room temperature ionic liquids (ILs), also called simply ILs, are substances formed only by ions and having melting temperatures less than 100 °C [1]. ILs have been a matter of studies for the past 25 years and new aspects and applications appear every day in the open literature. The main interest has been on their unique characteristics as liquids, and therefore solid–liquid transition properties have been a matter of several studies, mainly the melting temperature. Other melting properties such as glass transition temperature, enthalpy of fusion, and entropy of fusion have received less attention. These melting properties, the errors in the published data, the difficulties for their experimental determination, and mainly the possibilities of correlating and predicting melting properties are analyzed in this article.
There are difficulties for accurately measuring melting properties, difficulties that have been recognized and discussed by several authors. Holbrey and Rogers [2] presented a good summary of the main problems that present the experimental determination of melting properties of ILs. These are some of them: (1) Usually, cooling from the liquid state causes glass formation at low temperatures, because solidification kinetics are slow; (2) on cooling from the liquid, the low-temperature region is not usually bounded by the phase diagram liquidus line. It is extended down to a lower temperature limit imposed by the glass transition temperature; and (3) in some cases, there is evidence of multiple solid–solid transitions, either crystal–crystal polymorphism or formation of plastic crystal phases. The authors also give some recommendations on how to obtain good data: (1) The most efficient method for measuring the transition temperatures is differential scanning calorimetry; (2) thermodynamic data must be collected in heating mode to obtain reproducible results; and (3) long equilibration times, with small samples that allow rapid cooling, are needed to quench nonequilibrium states in mixtures and to obtain reliable transition data. It must also be considered that melting properties are influenced by the charge distribution on the ions, hydrogen-bonding ability, symmetry of the ions, and van der Waals interactions, but the presence of impurities in the samples and even confusion between melting and glass transition produce wrong data and add more difficulties to the development of correlating and predicting methods for estimating melting properties [3].
Also Preiss et al. [4] discuss the general reliability and reproducibility of the experimental data published. Aspects such as purity, phase behavior, disorder, and thermal history of a given substance, experimental mistakes, systematic errors, and lack of conventions to define melting properties are well analyzed. In particular, the authors discussed the presence of polymorphism (existence of more than one form or crystal structure) and conclude that “we think that the existence of polymorphs poses the most serious limitation on developing a prediction model.” In fact, melting properties might be influenced by the presence of solid–solid phase transitions, but more accurate data are needed to obtain a final conclusive answer to this phenomenon.
Data on melting properties have been presented by several authors, but in many cases agreement between values is poor. It seems that the difficulties mentioned above have surpassed the recommendations given by Holbrey and Rogers [2] and other authors. In fact, an approximate estimate of data indicates that for ILs there must be 1600 data for melting temperature, 800 data for glass transition temperature, 200 data for enthalpy of melting, and 50 data for entropy of melting available in the open literature. For some ILs several values of a melting property have been reported. For some cases, these values tremendously differ from one source to another. Aparicio et al. [5] analyzed published thermophysical properties data for ILs until 2010, including melting temperature, and concluded that it was necessary to perform further systematic thermophysical studies for the development of reference data sets. In a study, Valderrama [6] discussed several aspects related to available data and models presented in the literature to estimate the melting temperature of ILs.
The modeling of melting properties of ILs is perhaps one of the most challenging tasks. There are so many factors and phenomena occurring during transition from solid to liquid or vice versa that taking into account all factors in a single model is almost impossible. There are many studies explaining why a given IL or a group of ILs behave in a certain manner to find immediately several exceptions to such explanations. Preiss et al. [7] indicate that a group contribution method to estimate melting properties is not easy to adapt for ILs in which charges, ambiguous bonding situations, and the presence of inorganic elements affect the transition process in many different ways. In fact, there are much more than grouping groups as simply done for organic substances.
Despite all the problems mentioned previously, some proposals are found in the literature to estimate melting properties, mainly the melting temperature. A good review on the different methods for estimating thermophysical properties of ILs has been performed by Coutinho et al. [8]. The authors dedicate an important section of the article to the different attempts made by several authors presented in the literature to estimate melting properties. The authors discuss the different modeling approaches and make suggestions about what are the best methodologies for the prediction of each property. They state that because most of the literature analyzed is from about 2007 until 2011, this field of modeling and predicting IL properties is still in its infancy. After 5 years from that review, no major progress has been made, but several researchers are attempting different approaches to estimate phase transition properties of ILs. Molecular dynamics simulation has been used by Zhang and Maginn [9], Aguirre et al. [10] used group contribution methods, and Eike et al. [11] and Farahani et al. [12] used computational chemistry. In other reports, Zhu et al. [13] and Bai et al. [14] used quantitative structure–activity relationship models to estimate heat of fusion of several types of ILs, and Adamova et al. [15] analyzed the alternation effect in chemically homologous series.
Homology, homologous series, and homologous compounds are concepts derived from the word homologue, a term “used to describe a compound belonging to a series of compounds differing from each other by a repeating unit” [16]. This concept of chemical homology has been used by Valderrama and Rojas [17] for data selection and estimation of the normal melting temperature of ILs. According to the authors, the estimation method gives reasonable values of the normal melting temperature for engineering calculations. However, despite that most of the existing data published until 2012 was used, the method gave positive results for a reduced number of IL families only.
2 Data on melting properties
Data on melting properties of ILs are scarcer than for other physical and physicochemical properties, melting temperature being the most studied of these melting properties. In general, data are ambiguous, not very well defined and, as mentioned previously, values may tremendously differ from one another. Most reports including correlations with the use of literature data do not explain the reasons for choosing one value or another, although the literature sources are provided. Differences up to 70 K for the melting temperature (25% with respect to the lowest reported value), 20 K for glass transition temperatures, and 10 kcal mol−1 for the heat of fusion (100% with respect to the lowest reported value) are found for some ILs, which are not acceptable for producing good and general correlating and predicting models for these properties [6].
It should also be mentioned that data compilations and databases of melting properties are not clear enough about the accuracy of the values that are reported. In some cases, uncertain values are given [18–21]. Valderrama [6] analyzed the information provided by the NIST database for the melting temperature of ILs concluding that the huge differences between reported melting temperature values are not acceptable for producing good and general correlating and predicting models for the melting temperature. The same can be said about other melting properties such as glass transition temperature, heat of melting, and entropy of melting, as shown in Table 1. This table shows selected values of melting properties in which the magnitude of the discrepancies between reported values is listed. The discrepancies indicate that data analysis is required before formulating models or even for the direct use of the data in process design, process simulation, or process optimization.
Deviations of experimental melting data published by different authors for some ionic liquids.
Melting temperature | ||||
IL (full name) | IL (Abbreviation) | Tm (K) | %ΔD | |%ΔT|m |
1-Butyl-3-methylimidazolium chloride | [bmim][Cl] | 314 | – | – |
338 | 24 | 7.6 | ||
342 | 28 | 8.9 | ||
343 | 29 | 9.2 | ||
1-Butyl-3-methylimidazolium hexafluorophosphate | [bmim][PF6] | 212 | – | – |
265 | 53 | 25.0 | ||
277 | 65 | 30.7 | ||
284 | 72 | 34.0 | ||
1-Decyl-3-methylimidazolium tetrafluoroborate | [C10mim][BF4] | 196 | – | |
248 | 52 | 26.5 | ||
269 | 73 | 37.2 | ||
Glass transition temperature | Tg (K) | %D | |%ΔD|m | |
1-Butyl-3-methylimidazolium tetrafluoroborate | [bmim][BF4] | 176a | – | – |
186a | 10 | ≈5.5 | ||
188a | 12 | ≈6.8 | ||
190a | 14 | ≈8 | ||
1-Octyl-3-methylimidazolium hexafluorophosphate | [Omim][PhCH2CH2mim] | 191 | – | – |
202 | 11 | ≈5.8 | ||
Heat of melting | ΔHm | %ΔD | |%ΔD|m | |
1-Butyl-3-methylimidazolium bis(trifluoromethylsulfonyl)imide | [C2CF2CF2Him][bmim] | 22,430b | – | – |
24,530b | 2100 | 9.4 | ||
28,430b | 6000 | 27 | ||
1-Butyl-3-methylimidazolium hexafluorophosphate | [bmim][PF6] | 9210a,b | – | – |
13,300b | 4090 | ≈44 | ||
19,600b | 10,390 | ≈113 | ||
19,910a,b | 10,700 | ≈116 | ||
1-Ethyl-3-methylimidazolium tetrafluoroborate | [emim][BF4] | 9542b | – | – |
10,610b | 1068 | ≈11 | ||
12,512b | 2969 | ≈31 | ||
22,000a | 12,433 | ≈130 | ||
22,767b | 13,225 | ≈139 |
3 Homologous series and homologous compounds
In chemistry, a group or family is a column of elements in the periodic table of elements. Elements in the same group show some defined patterns for some atomic and chemical characteristics (atomic radius, ionization energy, and electronegativity). From top to bottom in a group, the atomic radii increases, and ionization energy and electronegativity decrease. Some few exceptions exist, but they do not alter this general pattern. Also, similar behavior is observed in horizontal sequences of the periodic system (known as periods), but groups usually have more significant periodic trends than periods [22]. This concept of forming families of elements in which certain characteristics and properties follow a defined pattern has been extended to compounds using the concept of homologue. What is not well defined for different type of substances is the extension of the family for which the homologue concept could be applied. For instance, among organic substances, the n-alkanes, the n-alkanols, or the organic acids form families. Among inorganic salts, classified by the anion, chlorides, bromides, carbonates, or sulfates form families and classified by the cation, calcium, magnesium, iron, or sodium salts form families. Among ILs, examples of families can be Cn-3-methylimidazolium-hexafluorophosphate [Cn-mim][PF6] or Cn-isoquinolinium-bis(pentafluoroethylsulfonyl)imide [Cn-isoq][BETI].
3.1 Homology and molten salts
In the case of salts, families could be formed by the cation and the anion. Thus, chloride salts or the bromide salts should form families. If melting properties for these groups of substances are plotted as a function of the molecular mass, it can be observed that some scattering and apparently homology does not work, as shown in Fig. 1. What is happening here is that anion and cation have much different effect depending on the combination of cation and anion, meaning which cation forms a salt with which anion. Therefore, it is necessary to better define the family, which in this case cannot be as big as all chlorides or all bromides. Fig. 2 shows the same chloride families but now forming compounds with the alkaline group of the periodic system only. The homology concept seems to work again as shown in the figure for the melting temperature and the heat of melting. In this figure, beryllium salts are not considered because of its known anomalies mainly owing to its large charge to size ratio compared with the other members of the group (Mg, Ca, Sr, and Ba) [23].

Melting properties of chloride salts with different cations as a function of the molecular mass of the salts.

Melting properties of chloride salts formed with alkali metal elements only as cations.
On the basis of the concept of homologous series, the concept of homologous compounds seems more interesting in some applications. Karapetyants [24] used this concept in what he called “comparative calculations” to determine several properties of solids. What the author actually did was related a given property of two types of compounds having either the same cation or the same anion. In addition, examples for the entropy of formation and the Gibbs free energy of formation were given. The concepts of Karapetyants are extended to melting properties of salts, as presented in Figs. 3 and 4, for the melting temperature and the heat of melting. In Fig. 3, homology is applied by comparing bromine salts with properties of similar fluorine salts. As observed, the data on melting temperature show some pattern but for the heat of melting dispersion is higher. If the families are reduced to fluorine and bromine salt having as cations elements of the second column of the periodic system the Fig. 4 is found, in which a more uniform pattern is observed.

Melting properties of different types of bromide versus fluoride salts showing compound homology.

Melting properties of bromide versus fluoride salts showing compound homology for cations with valence +2.
3.2 Homology and organic compounds
As observed in the preceding analysis, families of salts are not long enough to observe all characteristics and patterns that could be found in bigger families. Organic compound, however, may form longer families such as the n-alkanes, the n-alkanols, or the organic acids. In fact, these families show an acceptable smooth pattern as the carbon number increases for several properties [22]. But even for the n-alkanes or the n-alkanols, solid–liquid transition properties show some peculiarities that need to be discussed. Fig. 5 shows the melting temperature, the heat of melting, and the entropy of melting for the n-alkane family. As observed in the figure, the peculiar behavior shown by odd-carbon number and even-carbon number compounds, known for many years as “alternation effect”, is observed. For the melting temperature a ladder-type line is obtained, although for the melting enthalpy and entropy the steps are much more notorious presenting a saw tooth shape.

Change in several properties of n-alkanes as a function of the number of carbon in the n-alkane chain: (▪) normal melting entropy (kJ mol−1 K−1) × 102; (▴) normal melting temperature (K); (•) normal heat of melting (kJ mol−1) × 10. Data used were taken from Daubert et al. [25].
Baeyer in 1877 [26] first observed the alternation phenomenon for a family of n-alkanes (n < 10) with a triclinic crystal system. Fig. 6 shows the melting temperature versus the number of carbon atoms in the alkyl chain for n-alkyl cyanobiphenyl as observed by Baeyer [26]. In addition, Caspari [27] was probably the first author to relate the alternation phenomenon to crystal structure. Caspari wrote, “Ultimately the melting point must depend on the ease with which molecules are torn out of the crystal lattice, and it is plausible to suppose that the double cells of the odd carbon acids (having relatively lower m. p.'s) are less stable in this respect than the more compact cells of the even-carbon acids.”
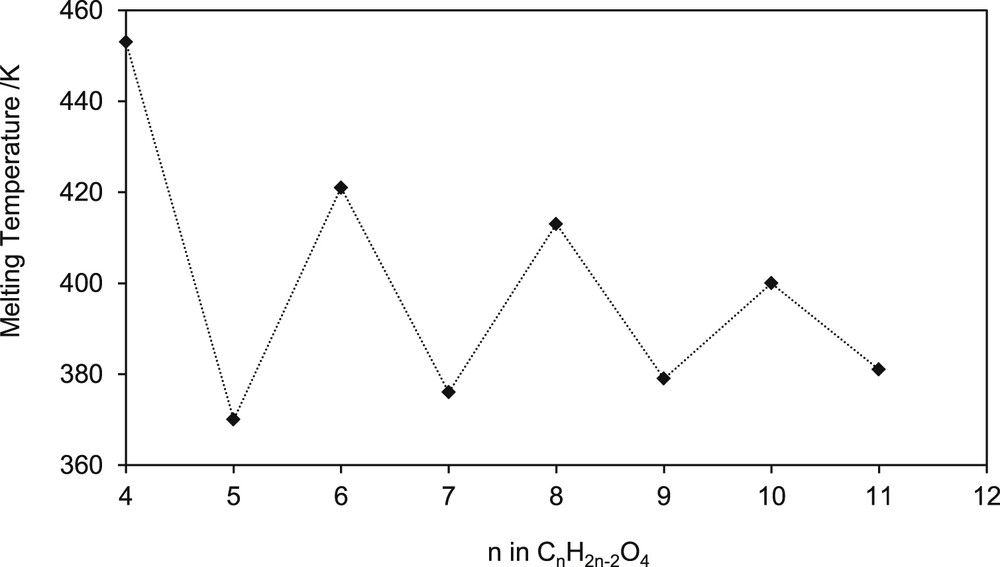
Melting temperature versus the number of carbon atoms in the alkyl chain for n-alkyl cyanobiphenyl. Data used were taken from Baeyer [26].
The alternation effect causes an additional problem for most property estimation methods (group contribution methods and modern computational chemistry calculations), in which the value of a property is obtained by summing up the contributions of each group forming the molecule. Most models being nowadays proposed do not consider these effects. One attempt to consider the odd–even effect on model proposals for estimating the melting temperature has been made by de la Rama et al. [28], who studied silver alkanethiolates forming lamellar structures. The authors state that constant parameters in a model cannot explain the odd–even effect that appears in some situations.
This alternation effect clearly affects homology, especially homologous family behavior. One could expect that the effect could be reduced for the homologous compound concept because in this method properties of different families are compared and deviations could cancel. This hypothesis, however, must be checked. Fig. 7(a–c) presents melting properties for the family of n-alkanes compared with the properties of n-alkanols. For glass transition temperature the example is given for two families of polymers (Fig. 7d), because n-alkanes and n-alkanols do not have glass transition temperature. As observed in these figures, the alternation phenomenon has more effect on the heat of melting and the entropy of melting in which two different lines are obtained when odd-carbon and even-carbon compounds are compared.

The concept of homologous compounds applied to melting properties of organic substances.
The information available in the literature and discussed in the preceding sections indicates that the homologous compound concept could be applied to ionic liquids, with some restrictions. In a previous work, Valderrama and Rojas [17] applied the homology concept with the aim of detecting erroneous data on melting temperature of ILs. They used some limited number of data and hexafluorophosphate ILs, [X][PF6], as reference fluids for the correlations. In the present work, the concept of homologous compounds discussed previously for molten salts, polymers, and organic substances is extended to ILs. Following the concept, one should expect that the properties of a family such as [Cn-mim][PF6] and the properties of another family such as [Cn-mim][BF4] follow a defined pattern. However, this is not necessarily true and must be demonstrated.
4 Extension to ILs
To apply the concept of homology to ILs, various restrictions were set and imposed by the authors [17]: (1) Several points must be available to perform homology (four or more); (2) if there are enough data available and a tendency line is not, that case is dismissed; (3) if several points are available and most of the number of points follow a clear tendency, those data are considered as valid data; (4) if the difference in melting temperatures of all ILs in the family is below experimental uncertainty, then the groups are accepted as the compounds are considered as homologous compounds; and (5) after one data point is accepted by homology that value is considered correct for all other homologous cases.
The first restriction is the one that limits the applicability of the homology concept to better analyze data on melting properties of ILs. As stated previously even for the melting temperature, the most studied of these properties, data are in some cases not enough to apply with confidence the homology concept. If one adds the great differences in the reported values, the problem becomes more difficult to solve, but still useful as shown in this article.
4.1 Homologous families and homologous compounds
Because of the especial characteristics and especial behavior mentioned previously, the homology is more complex for ILs, but we believe that is a matter of a good definition of the compounds to be homologated. Most probably, because of the especial effects of the type of cation and anion, the structure, the even–odd behavior, and other physicochemical characteristics, families will be shorter, but still the method can be useful for those cases in which experimental data are difficult or impossible to obtain.
In addition, the melting temperature curve for ILs in a homologous series shows another peculiarity for high carbon number in the alkyl chain, as seen in Fig. 8 for n > 12. This figure shows the variation in the melting temperature of [Cn-mim][BF4] as a function of the length of the alkyl chain. An interesting feature of the curve for the IL [Cn-mim][BF4] is the appearance of liquid crystalline phases as the chain gets longer (n > 12). This especial behavior has been seen in different ways. Rooney and Seddon [30] explain this phenomenon considering the isotropic behavior of salts with short alkyl chains (n = 2–10) enantiotropic behavior of longer chains (n > 12). However, Balaban et al. [31] explain this behavior as being a consequence of interpenetrating sublattices that are formed, with anions on one sublattice and cations on the other and each sublattice having different behavior, and stated that “this situation would then lead to a superionic material.” Biswas et al. [32] analyzed imidazolium-based ILs with different fatty acid anions and found that the length of the alkyl chain of the carboxylate anion in ILs influences the phase transition temperature of their crystalline solid phase and the mesophase stability.

Melting temperature Tm for the homologous series [Cn-mim][BF4]. Melting transitions are as follows: (▪) crystal–liquid; (□) glass–liquid; (•) crystal–liquid crystal. Data used were taken from Wypych [29].
In the present study, there are some cases in which homology did not work. This means that the melting temperature or the glass transition temperature data show some dispersion and with the information available it is not possible to find out which data are wrong. Those cases in which homology cannot be applied for the melting temperature have been discussed elsewhere [6,17]. In one of the articles, it was concluded that “according to the concept of chemical homology those compounds that do not follow a common smooth behavior could be in error.” But to apply this statement a large amount of data should be available. No applications of the homology concept to other melting properties, such as glass transition temperature or heat of melting, have been found in the literature.
Adamova et al. [33] determined melting temperature and heat of fusion for the family tetralkylphosphonium chlorides, [P8,8,8,n][Cl]. From Adamova's data, the entropy of fusion was determined as ΔSm = ΔHm/Tm for the five ILs in the series for which data were available. The values are shown in Fig. 9. The authors, referring to the family of compounds studied, from n = 1 to n = 14, state that “most of the studied compounds simply form a glass at low temperatures with no apparent freezing or melting transitions.” In fact, this happens for n = 3–10. Thus, the reported data on melting entropy are uncertain. Some of the values could belong to glass–glass transition.

Entropy of melting of alkyltrioctylphosphonium chloride (kJ kg−1 K−1).
4.2 Correlation and prediction
As explained earlier, the authors have previously used the compound homology concept for estimating the melting temperature but not for other melting properties [17]. The authors applied compound homology for all ILs for which experimental data were available and selected those that follow a certain pattern in all possible combinations. However, the study was done for a limited number of ILs and few homology cases could be done. Also, the authors used [PF6] ILs as reference fluids to estimate the melting temperature of other ILs.
In this work, the homologous compound concept is applied but comparison is not limited to a single IL. Fig. 10 presents the melting properties of selected ILs for which homology works in an acceptable way (low dispersion of data) for some ILs for which data are available. This figure shows melting temperature for [X][PF6] versus [X][Cl] and [X][BTI] versus [X][Cl], glass transition temperature for [X][Br] versus [X][S8] and [X][BF4] versus [X][DCA], and heat of melting for [X][BTI] versus [X][Cl] and [X][Br] versus [X][Cl]. The only two cases for which homology could be applied for the melting enthalpy are shown in Fig. 10 (e and f). In addition, in these two cases a reduced number of data were available. For the entropy of melting there were not enough data to perform homology.

Homology for melting properties of selected ionic liquids.
According to the aforementioned discussion and the analysis done using most of the existing data on melting properties, the homologous compound concept seems to be acceptable for its extension to the correlation and prediction of melting properties of ILs having homologous cation. For instance [bmim][Cl] and [bmim][Br] or [emim][Cl] and [emim][Br] are homologous compounds, and each pair has a common cation. Thus, the properties of the families [Xmim][Y] and [Xmim][Z] are related and follow a certain defined pattern, usually linear. Following previous studies [17,34], the melting properties of ILs [X][Y] can be related to the properties of ILs [X][Z] as follows:
(1) |
(2) |
(3) |
Tables 2 and 3 present values of the parameters am, bm, ag, bg, ah, and bh in Eqs. (1)–(3) for estimating melting properties of an IL of the type [X][Y] by knowing the melting property of an IL [X][Z].
Models for estimating the melting temperature of several ionic liquids of the type [X][Y] by knowing the melting temperature Tm of an [X][Z] ionic liquid.
Type of ILs | Model | Model for Tm for [X][Y] | N | R2 | %ΔD | |%ΔD| | |%ΔD|m |
Ammonium | TM1 | Tm[X][BTI] = 0.911 × Tm[X][BF4] − 124.1 | 4 | 0.91 | 0.0 | 1.8 | 2.6 |
Triazolium | TM2 | Tm[X][I] = 1.130 × Tm[X][BTI] + 42.234 | 6 | 0.89 | 0.1 | 3.2 | 4.7 |
Imidazolium | |||||||
Pyrrolinium | |||||||
Imidazolium | TM3 | Tm[X][PF6] = 1.245 × Tm[X][Br] − 80.641 | 5 | 0.93 | 1.3 | 3.3 | 4.4 |
Triazolium | |||||||
Ammonium | |||||||
Morpholinium | |||||||
Imidazolium | TM4 | Tm[X][TFO] = 1.582 × Tm[X][BTI] − 153.16 | 10 | 0.92 | 0.9 | 3.8 | 11.9 |
Benzotriazolium | |||||||
Pyridinium | |||||||
Thiazolium | TM5 | Tm[X][PF6] = 1.057 × Tm[X][BF4] + 7.17 | 20 | 0.98 | 0.2 | 3.9 | 8.3 |
Imidazolium | |||||||
Benzotriazolium | |||||||
Ammonium | |||||||
Pyridinium | |||||||
Imidazolium | TM6 | Tm[X][Cl] = 1.508 × Tm[X][BF4] − 224.8 | 10 | 0.86 | 0.6 | 6.3 | 13.9 |
Ammonium | |||||||
Pyridinium | |||||||
Thiazolium | TM7 | Tm[X][PF6] = 0.660 × [I] + 133.4 | 4 | 0.97 | 0.7 | 2.3 | 3.7 |
Imidazolium | |||||||
Ammonium | |||||||
Imidazolium | TM8 | Tm[X][TFPB] = 0.535 × Tm[X][PF6] + 240.5 | 4 | 0.99 | 0.0 | 0.2 | 0.3 |
Pyridinium | |||||||
Imidazolium | TM9 | Tm[X][TFO] = 2.054 × Tm[X][NO3] − 342.8 | 4 | 0.99 | 0.0 | 0.8 | 1.3 |
Morpholinium | |||||||
Pyrrolidinium | TM10 | Tm[X][C4F9BF3] = 0.845 × Tm[X][[n-C3F7BF3] + 55.6 | 9 | 0.77 | 0.22 | 2.93 | 8.99 |
Piperidinium | |||||||
Imidazolium | |||||||
Morpholinium | |||||||
Ammonium | |||||||
Imidazolium | TM11 | Tm[X][Cl] = 8.98 × Tm[X][BTI] − 2189.3 | 4 | 0.99 | 0.1 | 1.5 | 2.8 |
Models for estimating the other transition properties (glass transition temperature and heat of melting).
Types of ILs | Model | Model for a property for [X][Y] | N | R2 | %ΔD | |%ΔD| | |%ΔD|m |
Imidazolium | TG1 | Tg[X][DCA] = −0.258 × Tg[X][Cl] + 231.3 | 3 | 0.973 | 0.0 | 0.4 | 0.7 |
Imidazolium | TG2 | Tg[X][BTI] = 0.373 × Tg[X][BF4] + 115.8 | 6 | 0.573 | −0.2 | 0.74 | 1.8 |
Imidazolium | TG3 | Tg[X][BF4] = 0.697 × Tg[X][DCA] + 62.1 | 3 | 0.364 | −1.2 | 3.07 | 4.8 |
Pyridinium & | TG4 | Tg[X][Br] = 2.533 × Tg[X][BTI] − 244.1 | 4 | 0.984 | 0.0 | 1.1 | 2.2 |
Pyrrolidinium | |||||||
Pyridinium | TG5 | Tg[X][Br] = 2.642 × Tg[S8] − 465.0 | 3 | 0.999 | 0.0 | 0.0 | 0.0 |
Imidazolium | TG6 | Tg[X][BF4] = 0.747 × Tg[PF6] + 41.9 | 7 | 0.594 | 3.8 | 4.6 | 7.6 |
Imidazolium | DH1 | ΔHm[X][BTI] = 0.389 × ΔHm[X][Cl] + 15.62 | 3 | 0.992 | 0.00 | 0.50 | 0.9 |
Imidazolium | DH2 | ΔHm[X][Br] = 0.716 × ΔHm[X][Cl] + 6.945 | 5 | 0.975 | 0.16 | 2.6 | 5.0 |
The predictive capabilities of the models were also tested. This was done by estimating melting property of ILs that were not considered in obtaining the correlations shown in Tables 2 and 3. Table 4 shows predicted values of melting temperature and glass transition temperature of selected ILs. For instance, for the IL [dmprim][PF6] (not used in obtaining the correlation in Table 2), the melting temperature is predicted as 439 K using [dmprim][I] as reference fluid, whereas a literature value is 454 K (3.4% deviation). Also for the IL [hpmim][BTI], not used in obtaining the correlation in Table 3, the predicted glass transition temperature is 187.7 while a literature value is 188.2 K.
Predicted values of melting properties for some ionic liquids using the models presented in Tables 2 and 3.
Properties | Model | Reference IL (X) | Predicting IL (Y) | Lit. value | Pred. value | %ΔD |
Tm | TM3 | [N11(4-Me-CH2Ph)2][Br] | [N11(4-Me-CH2Ph)2][PF6] | 490.1 | 510.9 | 4.2 |
[N1144][Br] | [N1144][PF6] | 436.7 | 454.7 | 4.1 | ||
TM4 | [C4C2im][BTI] | [C4C2im][TFO] | 275.2 | 266.4 | −3.2 | |
[C8F17(CH2)2bipy][BTI] | [C8F17(CH2)2bipy][TFO] | 382.2 | 427.8 | 12.0 | ||
TM5 | [C14H19N2F4OB][BF4] | [C14H19N2F4OB][PF6] | 315.2 | 340.1 | 7.9 | |
[C15H30N2F4O3PB][BF4] | [C15H30N2F4O3PB][PF6] | 190.6 | 208.5 | 9.4 | ||
[C8H12N3F4B][BF4] | [C8H12N3F4B][PF6] | 436.1 | 467.9 | 7.3 | ||
TM7 | [dmprim][I] | [dmprim][PF6] | 439.2 | 454.3 | 3.4 | |
TM10 | [C10H16NF10O2B][n-C3F7BF3] | [C10H16NF10O2B][n-C4F9BF3] | 291.2 | 295.0 | 1.3 | |
[C13H22NF10B][n-C3F7BF3] | [C13H22NF10B][n-C4F9BF3] | 365.2 | 355.1 | −2.8 | ||
[C12H22NF10OB][n-C3F7BF3] | [C12H22NF10OB][n-C4F9BF3] | 284.2 | 291.6 | 2.6 | ||
Tg | TG2 | [hpmim][BF4] | [hpmim][BTI] | 188.2 | 187.7 | −0.2 |
TG3 | [C10mim][DCA] | [C10mim][BF4] | 248.5 | 236.6 | −4.8 | |
TG6 | [hpmim][PF6] | [hpmim][BF4] | 189.2 | 183.1 | −3.2 | |
[pmim][PF6] | [pmim][BF4] | 193.2 | 186.1 | −3.7 |
There are cases for which the homology compound concept does not apply. It seems that the change in cation and ion has different effects depending on the type of anion and cation. More information is needed about the real mechanism that produces the extraordinary characteristics of ILs in which the types of anion and cation are of special importance. When homology is done, all the effects of physical and chemical interactions are considered as a whole, but this is not enough.
For instance when a –CH2 group is added to a given n-alkane or n-alkanol to produce the next compound in the family chain, the effect in both families on a given property seems to be proportional to the chain length or the molecular mass. However, in the case of salts and ILs the addition of a –CH2 group to form the next compound in the family chain causes different effects if this occurs, for example, in the family [Cn-min][BF4] or [Cn-min][BTI]. If this behavior is complex and difficult to explain, the situation is worse when modeling is attempted.
As mentioned previously, the several attempts made by authors published in the literature to correlate and predict melting properties do not work in a general accurate way not only because data could be in error but also because most models do not take into account all the factors that may affect melting properties, factors that are anyways uncertain. According to Coutinho et al. [8], “important information concerning the nature of the solid phase itself seems to be missing in the models.” This means that most models do not take into account the formation of different crystalline solid phases with their own characteristics, different, for instance, for homologous compounds such as [C2-min][BF4] and [C2-min][BTI].
5 Conclusions
The homology concept applied to families of ILs seems to be a reasonable method for predicting melting properties of ILs. Care must be taken when choosing the family to be homologated because of the presence of chemical “anomalies” of some cations and anions forming the IL.
The compound homology concept seems to be more appropriate than homologous families but a minimum number of points must be available. At least three pairs of data for each pair of compounds are necessary.
For some ILs, values of a melting property reported in the literature tremendously differ from one source to another, so more accurate data are needed to formulate new models.
Group contribution methods to estimate melting properties of ILs must be adapted to consider charges, ambiguous bonding situations, and the presence of organic and inorganic elements. New models for solid–liquid transition properties should include factors related to the nature of the solid phase.
Acknowledgments
This work was supported by the National Council for Scientific and Technological Research (CONICYT), through the research grant FONDECYT 1120162. The authors thank the Center for Technological Information of La Serena, Chile, for computer support and the Direction of Research of the University of La Serena for permanent support and for an especial graduate fellowship for one of the authors (R.A.C.).
Notation
Symbolsam, bm
parameters in equation for the melting temperature, Tm[X][Y]
ag, bgparameters in equation for the glass transition temperature, Tg[X][Y]
ah, bhparameters in equation for the heat of melting ΔHm[X][Y]
%ΔDrelative % deviation between correlated and literature data
∣%ΔD∣absolute % deviation between correlated and literature data
∣%ΔD∣maxmaximum absolute % deviation between correlated and literature data
ΔHmheat of melting
ΔHm[X][Y]heat of melting of ionic liquid [X][Y]
R2coefficient of determination for linear correlations
ΔSmentropy of melting
Tmmelting temperature
Tm[X][Y]melting temperature of ionic liquid [X][Y]
Tgglass transition temperature
Tg[X][Y]glass transition temperature of ionic liquid [X][Y]
[X][Y]ionic liquids having [X] as the cation and [Y] as the anion
[X][Z]ionic liquids having [X] as the cation and [Z] as the anion
AbbreviationsIL
ionic liquid
Lit.literature
Pred.predicted