1 Introduction
Protection of functional groups is often necessary during a multistep organic synthesis, so the development of economical and efficient methods for this purpose is very important. Hydroxyl and amino groups in alcohols, phenols and amines are often present in organic molecules, and their protection is an important process during organic reactions. Among various procedures for the protection of hydroxyl and amino groups, acetylation with acetic anhydride or acetyl chloride in the presence of an acidic or basic catalyst is an efficient and common route due to the stability of the products in acidic media and easy introduction and removal of the acetyl group. For this purpose many Brönsted and Lewis acidic catalysts have been used [1–6]. Although these methods are improved, most of them suffer from disadvantages such as long reaction times, use of organic solvents, expensive catalysts, use of excess amounts of the acylating agents and harsh reaction conditions. Therefore there is still a need to find an alternative method which might be mild and catalytically and economically efficient.
In recent years, synthesis of nanocrystalline metal oxides has attracted much attention due to their unusual catalytic properties compared to bulk metals [7]. Among various metal oxides, TiO2 nanoparticles is one of the most utilized ones which have been widely investigated as the catalyst, pigment, food coloring substance, etc [8–9]. Preparation of the non-agglomerated TiO2 nanoparticles, with a narrow size distribution in anatase form, is very important to achieve higher catalytic activity and more readily handling than the commercial TiO2 [10–11]. On the basis of this subject, the synthesis of TiO2 over a silica support was explored as a useful way for preventing the agglomeration and formation of dispersed TiO2 aggregates [12]. Siliceous materials are desirable because they are chemically inert and have a high specific surface area and thermal stability.
Rice husk, the outer covering of rice grains, is one of the main agricultural residues which is obtained during the milling process. Application of rice husk as an energy source for biomass power plants, rice mills and brick factories is increasing due to its high calorific power [13]. In this combustion, rice husk ash (RHA) is produced. RHA contains a considerable amount of amorphous silica up to 80% and small proportion of impurities such as K2O, Na2O and Fe2O3 [14].
In recent years, investigation on the application of TiO2-based reagents in organic reactions became an important part of our ongoing research program [15–17]. In continuation of these studies and because that RHA possesses high silica content, we were interested to investigate the possibility of the preparation of anatase-phase TiO2 over this reagent.
2 Experimental
2.1 General
Chemicals were purchased from Fluka, Merck, and Aldrich chemical companies. All yields refer to the isolated products. Products were characterized by comparison of their physical constants, IR and NMR spectroscopy with authentic samples and those reported in the literature. The purity determination of the substrate and reaction monitoring were accompanied by TLC on silicagel polygram SILG/UV 254 plates.
2.2 Instrumentation
The FT-IR spectra were run on a VERTEX 70 Brucker company (Germany). Scanning election microphotographs were obtained on a SEM-Philips XL30. X-ray diffraction (XRD) measurements were performed at room temperature on a Siemens D-500 X-ray diffractometer (Germany), using Ni-filtered CoKα radiation (λ = 0.15418 nm).
2.3 Preparation of RHA/TiO2 nanocomposites
1.0 g of rice husk ash (used in our previous report [18])in absolute ethanol (6 mL) was stirred for 30 min at room temperature. Then the required amount of titanium tetraisopropoxide (TTIP) (volume ratio TTIP:EtOH of 1:6) was added dropwise to the solution with stirring for 2 h. In continue, deionized water was slowly added to the resulting mixture (TTIP:water with a ratio of 1:60) and the stirring was maintained for 2 h to hydrolyze the remaining TTIP completely. The prepared material was separated by filtration, washed with water, and dried at 80 °C in an oven. Finally, the obtained solid was calcinated at 500 °C for 3 h to obtain RHA/TiO2 nonocomposites. [Caution: Throughout the subsequent discussion, the samples will be named by indicating the titania content and support, as RHA/TiO2(20%), RHA/TiO2(30%) and RHA/TiO2(50%).]
2.4 General procedure for acetylation with Ac2O catalyzed by RHA/TiO2(30%)
1 mmol of the substrate (alcohol, phenol or amine) was added to a mixture of RHA/TiO2(30%) (20 mg) and acetic anhydride (1.5 mmol per OH/NH2 group) and the resulting mixture was stirred at room temperature. After completion of the reaction (mentioned by TLC), dichloromethane (20 mL) was added and the catalyst was separated by filtration. The organic phase was washed with 10% aqueous solution of sodium bicarbonate (2 × 20 mL) and dried over Na2SO4. The solvent was removed under reduced pressure to afford the desired product in good to high yields. The spectral (IR, 1H and 13C NMR) data of new compounds are presented below:
Benzene-1,2,3-triyl triacetate: White solid; IR (neat) ν = 1765 cm−1; 1H NMR (CDCl3, 400 MHz): δ = 2.31 (s, 6H, CH3), 2.32 (s, 3H, CH3),7.14 (d, J = 8.2 Hz, 2H, ArH), 7.29 (dd, J = 8.3 and 0.8 Hz, 1H, ArH) ppm; 13C NMR (CDCl3, 100 MHz): δ = 20.6, 21.0, 121.1, 126.4, 135.1, 143.9, 167.4, 168.3 ppm.
N-(naphthalen-1-yl)acetamide: White solid; IR (neat) ν: 3295, 1635 cm−1; 1H NMR (CDCl3, 400 MHz): δ = 2.30 (s, 3H, CH3), 7.45–7.87 (m, 8H, ArH, NH) ppm; 13C NMR (CDCl3, 100 MHz): δ = 22.8, 118.5, 122.3, 122.8, 125.2, 126.1, 127.3, 127.8, 128.4, 128.5, 133.1, 169.9 ppm.
2.5 Catalyst characterization
2.5.1 FT-IR analysis
Fig. 1 shows the FT-IR spectra of nano-TiO2 (anatase), RHA and 20, 30 and 50 W% RHA/TiO2. In all cases, the peaks at 3400 and 1630 cm−1 are attributed to the stretching and bending modes of the hydroxyl groups of Si–OH or Ti–OH and the adsorbed water [19]. The strong peak at 1100 cm−1 and the peaks at 801 and 468 cm−1 are assigned to the asymmetric stretching, symmetric stretching and bending modes of Si–O–Si, respectively. Because the vibration modes of Ti–O–Ti lie in the range of 500–700 cm−1 (Fig. 1, spectrum of TiO2) [20], the peaks appearing at 500–700 cm−1 in the prepared samples can be attributed to Ti–O–Ti (Fig. 1, spectra of RHA/TiO2 nanocomposites). Increasing the intensity of these bands by increasing the titanium content of the samples can be described on the basis of this fact. Theoretically, the stretching vibration of Ti–O–Si appeared as a weak peak at 960 cm−1, whereas such a peak was not observed in the prepared samples. So, it could be resulted from the FT-IR spectra and there is no binding between TiO2 and SiO2, and titania is only embedded into the RHA matrix [10].

FT-IR spectra of RHA, TiO2 and various RHA/TiO2 nanocomposites.
2.5.2 Powder X-ray diffraction
Fig. 2 represents the X-ray diffraction (XRD) patterns of the RHA and RHA/TiO2 nanocomposites. The broad peak appeared around 2θ equal to 22 in the RHA pattern clearly indicating that the silica of rice husk ash is mainly in the amorphous form [21]. The peaks appearing around 2θ = 25.3, 37.8, 47.8, 54.1 and 62.3 in RHA/TiO2 nanocomposites are related to the (101), (004), (200), (105) and (211) reflections which indicate that the anatase phase is the only phase present in all the prepared materials [22–23]. The intensity of anatase peaks increased by increasing in the titania contents, however broadening of these peaks show that the nanocomposite particles have very small size. These observations strongly suggested that not only the RHA inhibited the formation of the rutile phase, but also inhibited growth of crystal grain of titan [24].
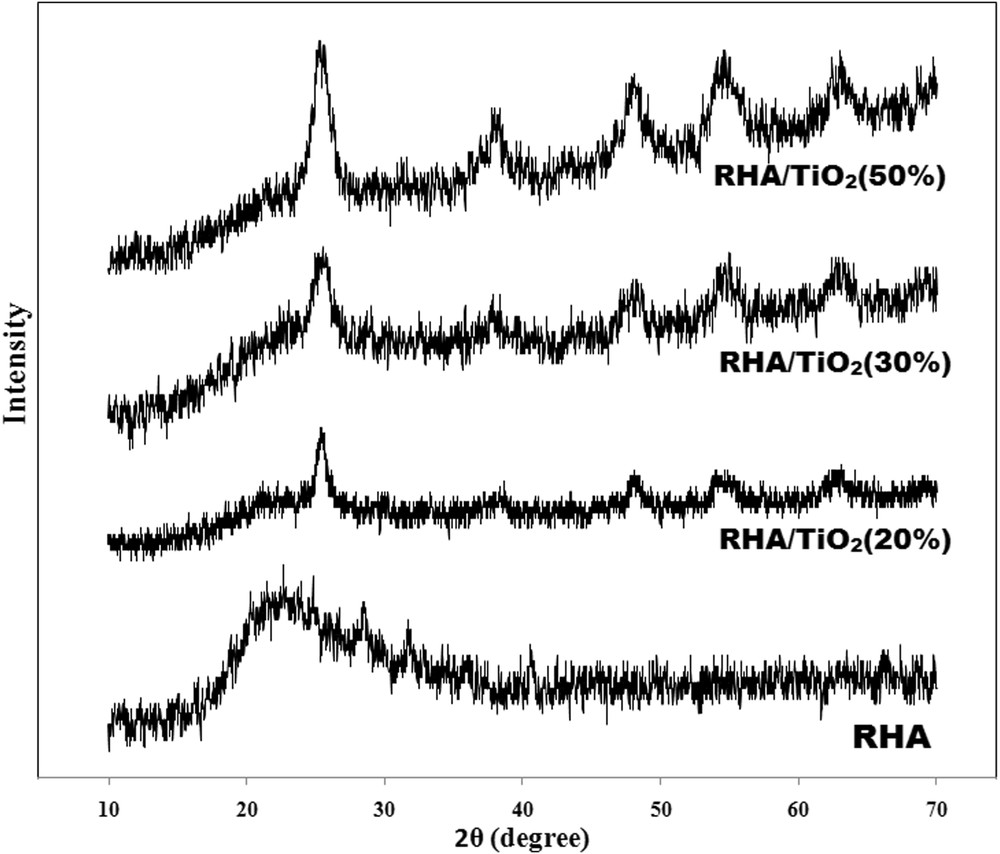
XRD patterns of RHA and various samples of RHA/TiO2 nanocomposites.
The average crystalline sizes of nanocomposites were estimated by XRD using Scherrer’s equation by considering the full width and half maximum (FWHM) value (Table 1). As can be seen, all of the synthesized nanocomposites have almost the same crystalline sizes.
XRD data of RHA/TiO2 nanocomposites.
Entry | Nanocomposite | 2θ | Peak width [FWHM] | Size [nm] |
1 | RHA/TiO2(20%) | 25.35 | 0.40 | 20 |
2 | RHA/TiO2(30%) | 22.38 | 0.38 | 21 |
3 | RHA/TiO2(50%) | 25.36 | 0.36 | 22 |
2.5.3 SEM and TEM analysis
Scanning electron microscopy (SEM) and transmission electron microscopy (TEM) were performed for the determination of the size distribution, particle shape and surface morphology, as shown in Fig. 3 and Fig. 4. It should be noted that these analysis were carried out on RHA/TiO2(30%) (the best ratio of RHA/TiO2 with the highest catalytic activity was determined in the acetylation of 4-chlorobenzyl alcohol). The SEM pictures show that RHA has a porous and irregular shape [25], and the porosity was substantially maintained over dispersion of TiO2 in RHA/TiO2(30%). It is clear from the TEM images that TiO2 nanoparticles, which can be seen as dark particles, are spherical with average size less than 10 nm in diameter, which have been dispersed in the matrix of RHA, as shown in Fig. 4.
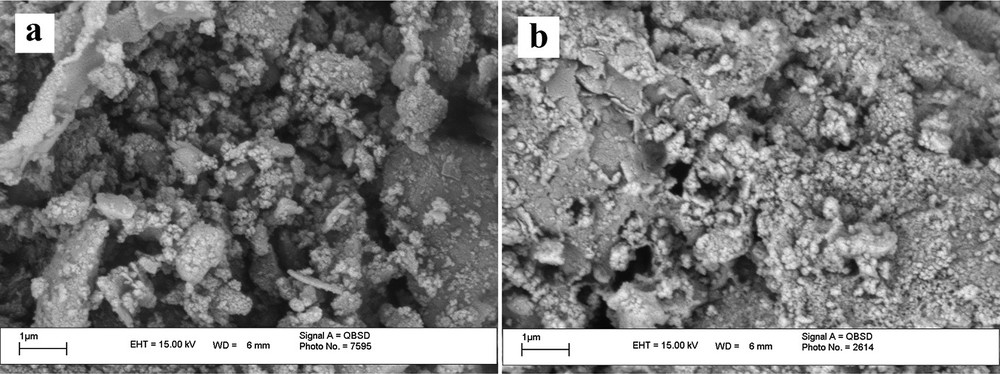
SEM images of RHA (a) and RHA/TiO2(30%) (b).
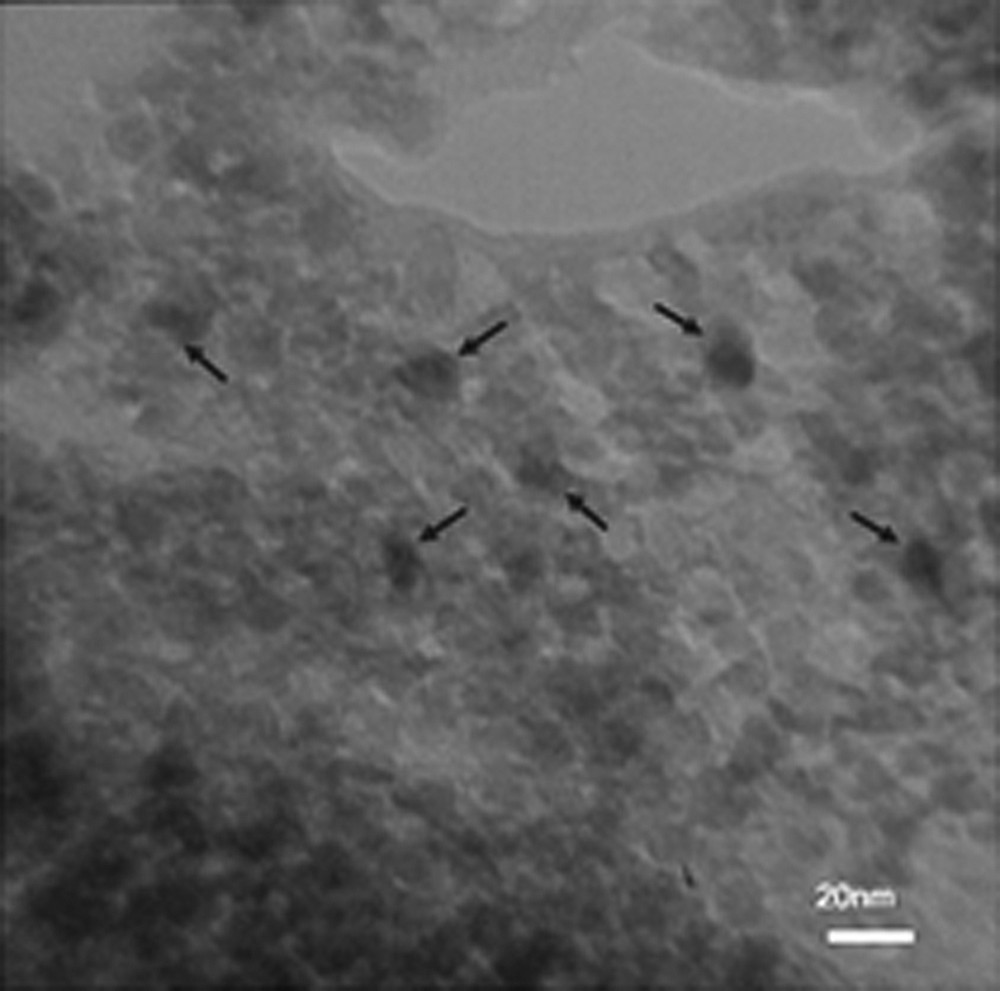
TEM images of RHA/TiO2 (30%).
2.5.4 N2 adsorption–desorption measurements
N2 adsorption–desorption measurements based on BET and BJH methods, which have been a powerful tool for nano- or meso-porous material characterization, were performed to obtain more information about the catalyst. The adsorption–desorption isotherm of RHA and RHA/TiO2(30%) nanocomposites is shown in Fig. 5a. Both samples exhibited a type IV isotherm with H3 hysteresis loop categories according to the literature, which indicates that the samples are mesoporous materials with slit-like pores [26]. These results also prove that the textural properties of RHA were substantially maintained over the preparation of the nanocomposite. The specific BET surface areas of RHA and RHA/TiO2(30%) are 57 and 50 m2/g, respectively. Furthermore, the curves of pore size distribution evaluated from desorption data by utilizing the BJH model are also shown in Fig. 5b. These curves show that there are some particles with average size less than 10 nm in diameter in RHA/(30%) which do not exist in RHA. These particles can be attributed to the TiO2 nanoparticles.
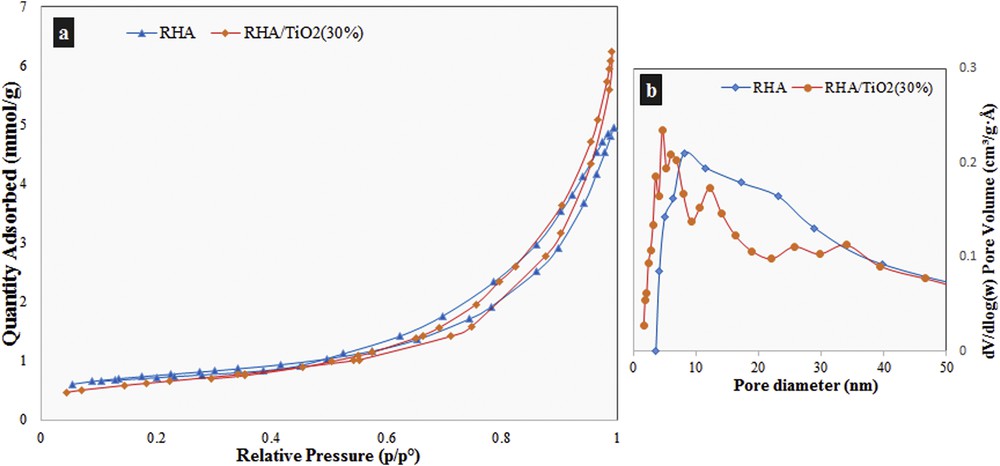
The N2 adsorption–desorption isotherm (a), and pore size distribution (b) of RHA and RHA/TiO2(30%).
3 Results and discussion
On the basis of the information obtained from the studies on RHA/TiO2 nanocomposites, it is expected that this reagent can be used as a catalyst for the promotion of the organic reactions. So this reagent was used in the promotion of the conversion of alcohols, phenols and amines to their corresponding acetates and/or amides with acetic anhydride (Scheme 1).
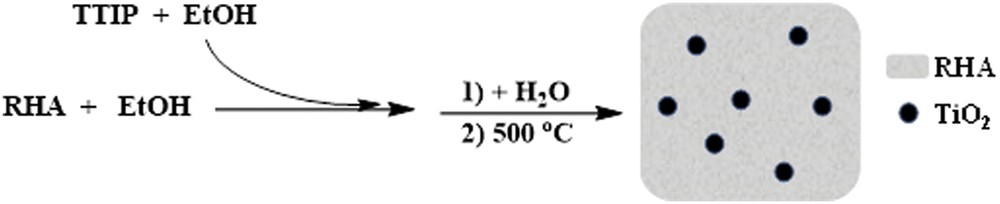
Preparation of RHA/TiO2 nanocomposites.
In the first step we focused our attention toward the optimization of the ratio of RHA to TiO2 for obtaining the highest catalytic activity. For this purpose, acetylation of 4-chlorobenzyl alcohol was studied and the best results were obtained using RHA/TiO2(30%) (Table 2). Further increase in this ratio did not improve the activity of the prepared nanocomposite. Then for obtaining the optimum reaction conditions, we studied the influence of the following factors on the acetylation of 4-chlorobenzyl alcohol with acetic anhydride: i) the amounts of the catalyst; ii) solvent or solvent-less media and iii) temperature. The obtained results clarified that the best conditions are the ones which are shown in Scheme 2.
Optimization of the best ratio of RHA/TiO2 nanocomposites in the acetylation of 4-chlorobenzyl alcohol.
Entry | Catalyst | Time (min) | Conversion (%) |
1 | RHA/TiO2(20%) | 15 | 85 |
2 | RHA/TiO2(30%) | 8 | 100 |
3 | RHA/TiO2(50%) | 8 | 100 |
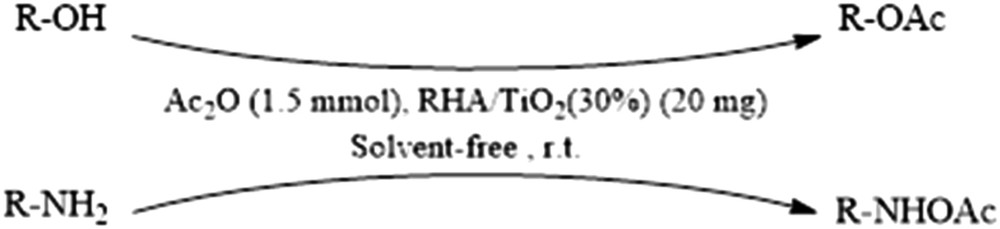
Acetylation of alcohols, phenols and amines catalyzed by RHA/TiO2(30%).
After optimization of the reaction conditions, different types of alcohols were subjected to the acetylation using this method (Table 3). Acetylation of various benzylic alcohols containing electron-withdrawing and electron-donating substituents proceeded efficiently with high isolated yields (Table 3, Entries 1–7). Primary, secondary and tertiary aliphatic alcohols were also efficiently converted to their corresponding acetates in almost quantitative yields at room temperature (Table 3, Entries 8–12). No elimination and rearrangement by-products were observed at all. Phenol and its derivatives also undergo acetylation easily using this method and their corresponding acetates can be isolated in excellent yields (Table 3, Entries 13–16). In the case of pyrogallol, higher amounts of the catalyst are needed due to high steric hindrance (Table 3, Entry 16). This method is also very useful for the acetylation of amines with acetic anhydride. All reactions are performed under mild reaction conditions in very short reaction times with high yields (Table 3, Entries 17–20).
Acetylation of alcohols, phenols and amines in the presence of RHA/TiO2(30%) under solvent-free conditions.
Entry | Aldehyde | Product | Time (min) | Yield (%)a |
1 | 7 | 96 | ||
2 | 8 (8,9,10,10)b | 97 (95,96,97,94) | ||
3 | 10 | 91 | ||
4 | 10 | 93 | ||
5 | 10 | 94 | ||
6 | 20 | 94 | ||
7 | 10 | 95 | ||
8 | 15 | 90 | ||
9 | 12 | 88 | ||
10 | 14 | 93 | ||
11 | 0.5 | 96 | ||
12 | 0.5 | 96 | ||
13 | 12 | 94 | ||
14 | 15 | 90 | ||
15 | 20 | 91 | ||
16c | 25 | 90 | ||
17 | 7 | 93 | ||
18 | 7 | 93 | ||
19 | 10 | 92 | ||
20 | 7 | 94 |
a Isolated yields.
b Results obtained using the recovered catalyst.
c 40 mg of the catalyst was used.
To check the reusability of the catalyst, the reaction of 4-chlorobenzyl alcohol and acetic anhydride under the optimized reaction conditions was studied. When the reaction was completed, dichloromethane was added and the catalyst was separated by filtration. The recovered catalyst was washed with dichloromethane, dried and reused for the same reaction. This process was carried out over five runs and all reactions led to the desired products with high efficiency (Table 3, Entry 2).
The possible mechanism for the acetylation of alcohols, phenols and amines in the presence of RHA/TiO2(30%) as a promoter is shown in Scheme 3. On the basis of this mechanism, RHA/TiO2(30%) catalyzes the reaction by the electrophilic activation of Ac2O to form a zwitterionic species, making the carbonyl group susceptible to nucleophilic attack by the substrate. Successive elimination of CH3CO2H results in the formation of the requested product and regenerates RHA-SO3H in the reaction mixture.

Proposed mechanism of the reaction.
To illustrate the efficiency of the proposed method, Table 4 compares our results in the acetylation of 4-chlorobenzyl alcohol with those reported by using the relevant reagents in the literature. It is clear that the present method is superior in terms of the reaction time and catalyst amount, especially compared with rice husk (RiH), rice husk ash (RHA) and TiO2 (Table 4, Entries 5–7).
Comparison of the results of the acetylation of benzyl alcohol catalyzed by RHA/TiO2(30%) with those obtained by using some of the reported catalysts.
Entry | Catalyst (loading) | Reaction conditions | Time (min) | Yield (%) | [Ref.] |
1 | Zeolite HSZ-360 (20 mg) | 60 °C/Neat | 60 | 84 | [1] |
2 | Cu(OTf)2 (2.5 mol%) | r.t./CH2Cl2 | 30 | 97 | [2] |
3 | RuCl3 (5 mol%) | r.t./CH3CN | 10 | 98 | [3] |
4 | Saccharinsulfonic acid (5 mol%) | Reflux/CH2Cl2 | 120 | 85 | [4] |
5 | RiH (300 mg) | 80 °C/Neat | 60 | 94 | [5] |
6 | RHA (50 mg) | 80 °C/Neat | 15 | 93 | [6] |
7 | TiO2 (30 mg) | 100 °C/Neat | 15 | 95 | This work |
8 | RHA/TiO2(30%) (20 mg) | r.t./Neat | 0.5 | 95 | This work |
4 Conclusions
In conclusion, rice husk ash was used as a silica support for the synthesis of anatase-phase titania nanoparticles and a RHA/TiO2 nanocomposite was obtained. Then, a simple and efficient protocol for the acetylation of alcohols, phenols and amines was developed using this nanocomposite. The methodology has several advantages such as: (i) high reaction rates and excellent yields, (ii) no side reactions, (iii) ease of preparation and handling of the catalyst, (iv) cost efficiency and effective reusability of the catalyst, (v) use of an inexpensive catalyst with lower loading and (vi) a simple experimental procedure and solvent free conditions. Further work to explore this catalyst in other organic transformations is in progress.
Acknowledgments
We are thankful to the University of Guilan Research Council for partial support of this work.