1 Introduction
Global production from capture fisheries and aquaculture supplied about 130 million tonnes of fish per year [1]. Seventy six percent (100 million tonnes) of this catch is intended for human consumption. However, depending on fish species and processing (canning, freezing, and so forth), only 50–70% of this catch is really used in human diet. The remainder, such as fishery by-products (e.g., heads, frames, skin, and viscera), are especially converted into animal feed and oil. However, this underutilized material has a nutritional value almost as good as whole fish [2]. Although there are many approaches to further utilize these resources, interest has been expressed in isolating or processing value-added components [3].
Cephalopods are an important economic resource for global fisheries. The cuttlefish (Sepia officinalis) is among the most exploited marine species in the Mediterranean and Atlantic waters. In the Mediterranean, the main resource of cuttlefish is located in the Gulf of Gabes (south-east of Tunisia), and the landings occur essentially in the fishing port of Sfax. The most important fisheries of cuttlefish in the northeast Atlantic waters are found in the “La Manche” channel, where S. officinalis comprised 66% of the total cephalopods landed in Basse-Normandie, which is the foremost producing region in France. During transformation steps in seafood plants, large quantities of waste, including viscera, are generated and discarded. It is estimated that 40% of the total body weight ends up as a processing by-product that is not utilized, causing a serious disposal problem. Traditionally, marine viscera have been considered as waste and have been utilized only to a minor extent [4]. Nowadays, marine by-product hydrolysates are widely investigated for their biological activities, and different purification ways are used for that purpose [5,6].
In the recent years, cephalopoda have been studied only to a minor extent in terms of enzymatic hydrolysis. The cuttlefish S. officinalis viscera have been studied only in terms of autolysis [7]. However, fish enzymes have been widely studied in terms of structural modification, functional properties, biological activities, and lipid and phospholipid recoveries [8–12].
In recent years, a large number of biologically active peptides have been generated from fishery waste and by-products (e.g., heads, frames, and viscera). Consequently, interesting and very promising new applications for the fish and shrimp by-product hydrolysates have emerged. For example, active factors such as peptides inhibiting the angiotensin I-converting enzyme (thus exhibiting an antihypertensive effect), gastrointestinal peptides such as gastrin and cholecystokinins 9–10, cellular growth factors11, factors such as calcitonin and calcitonin gene-related peptide 12 were detected in hydrolysate fractions. The presence of antioxidant compounds in marine hydrolysates has also been reported [13].
Production of fish protein hydrolysate (FPH) by proteinase treatment is a mean to transform by-products into products with improved functional and biological properties. Indeed, appropriate hydrolysis parameters can produce hydrolysates with different biological activities (e.g., antihypertensive, immunostimulatory, antioxidant, and so forth).
Enzymatic protein hydrolysis is a promising process for underutilized marine products. FPHs from various sources have been studied extensively and described by several researchers [14]. Enzymatic hydrolysis allows the production of small peptides and even free amino acids [15].
The molecular weight (MW) of the hydrolyzed proteins is one of the most important factors in producing protein hydrolysates with bioactive peptides. Accordingly, to obtain peptide fractions with desired molecular size, we have used ultrafiltration (UF) and nanofiltration (NF) systems. These processes used porous membranes characterized by the molecular weight cutoff (MWCO), which indicates the smallest MW component retained at 90% [16]. UF and NF aim at concentrating and/or fractionating one or several components of a solution to permit selective passage of one or several of these components through the membrane.
In the present study, the potential of various membranes to fractionate an FPH using UF and NF membranes is described in a first step. In a second step, a process integrating appropriate membranes was applied to fractionate and concentrate.
2 Materials and methods
2.1 Materials
The spray-dried cuttlefish Protein hydrolysates (CPH) (0.1 < MW < 10 kDa) were obtained by Protamex, Alcalase, and Flavourzyme bioreactor hydrolysis at the laboratory.
2.1.1 Biological material
Cuttlefish (S. officinalis) were provided by the seafood processing company “Calembo” (Sfax, Tunisia). The cephalopods were then immediately stored in ice and transported to the laboratory where they were eviscerated. The collected viscera were homogenized for 1 min and then frozen at −80 °C until used. Endogenous enzymes were not inactivated. The cuttlefish viscera fraction included all of the organs usually found in the abdomen of mature specimens, that is, the digestive gland, esophagi, stomach, digestive ducts, pyloric caeca, pancreatic diverticula, gonads, and accessory nidamental glands; only the ink gland was removed.
2.1.2 Enzymes
Enzymes used for the hydrolysis of cuttlefish viscera were provided by Novozymes A/S (Bagsvaerd, Denmark). Protamex is a Bacillus protease complex. Alcalase 2.4 L is a bacterial serine endopeptidase prepared from a strain of Bacillus lichenformis. Flavourzyme 500 MG is a fungal protease/peptidase complex produced by submerged fermentation of a selected strain of Aspergillus oryzae. It contains both endoprotease and exoprotease activities.
2.2 Experimental setup
2.2.1 Bioreactor hydrolysis process
Fig. 1 outlines the overall process used in the production of cuttlefish viscera hydrolysates. The frozen and minced viscera were kept overnight at 4 °C for thawing and homogenized with MilliQ-purified water (ratio 1/1, w/v). For each hydrolysis, 100 g sample viscera were used and the mixture was directly poured into a 300 mL closed glass vessel with a double jacket to enable thermic exchanges (heating or cooling). All hydrolysis reactions were performed at pH 8 and 50 °C for optimal enzyme activity (Alcalase, Protamex, and Flavourzyme). Before starting of the hydrolysis reaction, an initial 15 min mixing was done for the pH adjustment (through the addition of 1 M NaOH) and the obtention of the desired temperature (using a water bath). The hydrolysis reaction started by adding 0.1 and 1.5% w/w for Alcalase and Protamex, respectively. The whole system was continuously stirred at 300 rpm for 24 h. Temperature and pH were controlled by a pH-stat method (TIM 854, Radiometer analytical, SAS, France) and by the addition of 1 M NaOH. Such pH-stat allowed the estimation of the degree of hydrolysis (DH) based on the consumption of alkali to maintain pH at the desired value [17].
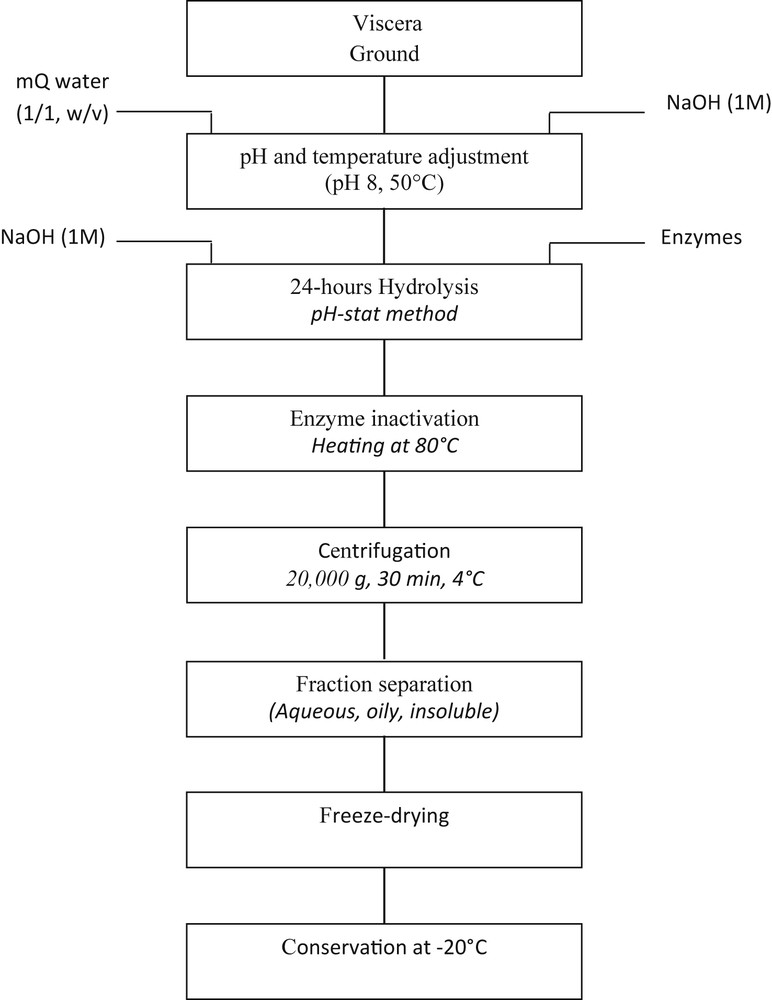
Flow scheme of the hydrolysis process.
The hydrolysis proceeded for 24 h and was stopped by heating at 80 °C for 15 min. After cooling to room temperature, hydrolysates were centrifuged at 20,000g for 30 min, and the aqueous (soluble) phase was then freeze-dried and stored at −20 °C.
2.2.2 Membrane fractionation
A UF/NF Microlab 40 pilot plant (VMA Industry) with a maximum capacity of 5 L was used (Fig. 2). Two organic UF membrane PCI with a tubular configuration were selected for treatment of CPH in total recycling mode (i.e., permeate + retentate): MT 44-ES 404 [MWCO 1–4 kDa, polyethersulfone (PES)] and XP 117 (MWCO 5 kDa, PES). The material used was specially manufactured for a feeble protein affinity [18], with a high selectivity and chemical resistance and also high fluxes and recovery rates. Operating conditions were tangential velocity 2.5 m s−1, temperature 25 °C, and pressure ranging from 8 to 25 bar. Although operating conditions were not optimized, they can be considered as standard conditions for the membranes, thus allowing a comparison of membrane performances, which were described by the evaluation of retention rate (RR).
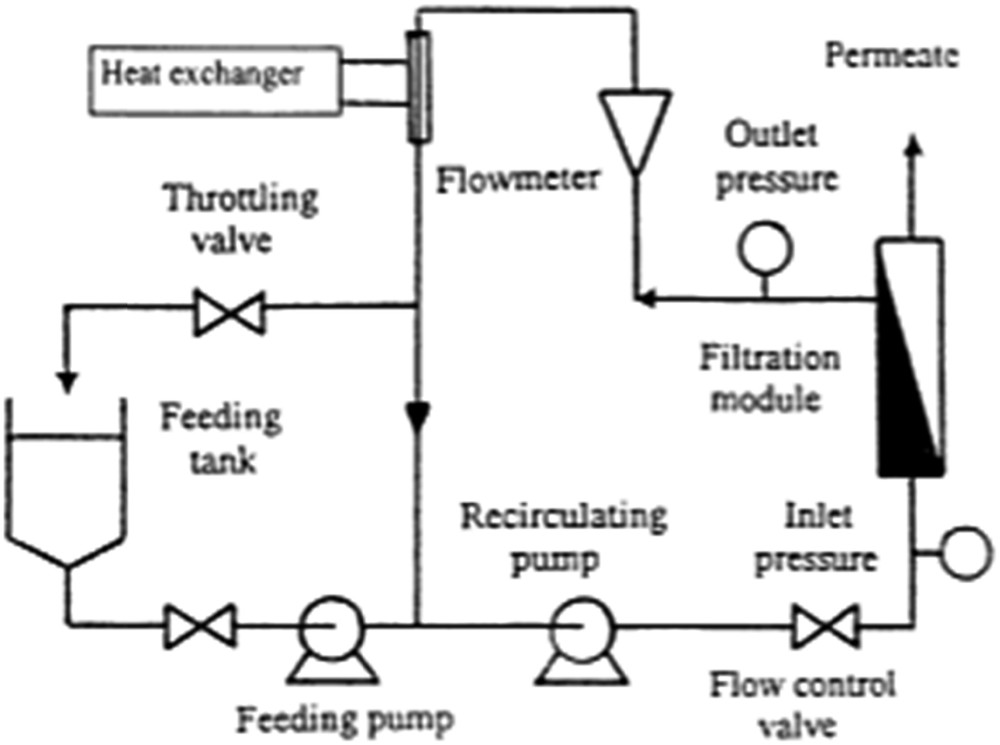
Schematic representation of the pilot plant.
The feeding tank of the Microlab 40 pilot plant was filled with 4 L of the hydrolysate solution obtained with Protamex, Alcalase, and Flavourzyme. Permeation fluxes were measured until a volume reduction factor of 4 was obtained.
2.3 Methods
All of the chemical analyses were performed in triplicate.
2.3.1 Dry matter and ash
The percentage of dry matter was estimated gravimetrically. Ash content was calculated by weighing the samples after an overnight heating of the dried samples at 600 °C.
2.3.2 Lipid extraction
Lipids were extracted according to the Folch procedure [19]. The total lipid content was determined gravimetrically after solvent extraction and evaporation.
2.3.2.1 Protein content
The total protein (N × 6.25) content of samples was determined using the Kjeldahl method [20].
2.3.3 Process performances
2.3.3.1 Degree of hydrolysis
The process performances are principally described by the degree of hydrolysis (number of peptide bonds broken/total peptide bonds) for the enzymatic hydrolysis process.
The values of the DH could be obtained according to the following expression [17]:
- where DH is a percent ratio between the number of peptide bonds cleaved and the total number of peptide bonds in the substrate; V is the volume (mL) of sodium hydroxide consumed during hydrolysis; M is the molarity of sodium hydroxide; α is the dissociation factor for α-NH2 groups = 0.88 at 50 °C; mp is the mass (g) of protein in the substrate introduced in the reaction (protein content determined as N × 6.25); and htot is the total number of peptide bonds in the protein = 8.6 equiv g kg−1 protein in fish samples.
2.3.3.2 Membrane fractionation
2.3.3.2.1 Water permeability
To understand the behavior of the membranes used for water filtration and to evaluate cleaning procedure efficiency, membrane permeability was determined (eq. 1). The first membrane permeability is the permeability determined before the first hydrolysate filtration. This first permeability (FP) is used as a reference value to determine the regeneration rate of the membrane, that is, the cleaning procedure efficiency to recover the initial state of the membrane (eq. 2).
(1) |
(2) |
After each use, the membrane was cleaned up according to the procedures recommended by the manufacturer. Water flux is measured either after a complete cleaning cycle (Jn), or after every acid and alkaline cleaning. The cleanability means the ability of the membrane to be regenerated after the hydrolysate filtration. It is expressed as the percentage of recovered flux after membrane cleaning (eq. 3):
(3) |
For membrane fractionation, the permeation flux and the selectivity of the membrane were followed. The permeation flux is defined by the volume of solution crossing the membrane per unit of surface and of time (L/h m−2). The selectivity is defined as the proportion of peptides retained by the membrane and can be expressed using MW distribution profile, or as follows, by the RR (eq. 4):
(4) |
2.3.4 MW distribution profile
MW distribution profiles of permeates and retentates were analyzed by size exclusion chromatography using a Tricorn Superdex Peptide 10/300GL (Amersham Pharmacia Biotech) in Fast Protein Liquid Chromatography (FPLC) mode (fractionation range of the column was 100–7000 Da) [13]. The maximal pressure admitted is 300 psi. The mobile phase (isocratic elution) consisted of water with TFA 0.1% and acetonitrile (70:30). The flow rate was 0.5 mL/min. The total column volume was 24 mL. The samples were centrifuged at 10,000 rpm and the volume deposited was 200 μL. The calibration standards injected were leucine–tyrosine (294 Da), vasopressine (1084 Da), insuline chaîne β (3496 Da), and ribonucléase A (13,700 Da).
The liquid chromatographic system consisted of a BioCAD SPRINT (PerSeptive Biosystems). The optic density of the compounds was read at 214 nm. This wavelength corresponds to the absorbance of peptidic bonds. Diamir (JMBS software) was used to collect, plot, and process the chromatographic data.
3 Results and discussion
3.1 Chemical composition of the raw material
As indicated in Table 1, the proximal compositions of the raw materials were 75% of moisture content, 2% of minerals, and about 18% of proteins.
Global chemical composition of the raw material (nonhydrolyzed viscera).
Substrate | Moisture | Ash | Total lipids | Proteins |
Raw cuttlefish viscera | 74.99 ± 0.1% | 1.95 ± 0.0% | 4.78 ± 0.7% | 17.45 ± 0.25% |
3.2 Degree of hydrolysis
After 24 h of hydrolysis, DH values for cuttlefish viscera were 3.2, 6.8, and 7%, respectively, for Protamex, Flavourzyme, and Alcalase. Hence, it appears that Alcalase performed the best in terms of DH for both substrates. This means that the peptides generated have the lowest MW. Therefore, the freeze-dried Alcalase hydrolysates were chosen for the fractionation process.
3.3 Nitrogen recovery
Protein recoveries into the soluble fraction after hydrolysis are given in Table 2. Protamex only led to 57.2% of protein recovery, whereas with Alcalase up to 64.3% of proteins have been recovered. As expected, these results are in accordance with the DH measured during hydrolysis.
Protein recoveries in the aqueous phase of cuttlefish hydrolysates.
Protein recovery (%) | Protamex | Alcalase | Flavourzyme |
Cuttlefish hydrolysates | 57.2 | 64.3 | 60.3 |
3.4 Measurement of hydrolysate/membrane material affinity
Static adsorption tests were carried out to evaluate the intensity of hydrolysate/membrane interactions. The adsorption extent was quantified in terms of flux reduction and pore diameter reduction. These parameters were mainly influenced by the membrane MWCO. They decreased as the MWCO increased, which means that peptide adsorption at the pore surface happened as a layer of a quasi-constant thickness. The chemical cleaning of fouled membranes with the NaOH–SDS solutions allowed more than 95% of the initial water flux to be recovered. Then, despite the high reduction in flux observed, especially for the 300 Da membrane, all these membranes should be correctly regenerated after treatment of the cuttlefish hydrolysate.
3.5 Potentiality of membranes to fractionate and concentrate CPH
The objective of this experimental work was to study the potentialities of membrane processes for concentrating and fractionating cuttlefish hydrolysates with a wide range of MW distribution.
According to the RR, UF membranes can be used to
- - separate peptides from enzyme or nonhydrolyzed proteins if the membrane has a low RR (in this case, peptides can pass freely through the membrane but proteins are retained);
- - concentrate peptide solutions if the membrane has a maximum RR (about 100%);
- - purify solutions (e.g., to eliminate salts or decrease undesirable odor) if the membrane has a high rejection rate; and
- - fractionate peptides with respect to their MW distribution if the membrane has an intermediate RR.
3.6 Follow-up of membrane permeation fluxes and regeneration
Water permeation fluxes obtained after the acid–alkaline initial cleaning procedure and before and after hydrolysate UF with a cleaning operation are presented in Table 3.
Permeation fluxes before, during, and after ultrafiltration of cuttlefish hydrolysate at 15 bar and 5 and 15 bar and 25 °C.
Membrane | XP 117 | MT44-ES 404 |
Ji (L/h m2) | 612.41 | 216.97 |
J (L/h m2) | 10.41 | 7.84 |
J0 (L/h m2) | 80.32 | 47.74 |
Jf (L/h m2) | 458.9 | 156.17 |
Regeneration rate (%) | 75% | 72% |
Fig. 3 outlines the evolution of water permeation fluxes estimated after acid and alkaline conditioning, hydrolysate UF, and again an acid–alkaline cleaning process after filtration essays on the XP 117 and MT44-ES 404 membranes. At first sight, it appears that water permeation fluxes are higher with the XP 117 membrane, regardless of the filtration cycle phase. In fact, the Ji values of XP 117 are three times higher than that of the ES 404. This factor 3 is kept for all of the different phases, that is, permeate fluxes directly measured after hydrolysate filtration (J0) and also water fluxes estimated after the cleaning process (Jf). This result is owing to different pore densities.

Evolution of water permeation fluxes after each step of the filtration cycle with the XP 117 and ES 404 membranes. TMP = 15 bar, θ = 25 °C.
After cuttlefish hydrolysate filtration, the permeate flux significantly decreases from 621.5 L/h m2 (after acid and alkaline cleaning) to 80.32 L/h m2 for XP 117 and from 219.22 to 47.74 L/h m2 for ES 404, with decrease rates of 87 and 78%, respectively. After the cleaning procedure prescribed by the manufacturer, water fluxes rise up to 458 and 156.17 L/h m2, with regeneration rates of 75 and 72%, respectively.
In Table 3, the regeneration rates are those calculated at the end of a filtration cycle. However, in this study, the regeneration rates were also determined after each cleaning procedure (acid or alkaline flush) with an estimation of permeation fluxes with distilled water (Fig. 4).
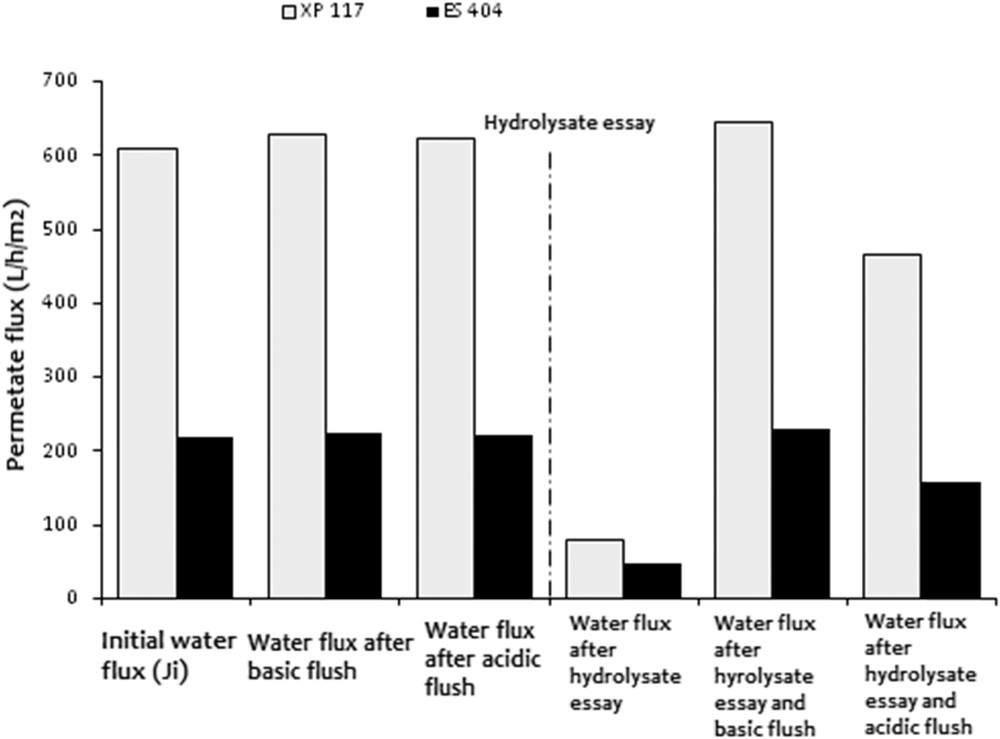
Water permeation fluxes obtained after filtration of hydrolysate samples (TMP = 15 bar, θ = 25 °C) for the XP 117 and ES 404 membranes.
From Fig. 4, we can notice that after hydrolysate filtration followed by Ultrasil alkaline cleaning, the water permeation fluxes are totally recovered and sometimes even higher than those initially calculated before the filtration of the hydrolysate (XP 117). The apparent constriction of the membrane pores is because of the effect of the acidic treatment.
However, the classical leaning procedure recommended by the manufacturer involves a cleaning process with the nitric acid to neutralize the separation interface and to avoid the chemical changing in the hydrolysate sample to filter. In this study, after the acidic cleaning procedure, the regeneration rates decrease for both of the membranes (Fig. 4). Thus, the classical cleaning procedure does not seem to be efficient for the recovery of the initial characteristics of the membranes because of the fouling. According to Dumay et al. [10], the use of more drastic cleaning conditions (reagent concentration, contact time, and temperature) would help to increase the regeneration rates only up to 5% and as a consequence does not justify such energy and time consumption.
3.7 Analysis of MWs of the peptidic populations
The impact of fractionation on the CPH peptidic population is shown. Peptidic populations are described in terms of chromatographic profiles (SEC-FPLC). The elution profiles of the permeates and the retentates resulting from the fractionation with the ES 404 and XP 117 membranes were compared to the peptidic profile of the raw hydrolysate. These are reported in Fig. 5.
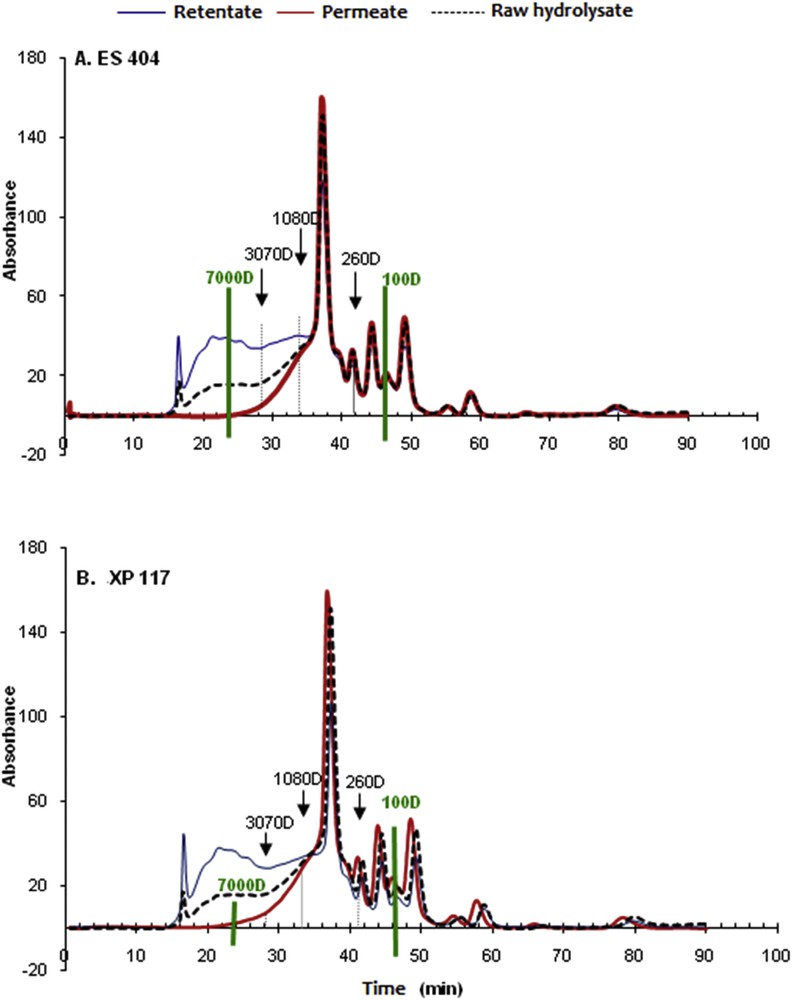
Chromatographic profiles of the retentates and the permeates obtained after hydrolysate ultrafiltration with the ES 404 (A) and XP 117 (B) membranes. TMP = 5 bar, θ = 25 °C, v = 2.3 m s−1, and volume reduction factor = 4.
A quick comparison between the chromatograms shows that the profiles are similar. A clear discrimination appears between the peptidic profile of the permeate and one of the retentates concerning the peptides with an MW > 3000 Da. The elution profiles for the two membranes show an important peak in the permeate concerning molecules with an MW < 1000 Da. Concerning the retentate, the peak is near 13,000 Da.
Under an MW of 3000 Da, the superposition of the three chromatograms, especially for the ES 404, shows the good selectivity of the membranes depending on the MWCO. Moreover, we can notice the existence of “pivot point” where the peptidic composition of the resulting filtration fraction will vary. Two pivot points are highlighted here as follows:
- - One of the ES 404 membranes (Fig. 5A), where the permeate and the retentate are enriched with peptides, respectively, > 0.7 and < 0.7 kDa.
- - One of the XP 117 membranes (Fig. 5B), where the permeate and the retentate are enriched with peptides, respectively, > 1.08 and < 1.08 kDa.
The RRs of the tested membranes are shown in Fig. 6. More than 90% of the peptides superior to 3 kDa are retained by both of the membranes. The RRs for the peptidic population classes > 2.1 kDa are always inferior for the UF XP 117. The UF ES 404 is therefore more efficient and selective. For example, concerning an MW > 7 kDa, the RR is 100% for ES 404 and only 93.47% for XP 117. The same thing is noticed for peptidic populations comprised between 5.4 and 7.1 kDa, the RR is 99% for ES 404 and only 91.52% for XP 117. Under an MW of 3 kDa, the RRs show a decrease but remain higher for ES 404. Concerning the interval 2.1–3 kDa, the RRs are 75.14 and 63.07%, respectively, for ES 404 and XP 117 membranes.
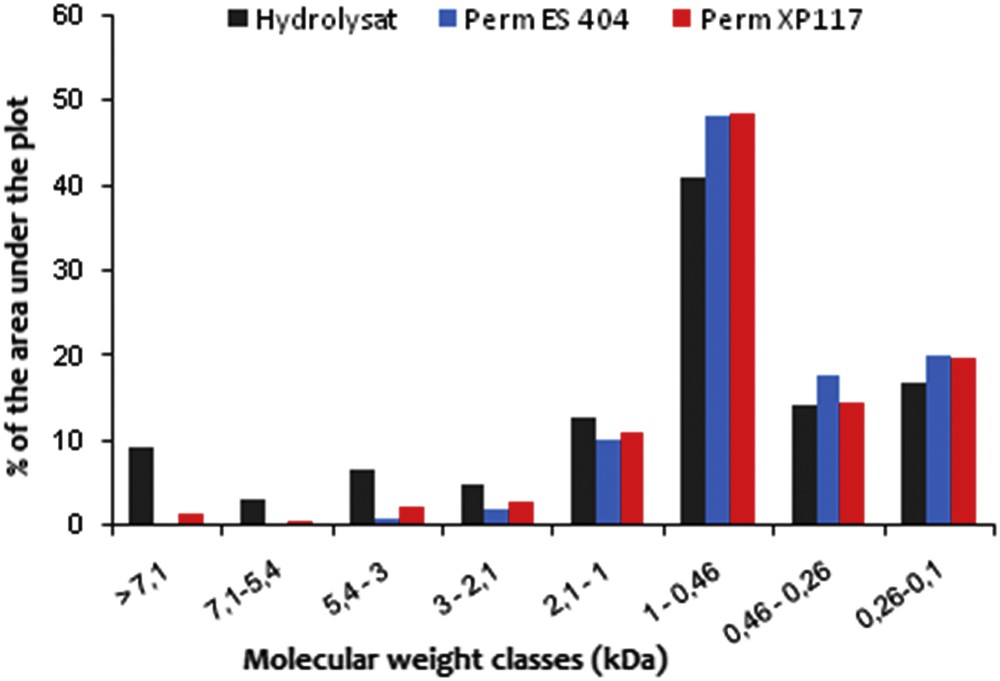
Retention rates of the peptides (%) according to the molecular weight classes calculated after filtration of the cuttlefish viscera hydrolysate with the ES 404 and XP 117 membranes. Transmembrane Pressure (TMP) = 5 bar, volume reduction factor = 4, θ = 25 °C, and v = 2.3 m s−1.
Anyway, the combination of such operations allows to make the difference between the fractionation performances of the two membrane performances and consequently to distinguish between the materials used. The ES 404 membrane composed of PES with an MWCO of 4 kDa is the most interesting to be used in an industrial scale. Thus, this membrane may be used for the concentration or purification of hydrolysates.
Concerning the result of the selectivity, the examination of the permeate content shows that the most important percentage of the MW peptides is < 1 kDa regardless of the membrane used (Fig. 7). This result is confirmed by MW profiles of the permeate and the retentate (Fig. 8). The elimination of all of the peptides of MW > 4 kDa was observed in the UF permeate. This is explained by a good selectivity of the membrane tested.
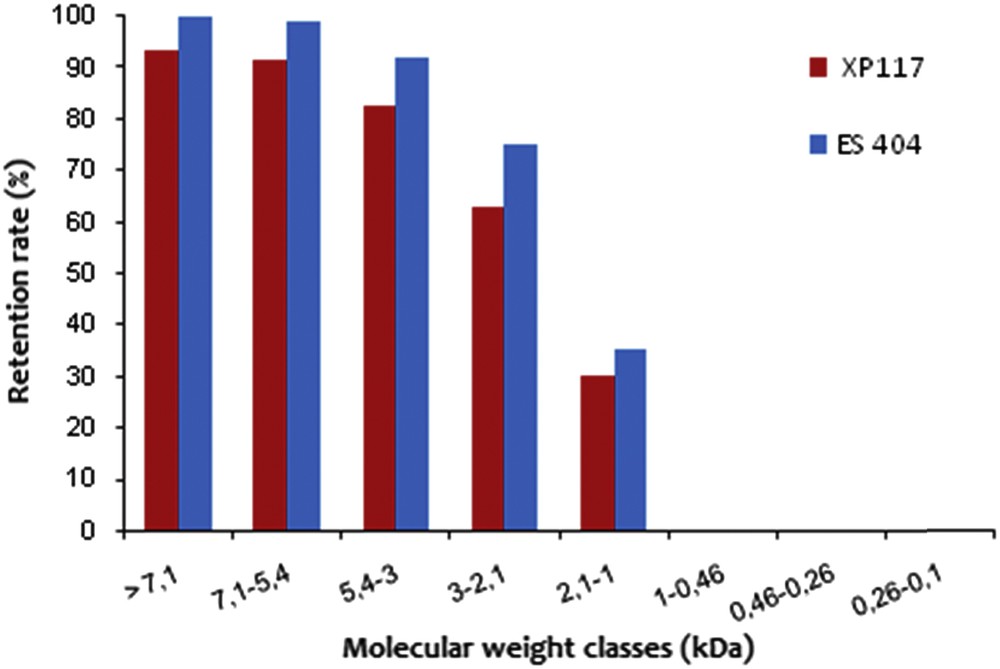
Distribution of the molecular weights of the peptides in the UF permeate obtained by the ES404 and XP 117 membranes. PTM = 5 bar, Volume Reduction Factor (VRF) = 4, θ = 25 °C, v = 2.3 m s−1.

Comparison between the chromatographic profiles of permeates (A) and retentates (B) obtained with the ES 404 and XP 117 membranes. TMP = 5 bar, θ = 25 °C, v = 2.3 m s−1, and volume reduction factor = 4.
Another important peptidic population is represented by oligopeptides with an MW comprised between 260 and 460 Da (17.82% for ES 404 and 14.6% for XP 117). The MW between 100 and 260 Da is about 20% regardless of the membrane used. Thus, after membrane fractionation, the permeate becomes enriched in oligopeptides and amino acids with MW < 260 Da, which fulfills the intended starting objective fixed in this study, which is the obtention of a permeate enriched in amino acids and valuable compounds that may be used for animal nutrition and bioactivities.
To observe the rejection rate of the peptides according to their MWCO, the grading curves of the two UF membranes have been established (Fig. 9). What is noticeable is that beyond an MW of 1500 Da, the curves indicate that the two membranes behave differently. Although both of the membranes retain peptides of high MW, the maximum MWs retained quietly correspond to the nominal MWCO provided by the manufacturers (4000 and 8000 Da). It also appears that the lower MWCO membrane retains better peptides up to 800–1000 Da. This shape is explained by the smaller nominal MWCO of the ES 404 membrane, which will generate higher RR (%) for peptides with MW > 4400 Da. The gap between the two curves is reduced starting from 5000 Da, where the molecules are supposed to be retained by both of the membranes. However, as mentioned previously, the ES 404 shows a better selectivity. The results of the peptidic distributions and the RRs were confirmed by the estimation of the nominal and apparent MWCO. The relatively low peptidic retention of the XP 117 membrane is explained by the apparent MWCO.
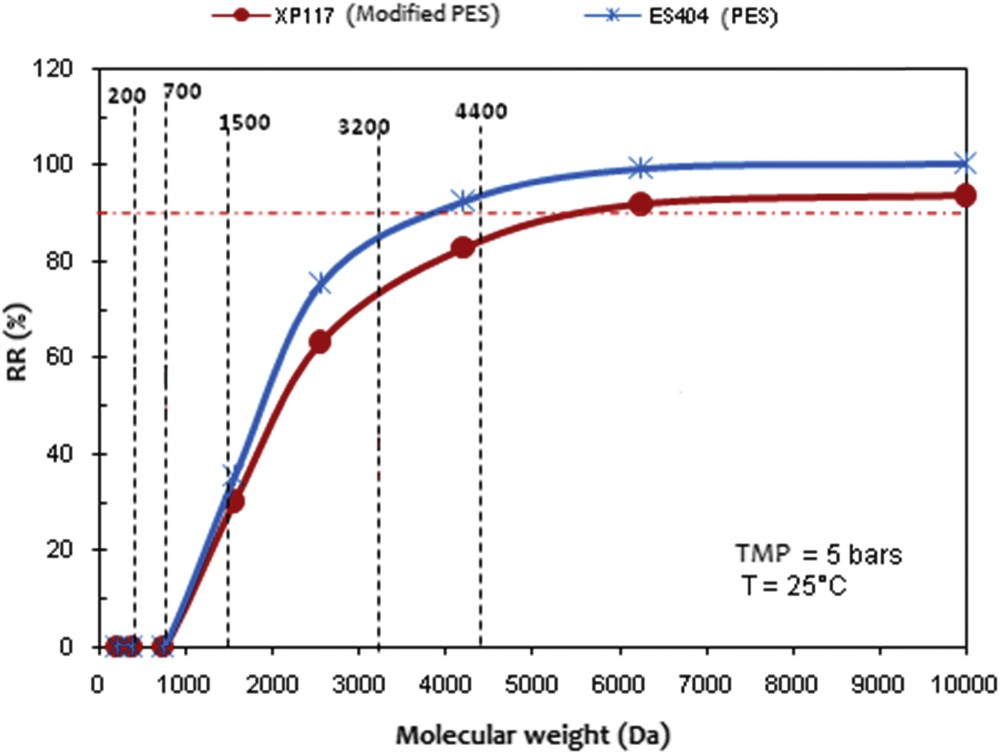
Evolution of the RR (%) according to the molecular weight (Da) after ultrafiltration of the cuttlefish viscera hydrolysate with the ES 404 and XP 117 membranes. TMP = 5 bar, θ = 25 °C, volume reduction factor = 4, and v=2.5 m s−1.
The results of the apparent MWCO are presented in Table 4. These results explain what we have obtained concerning the distribution of peptidic populations and the RR. The apparent MWCO of the XP 117 membrane (experimental MWCO ≤ 4 kDa) is higher than the nominal MWCO. This explains the weak RRs, particularly for peptides with an MW > 5 kDa, which were supposed to be in the concentrate. On the contrary, for the ES 404 membrane, the apparent MWCO is inferior to the nominal MWCO, explaining as a consequence the high RRs obtained for the peptides of an MW between 2.1 and 3 kDa, which is higher than 70%. Thus, the choice of the ES 404 membrane seems to be more suitable for the objectives that we have fixed in this study. In fact, the purposes were to concentrate the biologically active peptides in the retentate and to pass oligopeptides and free amino acids in the permeate for a nutritional purpose.
Nominal and apparent MWCO of the ES 404 and XP 117 membranes obtained after estimation of the RR (%) and drawing of the grading curves.
Membrane characteristics | Nominal MWCO (Da) | Apparent MWCO (Da) |
ES 404 (4 kDa, PES) | 4000 | 3700 |
XP 117 (4 kDa, modified PES) | 4000 | 5500 |
4 Conclusions
Performances of the two UF membranes were tested to determine their potential in a process integrating an enzymatic reactor and membrane filtration steps for the treatment of cuttlefish protein hydrolysates. The aim of this study was also to select a membrane for the fractionation of cuttlefish protein hydrolysate to enrich the permeate in the peptidic fraction < 2 kDa, which are known to have high biological activities. The impact of the membrane MWCO on separation performances (flux, retention capacity, and cleaning effectiveness) was studied.
The study showed the good performances of the organic membranes tested to fractionate cuttlefish protein hydrolysates with biological activity properties. The membranes exhibited interesting selectivity characteristics. The ES 404 4 kDa membrane produced the most enriched permeate in small peptides and was best suited for the objective of this work. According to their performances in terms of peptide retention, these membranes can be used for different purposes. The UF membranes of the intermediate MWCO are potentially well suited for the fractionation of peptides, because their flux and retention are good.
Future works will explore some fractionation criteria other than MW, such as peptide charge and peptide hydrophobicity. A better separation between peptides above and below 2 kDa could also be obtained by varying the pressure, which means that the MWCO of a membrane can be adjusted according to fractionation objective by acting upon the pressure.
Then, the optimized process could be applied to concentration of peptidic fractions with a wide range of biological activities.