1 Introduction
Homogeneous catalysts are frequently used in highly selective organic transformations. Especially in asymmetric synthesis, the contribution of homogeneous catalysts is significant [1] because of a number of important advantages as compared to their heterogeneous counterparts. With the catalyst dissolved and all catalytic sites accessible, homogeneous catalysts are superior in terms of activity and selectivity and possess a high atom efficiency [2,3]. Furthermore, catalytic properties such as chemo-, regio-, and stereoselectivity can be tuned easily because of the well-defined nature of the homogeneous catalysts. Finally due to these beneficial features, homogeneous catalysts can in general be operated under mild reaction conditions.
A major problem in the field of homogeneous catalysis though is separation of the catalyst from the product mixture. The commonly used method, separation by distillation, requires elevated temperatures that are not compatible with most homogeneous catalysts, which in general are thermally sensitive in nature. The homogeneous reactions that have been commercialised either involve volatile substrates and products or do not contain thermally sensitive organic ligands [3]. Both from a commercial and an environmental point of view it would be highly desirable to develop a general method that incorporates the advantages of homogeneous and heterogeneous catalysis into one chemical process. The ultimate goal is to design a catalytic system with a high activity and selectivity, an efficient catalyst recycling and which possesses a high stability. Ultimately, this leads to cleaner, faster and cheaper catalytic processes and eventually will lead to green commercial processes.
2 Homogeneous catalysts on organic supports
An attractive approach to design green commercial processes is the development of recyclable homogeneous catalysts [4]. One way of approaching this challenge is by anchoring the homogeneous catalyst on a large, soluble organic support that can be separated from the product mixture by nano- or ultrafiltration. The advantages of a soluble support are the equal distribution of the active catalytic sites throughout the reaction solution and preservation of the catalytic activity as compared to the corresponding non-supported homogeneous catalyst. Other commonly used approaches for catalyst recycling are the grafting of homogeneous catalysts to solid supports and the use of biphasic systems such as aqueous and fluorous biphasic catalysis, ionic liquids and supercritical fluids [3,5,6].
2.1 Homogeneous catalysts on dendritic supports
Currently, there is considerable attention for the development of multimetallic, macromolecular assemblies for application in homogeneous catalysis under continuously operating conditions in a nanofiltration membrane reactor [2]. These nanosize homogeneous catalysts can be recovered in situ from the product stream by ultra- or nanofiltration techniques and reused again. Ultra- (UF) and nanofiltration (NF) membranes are defined to retain macromolecules with dimensions between 8 and 800 nm and 0.5 and 8 nm, respectively. The recent development of solvent resistant nanofiltration membranes has made the non-destructive, energy efficient separation and concentration of reusable macromolecular catalysts from the product stream by filtration potentially feasible [7]. Already in 1994, the first example of such a macromolecular assembly, a so-called metallodendrimer (i.e. a dodecanickel carbosilane dendrimer), was used as a homogeneous catalyst in the regioselective Kharasch addition of polyhalogenoalkanes to C–C double bonds [8]. This marked the beginning of the development of catalytically active metallodendrimers, a completely new research area in the field of dendrimer chemistry [9].
Recently, the soluble nickelated carbosilane dendrimers 1 and 2 (Fig. 1) were synthesized and their retention behaviour examined in a nanofiltration membrane reactor [10]. A filtration test (with 1) in a NF-membrane reactor (Fig. 2) showed retention of 97.4% of 1 in combination with the MPF-50 membrane. (SelRo-nanofiltration membranes (MPF-60, MWCO = 400 Da; MPF-50, MWCO = 700 Da) were purchased from Koch membrane Systems, Inc., Düsseldorf, Germany; further product information can be found at http://www.kochmembrane.com.) As promising as this seems, it means, however, that in a continuous set-up after 30 exchanged reactor volumes already half the amount of catalytic material has been washed out. For 2, 99.8% retention was found which still is not sufficient for operating under continuous reaction conditions (after 100 exchanged reactor volumes a wash-out of 20% is found). The size and molecular structures of complexes 1 and 2 were determined by molecular modelling studies. (For molecular modelling, the Spartan 5.1.1 [SGI] program was used with MMFF94 as the force field.) Going from 1 to 2 the diameter significantly increases from 2 to 3 nm. Remarkably, this 1 nm increase in radius resulted only in an increase of 2.4% in retention. These dendritic materials possess flexible backbones and are thus able to undergo shape-changes in solution [11]. This behaviour can affect their retention rates by nanofiltration membranes. Even higher-generation dendrimers are still not fully retained by NF-membranes. This behaviour most likely originates from the flexible backbone of these carbosilane dendritic catalysts; going up in generation does not necessarily results in a significant increase in the size of the dendrimers, but rather a back folding of the dendritic branches concomitant with denser molecules is encountered. In addition, the dendrimer size in solution is not well defined and varies upon altering the polarity of the solvent or when pressure is applied [12].

Nickelated carbosilane dendrimers 1 and 2.
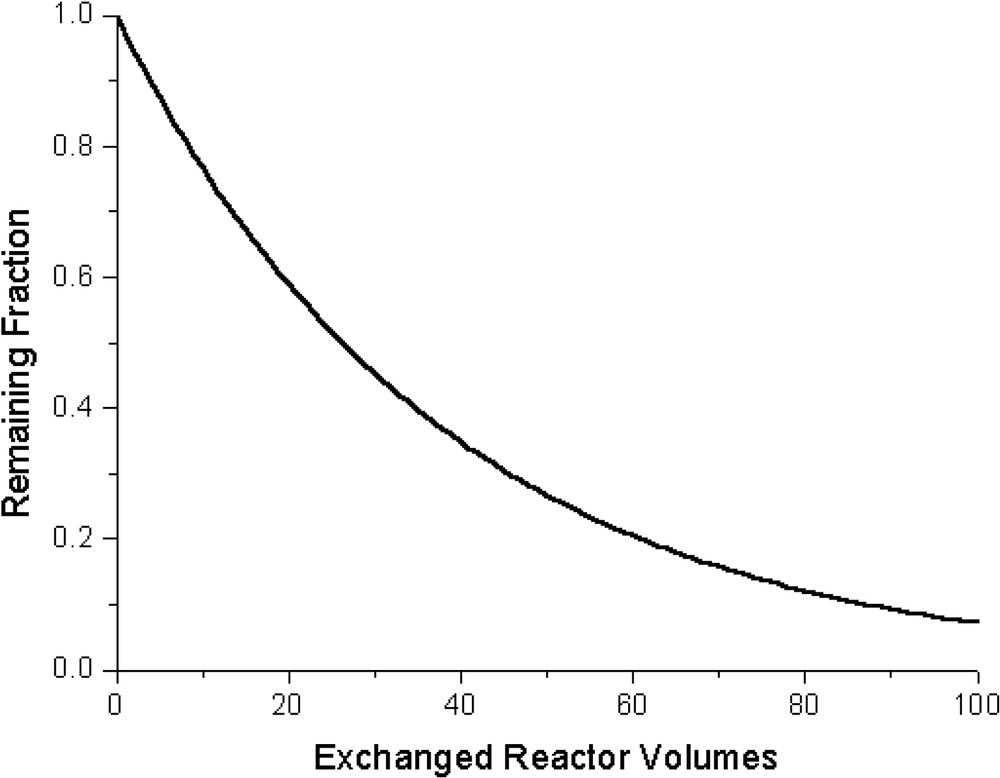
Display of the retained fraction of 1 versus the number of exchanged reactor volumes in a nanofiltration membrane reactor.
2.2 Homogeneous catalysts on shape-persistent supports
To study the role of flexibility of the carbosilane dendrimers on their retention behaviour, we started a detailed investigation on the influences of flexibility (rigidity), size, and geometry of macromolecular catalysts on their retention rate by nanofiltration membranes [13]. Investigating which of these parameters are important for the retention of macromolecules by NF-membranes would enable future predictions of the minimal size and optimal geometry needed for macromolecular catalysts to obtain efficient retention rates. To investigate the importance of rigidity on the retention of macromolecules by NF-membranes shape-persistent catalysts containing rigid aromatic backbones were selected.
In order to prepare recyclable catalysts, the immobilization of so-called pincer catalysts on soluble or solid supports (Fig. 3) was intensively studied in recent years. It was demonstrated that the aryl-C4 position (R′′) is an excellent branching point for anchoring these pincer complexes to support materials such as dendrimers, fullerenes, polymers, solid supports, and peptides [8,10,14]. The coordinating substituents E (mostly NR2, SR or PR2) can also be varied rather easily which has led to the incorporation of various (transition) metals into this monoanionic, terdentate coordinating ECE-ligand. Due to the stabilizing terdentate coordination mode of these metal complexes, they are also rather robust and do not suffer from deactivation or decomposition [15]. As a consequence these organometallic compounds can be used as catalyst in various organic reactions and are, therefore, suited for attachment to soluble organic macromolecular supports in order to create recyclable homogeneous catalysts.

Metalated pincer complexes (for attachment) on supporting materials.
For our studies to investigate the influences of flexibility (rigidity), size, and geometry of macromolecular complexes on their retention rate by nanofiltration membranes, the [PdIIX(NCN)] moiety was used as the catalytically active site. This moiety was chosen because of its chemical and heat stability preventing deactivation and decomposition, as well as its high activity as a Lewis acid catalyst in Michael-type reactions [16]. Also, a series of mononuclear para-substituted [PdIIX(NCN)] complexes was synthesized to study the electronic influence of the para substituent, the anchoring point for immobilization, on the Lewis-acidic palladium centres by means of catalysis and DFT calculations [17]. This was done to estimate beforehand any uncontrolled influences from these para functionalities on the catalytic activity of the pincer moiety when incorporated in a macromolecular complex. From these results, it became clear that in general the para substituents only slightly affect the catalytic activity of the palladium(II) centres. Thus, the pincer moiety possesses all desired properties to be incorporated into a recyclable macromolecular homogeneous catalyst and which also is stable under continuous operating conditions thereby retaining the catalytic activity of the non-supported pincer-metal complex.
Nanosize molecules with shape-persistent cores of types 3a–6a (Fig. 4, M = Pt, L = halide) were synthesized in order to investigate the influence of size and geometry on the retention rates of these macromolecules by MPF-60 and MPF-50 nanofiltration membranes [13]. As reference, the mononuclear [PtBr(NCN)] complex 7a was also tested in a nanofiltration membrane reactor [18]. For the retention experiments the [PtX(NCN)] moiety (instead of the catalytically active Pd-analogue) was used because exposing these complexes in solution to gaseous SO2 results in an instantaneous colour change from colourless to deep orange due to the formation of a penta-coordinated platinum η1-SO2 complex [14d, 19]. As a consequence, UV/Vis spectroscopy can be used to conveniently determine the concentration of the various complexes in both retentate and filtrate. To obtain impressions of the size and molecular structures of complexes 3a–7a, molecular modelling studies were performed on these platinum complexes. While in complexes 3a and 4a the organometallic sites are part of the shape-persistent core, 5a and 6a possess a more flexible organometallic shell around a rigid core [13]. Compounds 3a, 4a, and 7a are rather flat two-dimensional structures while 5a possesses a spherically shaped, three-dimensional geometry. Despite the rigid two-dimensional core, 6a exerts a three-dimensional structure due to the more flexible organometallic shell at its periphery.

Shape-persistent nanosize complexes.
The nanofiltration experiments were performed in a continuously operating high-pressure-membrane-reactor equipped with the MPF-60 or MPF-50 nanofiltration membrane. The concentrations of the multi(NCN-Pt) complexes in both the permeate and the retentate were determined and the resulting retention rates of the complexes are summarized in Table 1 [13]. With the MPF-60 membrane, the concentration of dodecakis(NCN-Pt) 6a in the permeate was below the detection limit of UV/Vis spectroscopy, i.e. a retention rate higher than 99.9%. The three-dimensional octakis(NCN-Pt) 5a showed retention of 99.5%. The two-dimensional phenylene-extended tris(pincer) 4a had a retention rate of 98.7%, while for the tris(pincer) complex 3 retention dropped below the 94%. The mononuclear NCN-pincer complex showed a retention rate of 82.4% only; the catalyst is completely washed out of the reactor within 30 cycles, which is far from being sufficient for continuous catalysis.
Retention rates of the various platinated NCN-pincer complexes
Compound | Ra (%) (MPF-60) | Ra (%) (MPF-50) |
3 | 93.9 | n.d. |
4 | 98.7 | 97.6 |
5 | 99.5 | n.d. |
6 | > 99.9 | 99.9 |
7 | 82.4 | n.d. |
To further investigate the concept of shape-persistence in the core of the macromolecular catalysts for attaining optimal retentions, the retention rates of 4a and 6a were related to the calculated molecular volumes of these complexes. The same procedure was performed with the flexible G0–Ni4, 1, and G1–Ni12, 2, carbosilane dendrimers (Fig. 1) [10]. For 4a and 6a, molecular volumes of 1.2 × 103 and 4.4 × 103 Å, respectively, were derived by molecular modelling studies while the G0–Ni4 and the G1–Ni12 dendrimers 1 and 2 possess molecular volumes of 1.7 × 103 and 5.3 × 103 Å3, respectively [13]. Earlier studies with the MPF-50 membrane yielded rates of retention of 97.4% for 1 and 99.8% for 2, respectively. Thus, although 4a (R = 97.7%) and 6a (R = 99.9%) possess smaller molecular volumes as compared to G0– 1 and G1–dendrimer 2, respectively, the shape-persistent materials 4a and 6a are retained more efficiently by the nanofiltration membranes than the more flexible dendritic systems. Although these differences in retention seem rather small, under continuously operating reaction conditions, such small differences (between 0.1 and 0.5%) already have a significant impact [2].
2.3 Application in a nanofiltration membrane reactor under continuous reaction conditions
For application as homogeneous catalysts in the double Michael reaction between methyl vinyl ketone (MVK) and ethyl α-cyanoacetate complexes 3b–7b were synthesized (Fig. 4, M = Pd, L = OH2(BF4)) [16, 17]. Compared to the catalytic activity of the reference monometallic catalyst 7b, it became clear that the catalytic activities per PdII centre for the multi(NCN-Pd) catalysts 3b–5b were similar, indicating that all PdII centres act as independent catalytic sites [17]. This implies an efficient use of all catalytic sites during catalysis and once again underlines that the Lewis acid catalytic activity is almost independent of the para functionality of the pincer moiety. Interestingly, dodecakis(NCN-Pd) 6b showed an almost threefold increase in catalytic activity per PdII centre which is proposed to be due to cooperative effects between different catalytic sites, since the palladium centres are much closer together than in the other shape-persistent compounds [17,20].
Dodecakis(NCN-Pd) complex 6b was applied as a homogeneous catalyst in the double Michael reaction between MVK and ethyl α-cyanoacetate under continuous reaction conditions in a nanofiltration membrane reactor [20]. Due to its macromolecular dimension, the catalyst is retained in the reactor during catalysis (R = 99.5% determined by ICP–AAS). Complex 6b was found to be stable under the continuous reaction conditions as a constant activity was obtained at prolonged reaction times (26 h, 65 exchanged reactor volumes). The total turnover number of the catalyst was thereby increased by a factor greater than 40 from 80 (batch) up to > 3000 mol molPd–1 [20]. Thus, with a number of important conditions fulfilled, i.e. high rates of retention, high catalyst stability and solvent resistant membranes, membrane technology is very promising for extended application of homogeneous catalysts in industrial processes.
3 Outlook
An increasing number of macromolecular homogeneous catalysts is reported that show high retentions by NF-membranes [2]. In future, for NF-membrane technology to be applicable on a large industrial scale, research should be focused on the durability of the catalyst during catalysis. The endurance of many homogeneous catalysts, in contrast to heterogeneous catalysts, does not yet meet the requirements needed for long running continuous catalytic processes. For a broader application of homogeneous catalysts under continuous reaction conditions, both improvement of the membrane technology (especially more resistant membranes) and finding more catalytic systems that possess the required properties such as high retention rates and catalyst stability are of crucial importance. Furthermore, developing recyclable homogeneous catalysts based on a variety of different catalytic units is also desirable, since it allows the application of a broad range of selective organic transformations in a nanofiltration membrane reactor, the first step toward large-scale continuous processes.
4 Conclusions
Comparison between shape-persistent complexes and flexible carbosilane dendrimers of similar nanosize dimensions (according to molecular modelling), resulted in higher retentions for the shape-persistent complexes. Especially, by relating the molecular volumes of the various complexes to the retention rates, it became clear that shape-persistence is advantageous for obtaining optimal retentions by nanofiltration membranes. Therefore, for future development and application in the area of homogeneous catalyst recycling by nanofiltration membranes it is advisable to use macromolecular catalysts with a high degree of shape-persistence in the organic backbone.
Acknowledgements
We thank Mr. N. Ronde and Prof. Dr. D. Vogt (Technical University Eindhoven) for performing the nanofiltration experiments.