1 Introduction
Phenanthrenes belong to an important skeleton of organic compounds owing to their core structure in natural products [1–5]. A great number of these alkaloids exhibit interesting biological activities such as anti-inflammatory [1,6,7], anticancer [4,8,9], antimicrobial [10–12], or antiviral [13–15]. N-(2,3-Methylenedioxy-6-methoxyphenanthr-9-ylmethyl)-4′-cyanopiperidine, 1 (Fig. 1), is well known for its inhibition of the growth of cancer cells [16]. Moreover, phenanthrene is of great interest to organic chemists because of its important properties either in materials science based on their photoconducting, photochemical, and electroluminescent properties [17–24].
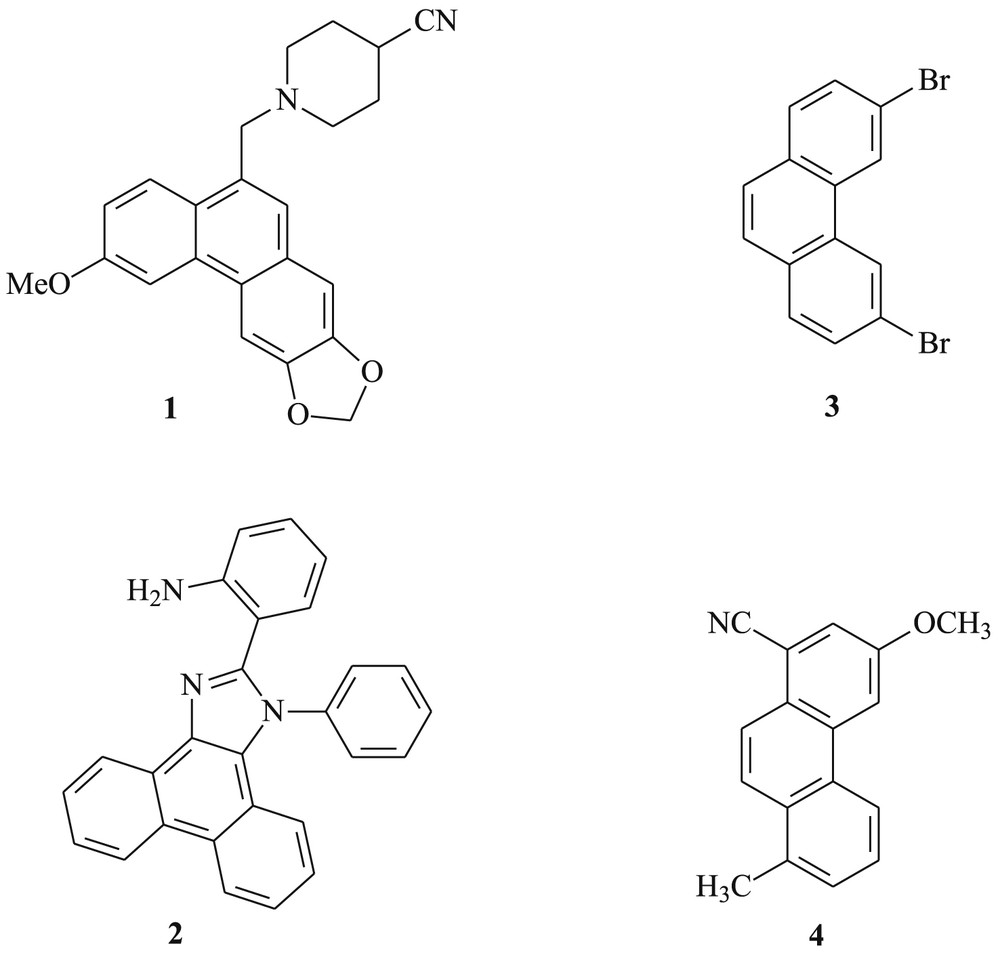
Representative examples of phenanthrene derivatives.
During the past few years, conjugated polymers and oligomers based on various aromatic building blocks have received considerable attention, owing to their intriguing photoluminescent and electroluminescent properties as well as their promising applications in the field of organic light emitting diodes (OLEDs) [25–30]. Phenanthrene and its derivatives, as their physical properties, including UV–vis, photoluminescence, thermal, and electrochemistry, have been extensively studied and have demonstrated that these compounds could serve as emitters for OLEDs [31–39].
Among the reported work, Nayak [40] reported the synthesis of novel class of blue-green fluorescent phenanthromidazoles like 2-(1-phenyl-1H-phenanthro[9,10,d]imidazol-2-yl)aniline 2 and reported on its optical, electrochemical, and thermal properties.
For the last several decades, much attention has been focused on the development of synthetic methods for the synthesis of phenanthrene derivatives. The most practical and widely used route to the synthesis of these compounds is the photocyclization of stilbenes [41–44], as it is well suited to the laboratory scale. Our research group has previously reported the synthesis of 3,6-dibromophenanthrene 3, using a photochemical procedure, and described its use in the synthesis of a helical optically active phosphine [41]. More recently, Mallory et al. [43] prepared 3-methoxy-8-methylphenanthrene-1-carbonitrile 4 using the same approach. Several nonphotochemical approaches have been investigated, among them are Diels–Alder reaction [45], Friedel–Crafts reaction [46,47], the photochemical procedure radical cyclizations [48], ring-closing metathesis [49–52]. In addition, complicated precursors, expensive and sensitive metallic catalysts, or harsh reaction conditions were needed for the synthesis of aromatic compounds [53–55]. Phenanthrene synthesis via photocyclodehydrogenation of stilbene derivatives still represents a simple and efficient synthetic method.
In this article, we describe the synthesis and characterization of new phenanthrene derivatives with cyano groups at selected positions on the aromatic rings. Our synthetic approach relies on the easy preparation of cyanodiarylethylenes via Knoevenagel reaction, which are then converted into phenanthrenes through the classical photocyclodehydrogenation.
2 Results and discussion
In the first part of our work, we have used the Knoevenagel condensation for the synthesis of several functionalized 1,2-diarylethylenes containing the powerful electron-withdrawing cyano group, using a simple and efficient route shown in Scheme 1. First, we have carried the reaction between arylacetonitriles 5–6 and the aromatic aldehydes 7a–e in dry methanol, in the presence of sodium methoxide as a base at room temperature, to obtain the resulting 1,2-diarylethylenes 8a–i in 51–85% yield (Scheme 1, Table 1).
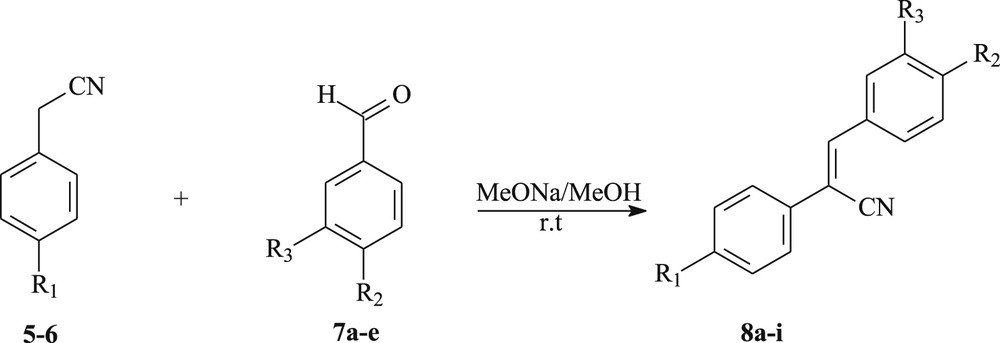
Synthetic pathway for the synthesis of 1,2-diarylethylenes 8a–i.
Chemical yields of 1,2-diarylethylenes 8a–i.
1,2-Diarylethylene | R1 | R2 | R3 | Yield (%)a |
8a | H | Br | H | 85 |
8b | H | OCH3 | H | 76 |
8c | H | CN | H | 82 |
8d | H | OCH3 | OCH3 | 75 |
8e | H | H | OCH3 | 73 |
8f | CH3 | Br | H | 71 |
8g | CH3 | OCH3 | H | 75 |
8h | CH3 | CN | H | 78 |
8i | CH3 | OCH3 | OCH3 | 74 |
a Isolated yields.
To prepare a novel and highly valuable polysubstituted phenanthrenes, we have envisaged to subject the resulting diarylethylenes for photocyclization. Thus, 500 mg of each olefin 8 have been dissolved in 1.2 L of toluene, and then irradiated using a 500 W high-pressure mercury immersion lamp, in the presence of a stoichiometric amount of iodine as the oxidizing agent and an excess of propylene oxide as a hydrogen iodide scavenger to produce the corresponding phenanthrene. This allowed us to obtain phenanthrenes P1–P9 in 51–83% yield (Scheme 2, Table 2).
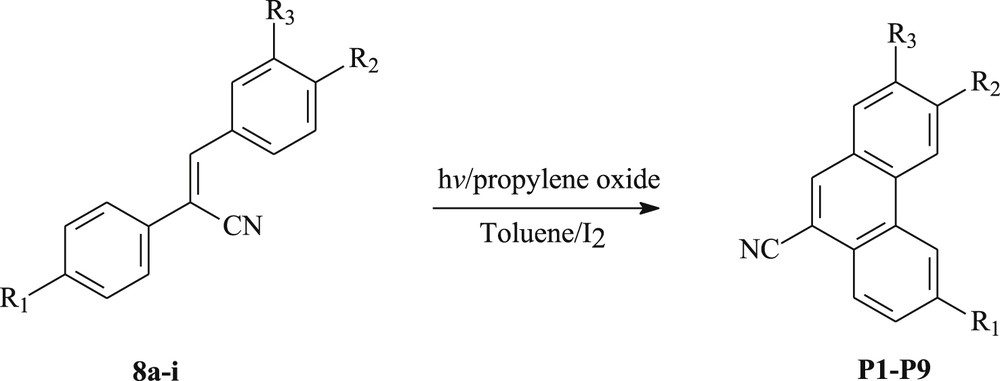
Photocyclization of 1,2-diarylethylenes 8a–i into phenanthrenes P1–P9.
Chemical yields of cyanophenanthrenes P1–P9.
Phenanthrene | R1 | R2 | R3 | Yield (%)a |
P1 | H | Br | H | 70 |
P2 | H | OCH3 | H | 75 |
P3 | H | CN | H | 84 |
P4 | H | OCH3 | OCH3 | 65 |
P5 | H | H | OCH3 | 62 |
P6 | CH3 | Br | H | 83 |
P7 | CH3 | OCH3 | H | 76 |
P8 | CH3 | CN | H | 75 |
P9 | CH3 | OCH3 | OCH3 | 72 |
a Isolated yields.
To extend the scope of these reactions, a new derivative of cyclopenta[a]naphthalene has been prepared according to the same synthetic approach. Our procedure uses 2-(p-tolyl)acetonitrile 9 and thiophene-2-carbaldehyde 10 as key building blocks for the synthesis olefin 8j, which is then converted to 5-methylnaphtho[2,1-b]thiophene-8-carbonitrile P10 by photolysis (Scheme 3). The 1H NMR data of compound P10 showed that proton H9 is manifested in the form of a desheilded singlet at 8.24 ppm, a doublet at 7.98 ppm is ascribed to H3, and H4 takes the form of a singlet at 8.14 ppm, which is observed in the most desheilded aromatic area.
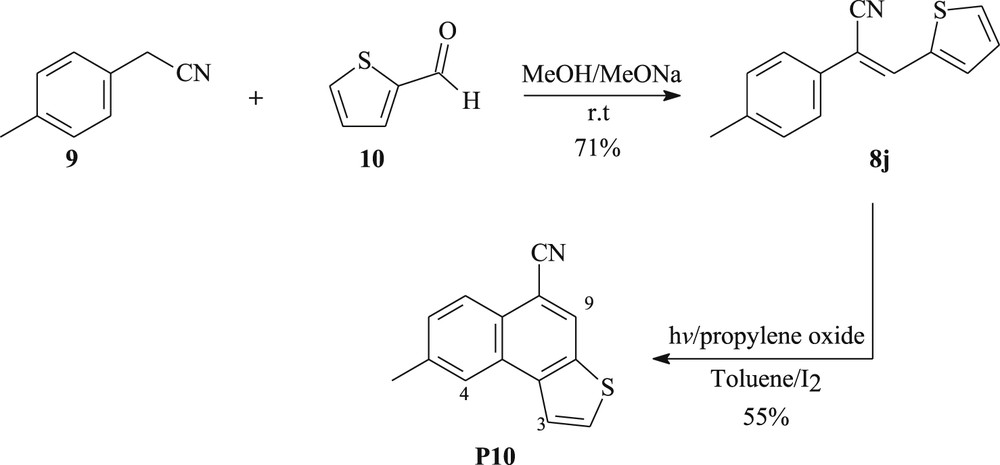
Synthetic pathway for the synthesis of compound P10.
The optical properties of the target cyanophenanthrenes have been investigated using UV–vis absorption spectroscopy in dilute chloroform solutions (7 × 10−5 M) as depicted in Fig. 2. The UV–vis spectrum of these compounds exhibited a strong absorption in the region of 250–450 nm. These absorption bands are associated with π–π∗ and n–π∗ electronic transitions. The gap energy (Eg) values of the phenanthrenes determined from the absorption edge of the solution spectra are given in Table 3.
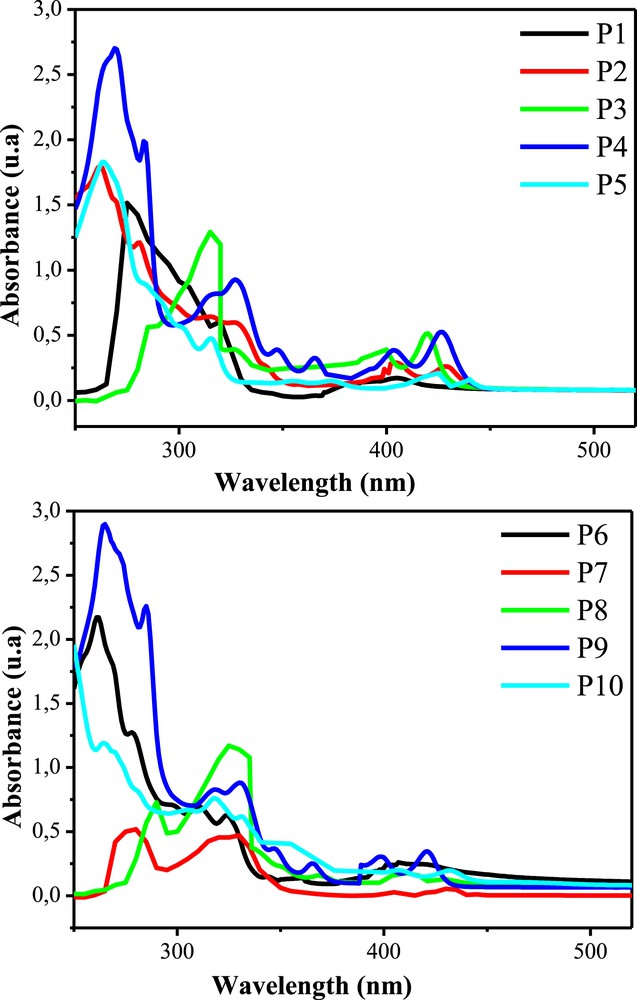
UV–vis absorption spectrum of cyanophenanthrenes P1–P10 in dilute chloroform solutions (7 × 10−5 M).
Optical properties of cyanophenanthrenes P1–P10.
Compound | a (nm) | λonset (nm) | Eg-opb (eV) |
P1 | 274 | 441 | 2.81 |
P2 | 261 | 446 | 2.78 |
P3 | 315 | 430 | 2.88 |
P4 | 268 | 441 | 2.81 |
P5 | 264 | 454 | 2.73 |
P6 | 268 | 464 | 2.67 |
P7 | 279 | 441 | 2.81 |
P8 | 325 | 428 | 2.89 |
P9 | 265 | 432 | 2.87 |
P10 | 264 | 446 | 2.78 |
a Absorption maxima measured in a chloroform solution (7 × 10−5 mol L−1) at room temperature.
b The optical gap (Eg-op) was estimated from the onset point of the absorption spectra: Eg-op = 1240/λonset.
To investigate the redox behavior of phenanthrenes and to estimate their highest occupied molecular orbital (HOMO) and lowest unoccupied molecular orbital (LUMO) energy levels, cyclic voltammetry (CV) was applied to the materials. Knowledge of the HOMO and LUMO energy levels is of crucial importance for the selection of the cathode and anode materials for OLED devices. The use of CV analysis is of good reliability as the electrochemical processes probed therein are similar to those involved in charge injection and transport processes in OLED devices.
The cyanophenanthrenes P1-P10 have been scanned both positively and negatively, in solutions of tetra-n-butylammonium perchlorate (n-Bu4NClO4) in acetonitrile (0.10 M) with a scan rate of 50 mV s−1 at room temperature. The obtained cyclic voltammogram of P1 is shown in Fig. 3 as an example and the electrochemical properties of all products are summarized in Table 4. We found that the oxidation and reduction processes of the phenanthrenes were irreversible. The onset potentials for oxidation were 1.53, 1.48, 2.13, 2.09, 1.71, 1.63, 1.60, 2.19, 2.20, and 2.17 V (vs saturated calomel electrode [SCE]) for cyanophenanthrenes P1–P10, respectively, whereas the onset potentials for reduction were −1.09, −1.07, −0.54, −0.57, −0.85, −0.38, −0.83, −0.60, −0.56, and −0.58 V (vs SCE), respectively (Table 4). From the onset potentials of the oxidation and reduction processes, the electrochemical band gaps (Eg) could be calculated as follows [56]:
EHOMO (ionization potential) = −(Vonset-ox − VFOC + 4.8) |
ELUMO (electron affinity) = −(Vonset-red − VFOC + 4.8) |
Eg-el = (ELUMO − EHOMO) eV |
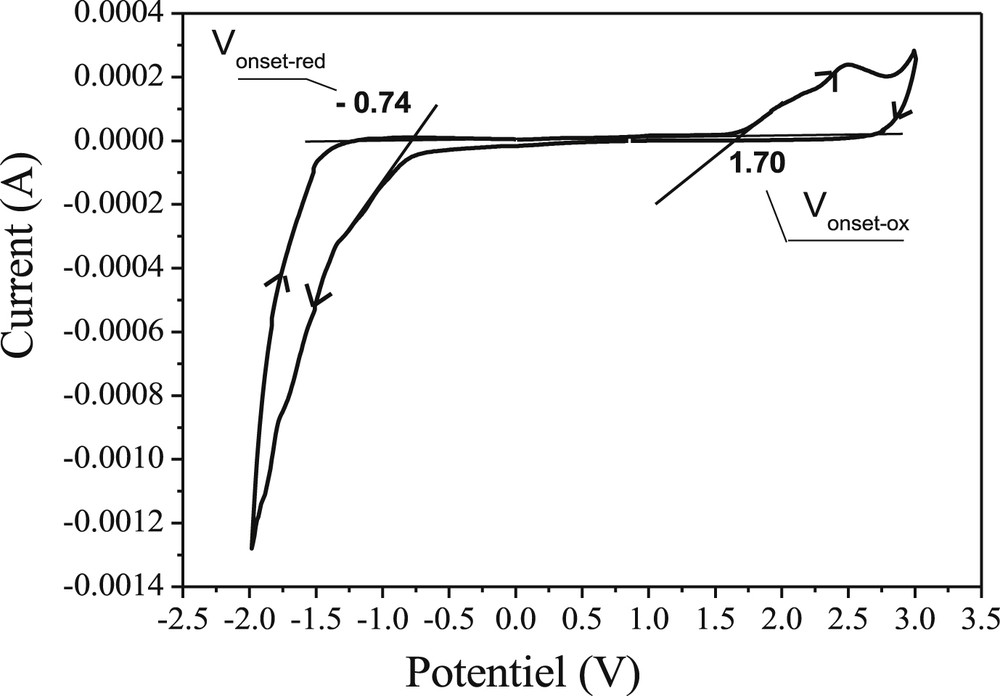
Cyclic voltammogram of P1 in 0.1 M (n-Bu)4NClO4/acetonitrile at a scanning rate of 50 mV s−1.
Electrochemical properties of cyanophenanthrenes P1–P10.
Compound | Vonset-ox (V) | Vonset-red (V) | EHOMOa (eV) | ELUMOb (eV) | Eg-elc (eV) |
P1 | 1.53 | −1.09 | −5.65 | −3.03 | 2.62 |
P2 | 1.48 | −1.07 | −5.60 | −3.05 | 2.55 |
P3 | 2.13 | −0.54 | −6.25 | −3.58 | 2.67 |
P4 | 2.09 | −0.57 | −6.21 | −3.55 | 2.66 |
P5 | 1.71 | −0.85 | −5.83 | −3.27 | 2.56 |
P6 | 1.63 | −0.38 | −5.75 | −3.20 | 2.55 |
P7 | 1.60 | −0.83 | −5.72 | −3.29 | 2.43 |
P8 | 2.19 | −0.60 | −6.31 | −3.52 | 2.79 |
P9 | 2.20 | −0.56 | −6.32 | −3.56 | 2.76 |
P10 | 2.17 | −0.58 | −6.29 | −3.54 | 2.75 |
a Calculated from the oxidation potential.
b Calculated from the reduction potential.
c Band gap was calculated from the difference between HOMO and LUMO.
The estimated EHOMO and ELUMO values of products P1–P10 are given in Table 4. The slight difference between the optical and electrochemical band gap (0.03–0.38 eV) is attributed to the interface barrier between the electrode and the helicene, and the exciton binding energy [57,58].
A comparison of these compounds revealed that the HOMO energy levels were slightly different (Fig. 4). However, a higher electron affinity was observed for compound P7. The LUMO levels of these π-conjugated tricyclic systems were comparable with that of bathocuproine (BCP). BCP is widely used as an electron-injection hole-blocking layer in OLEDs [59,60]. It prohibits excitons diffusing toward the Al electrode where they would otherwise be quenched. However, it is known that BCP crystallizes quickly, which results in degradation of the device performance [61,62]. For this reason, it is desirable to replace BCP by other materials in such devices. In fact, the fused tricyclic systems P1–P10 might be good replacements for BCP and for the elaboration of multilayer devices.
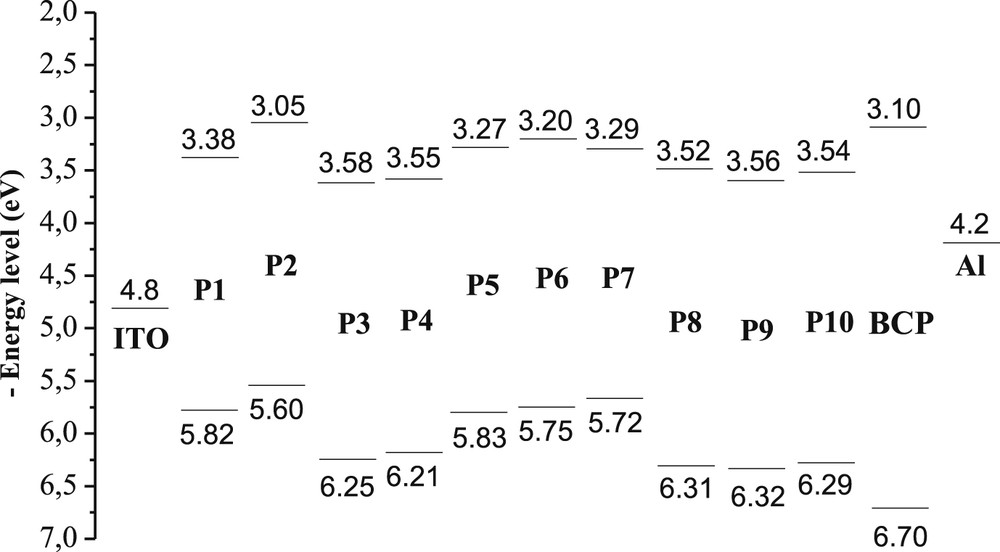
Comparison of the HOMO–LUMO energy levels of P1–P10 and those of BCP, indium tin oxide (ITO), and aluminum.
3 Conclusion
We have described a straightforward method for the preparation of new cyanophenanthrenes in good yields under mild conditions. The photophysical and electrochemical behaviors of these compounds indicated that they might be suitable candidates for electron-injection hole-blocking layers. Work is currently under way toward the application of these materials in multilayer devices.
4 Experimental section
4.1 General
All reagents and solvents used in this work are available commercially and used without further purification. The reactions were monitored by thin-layer chromatography using commercial silica-gel plate 60F254. Melting points were measured on a Bibby Scientific Stuart Digital, Advanced, SMP30. The 1H NMR and 13C NMR spectra were recorded in a Bruker AC-300 and were obtained in CDCl3 as a solvent with tetramethylsilane as the internal reference. UV–vis spectra were recorded on a spectrophotometer UV-1600PC. CV measurements have been conducted using a standard one-compartment, three-electrode electrochemical cell using a CH-660B potentiostat. Investigations have been carried out in millimolar anhydrous 1,2-dichlorobenzene solutions containing tetra-n-butylammonium perchlorate (0.5 M) as a supporting electrolyte. A platinum wire has been used as the counter electrode. An ALS Co. Ltd Ag+/Ag electrode ([Ag+] = 10−2 M in CH3CN) has been used as a reference. This reference electrode was calibrated with respect to the formal potential of the ferrocenium/ferrocene (Fc+/Fc) couple in CH3CN. All potentials values have been converted toward SCE using E versus SCE = E versus Ag/Ag+ (10−2) + 298 mV. Yields refer to quantities obtained after chromatography.
4.2 General procedure for the preparation of the α,β-unsaturated nitriles (8a–i)
A mixture of arylacetonitrile 5 or 6 (12.9 mmol, 1 equiv) and aldehyde 7 (12.9 mmol, 1 equiv) in dry methanol (30 mL) was stirred at 0 °C for 5 min. Then, sodium methoxide (1.2 g, 25.9 mmol) was added in small portions, and the mixture was stirred for 30 min at 0 °C and then for 12 h at room temperature. The precipitate was collected using a sintered glass filter, washed with MeOH and then with water, and dried.
4.2.1 (Z)-3-(p-Bromophenyl)-2-phenylacrylonitrile (8a)
White solid, 85%, mp = 99–101 °C; 1H NMR (300 MHz, CDCl3): δ (ppm): 7.38–7.48 (m, 4H); 7.59 (d, J = 8.4 Hz, 2H); 7.66 (d, J = 7.2 Hz, 2H); 7.74 (d, J = 8.1 Hz, 2H); 13C NMR (75 MHz, CDCl3): δ (ppm): 112.14 (C); 117.08 (CN); 124.31 (CBr); 125.52 (2CH); 128.60 (2CH); 128.91 (CH); 130.08 (2CH); 131.71 (2CH); 132.15 (C); 133.75 (C); 140.10 (CH).
4.2.2 (Z)-3-(p-Methoxyphenyl)-2-phenylacrylonitrile (8b)
White solid, 76%, mp = 93–95 °C; 1H NMR (300 MHz, CDCl3): δ (ppm): 3.89 (s, 3H, OCH3); 6.99 (d, J = 8.7 Hz, 2H); 7.38–7.48 (m, 4H); 7.66 (d, J = 8.7 Hz, 2H); 7.89 (d, J = 8.7 Hz, 2H); 13C NMR (75 MHz, CDCl3): δ (ppm): 54.88 (OCH3); 108.41 (C); 113.93 (2CH); 117.89 (CN); 125.29 (2CH); 126.11(C); 128.19 (CH); 128.45 (2CH); 130.63 (2CH); 134.47 (C); 141.29 (CH); 160.99 (C).
4.2.3 (Z)-3-(p-Cyanophenyl)-2-phenyl)acrylonitrile (8c)
White solid, 82%, mp = 66–68 °C; 1H NMR (300 MHz, CDCl3): δ (ppm): 7.46–7.50 (m, 3H); 7.53 (s, 1H, Hvinyl); 7.69–7.76 (m, 4H); 7.95 (d, J = 8.1 Hz, 2H); 13C NMR (75 MHz; CDCl3): δ (ppm): 113.14 (C); 115.04 (C); 116.53 (CN); 117.57 (CN) 125.73 (2CH); 128.74 (2CH); 129.05 (2CH); 129.56 (CH); 132.08 (2CH); 133.16 (C); 137.37 (C); 138.78 (CH).
4.2.4 (Z)-3-(3′,4′-Dimethoxyphenyl)-2-phenylacrylonitrile (8d)
Yellow solid, 75%, mp = 91–93 °C; 1H NMR (300 MHz, CDCl3): δ (ppm): 3.97 (s, 3H, OCH3); 4.00 (s, 3H, OCH3); 6.94 (d, J = 8.4 Hz, 1H); 7.36–7.48 (m, 5H); 7.66 (d, J = 7.2 Hz, 2H); 7.74 (d, J = 1.8 Hz, 1H); 13C NMR (75 MHz, CDCl3): δ (ppm): 55.52 (2 OCH3); 108.02 (C); 110.26 (CH); 110.45 (CH); 118.19 (CN); 123.92 (CH); 125.30 (2CH); 126.26 (C); 128.29 (CH); 128.53 (2CH); 134.33 (C); 141.64 (CH); 148.57 (CO); 150.67 (CO).
4.2.5 (Z)-3-(3′-Methoxyphenyl)-2-phenylacrylonitrile (8e)
Yellow solid, 73%, mp = 40–42 °C; 1H NMR (300 MHz, CDCl3): δ (ppm): 3.88 (s, 3H, OCH3); 7.00 (dd, J1 = 2.1 Hz, J2 = 7.8 Hz, 1H); 7.36 (d, J = 7.8 Hz, 1H); 7.40–7.51 (m, 6H); 7.68 (d, J = 7.8 Hz, 1H); 7.69 (d, J = 8.4 Hz, 1H); 13C NMR (75 MHz, CDCl3): δ (ppm): 54.91 (OCH3); 111.37 (C); 113.00 (CH); 116.52 (CH); 117.56 (CN); 121.71 (CH); 125.54 (2CH); 128.57 (2CH); 128.74 (CH); 129.44 (CH); 133.98 (C); 134.47 (C); 141.69 (CH); 159.37 (CO).
4.2.6 (Z)-3-(p-Bromophenyl)-2-(p-methylphenyl)acrylonitrile (8f)
White solid, 71%, mp = 107–109 °C; 1H NMR (300 MHz, CDCl3): δ (ppm): 2.40 (s, 3H, CH3); 7.23 (d, J = 8.1 Hz, 2H); 7.41 (s, 1H, Hvinyl); 7.54–7.60 (m, 4H); 7.72 (d, J = 8.7 Hz, 2H); 13C NMR (75 MHz, CDCl3): δ (ppm): 20.61 (CH3); 112.11 (C); 117.17 (CN); 124.04 (C); 125.39 (2CH); 129.28 (2CH); 130.00 (2CH); 130.96 (C); 131.65 (2CH); 132.32 (C); 139.02 (CH); 139.19 (C).
4.2.7 (Z)-3-(p-Methoxyphenyl)-2-(p-tolyl)acrylonitrile (8g)
Yellow solid, 75%, mp = 95–97 °C; 1H NMR (300 MHz, CDCl3): δ (ppm): 2.39 (s, 3H, CH3); 3.87 (s, 3H, OCH3); 6.97 (d, J = 8.7 Hz, 2H); 7.23 (d, J = 8.1 Hz, 2H); 7.42 (s, 1H, Hvinyl); 7.54 (d, J = 8.1 Hz, 2H); 7.86 (d, J = 8.7 Hz, 2H); 13C NMR (75 MHz, CDCl3): δ (ppm): 20.56 (CH3); 54.86 (OCH3); 108.42 (C); 113.89 (2CH); 117.98 (CN); 125.17 (2CH); 126.26 (C); 129.14 (2CH); 130.50 (2CH); 131.65 (C); 138.33 (C); 140.31 (CH); 160.82 (CO).
4.2.8 (Z)-3-(p-Cyanophenyl)-2-(p-tolyl)acrylonitrile (8h)
White solid, 78%, mp = 190–192 °C; 1H NMR (300 MHz, CDCl3): δ (ppm): 2.37 (s, 3H, CH3); 7.30 (d, J = 8.7 Hz, 2H); 7.50 (s, 1H, Hvinyl); 7.61 (d, J = 8.1 Hz, 2H); 7.76 (d, J = 8.4 Hz, 2H); 7.97 (d, J = 8.1 Hz, 2H); 13C NMR (75 MHz, CDCl3): δ (ppm): 21.17 (CH3); 113.41 (C); 115.48 (C); 117.13 (CN); 118.12 (CN); 126.11 (2CH); 129.46 (2CH); 129.91 (2CH); 130.88 (C); 132.53 (2CH); 138.05 (CH); 138.17 (C); 140.51 (C).
4.2.9 (Z)-3-(3′,4′-Dimethoxyphenyl)-2-(p-tolyl)acrylonitrile (8i)
Yellow solid, 74%, mp = 95–97 °C; 1H NMR (300 MHz, CDCl3): δ (ppm): 2.41 (s, 3H, CH3); 3.96 (s, 3H, OCH3); 3.99 (s, 3H, OCH3); 6.94 (d, J = 8.1 Hz, 1H); 7.26 (d, J = 7.8 Hz, 2H); 7.37 (d, J = 8.4 Hz, 1H); 7.42 (s, 1H, Hvinyl); 7.57 (d, J = 7.8 Hz, 2H); 7.72 (s, 1H); 13C NMR (75 MHz, CDCl3): δ (ppm): 21.05 (CH3); 55.97 (OCH3); 56.05 (OCH3); 108.94 (C); 111.21 (CH); 111.24 (CH); 118.60 (CN); 124.11 (CH); 125.67 (2CH); 127.03 (C); 129.65 (2CH); 132.11 (C); 138.88 (C); 141.03 (CH); 149.27 (C); 151.19 (C).
4.2.10 (Z)-3-(Thiophen-2-yl)-2-(p-tolyl)acrylonitrile (8j)
Orange solid, 71%, mp = 125–127 °C; 1H NMR (300 MHz, CDCl3): δ (ppm): 2.39 (s, 3H, CH3); 7.12–7.15 (m, 1H); 7.22 (d, J = 8.1 Hz, 2H); 7.51–7.55 (m, 3H); 7.60 (s, 1H, Hvinyl); 7.65 (d, J = 3.6 Hz, 1H); 13C NMR (75 MHz; CDCl3): δ (ppm): 20.60 (CH3); 108.08 (C); 117.62 (CN); 125.10 (2CH); 127.63 (CH); 129.01 (CH); 129.25 (2CH); 130.71 (C); 131.21 (CH); 132.59 (CH); 137.65 (C); 138.65 (C).
4.3 General procedure for the photocyclization of α,β-insaturated nitriles into phenanthrenes
To a solution of olefin 8 (2 mmol) in toluene (1.2 L) was added iodine (558 mg, 1.1 equiv). The solution was degassed for 15–30 min and then propylene oxide (50 equiv) was added. Irradiation was performed using a falling-film photoreactor and a high-pressure Hg-vapor lamp (500 W, Hanovia). A flow of argon was maintained throughout the irradiation. The reaction was monitored by thin-layer chromatography. After completion, the solvent was removed under reduced pressure and the crude residue was purified by silica gel column chromatography with cyclohexane/EtOAc (80:20) as the eluent. Spectroscopic data for the phenanthrene derivatives are given subsequently.
4.3.1 3-Bromophenanthrene-9-carbonitrile (P1)
Yellow solid, 70%, mp = 176–178 °C; 13C NMR (300 MHz, CDCl3): δ (ppm): 7.73–7.79 (m, 4H); 8.14 (s, 1H, H10); 8.25–8.28 (m, 1H); 8.54–8.57 (m, 1H); 8.74 (s, 1H, H5); 13C NMR (75 MHz, CDCl3): δ (ppm): 109.44 (C); 117.04 (CN); 122.60 (CH); 124.15 (C); 125.36 (CH); 125.68 (CH); 127.78 (C); 128.04 (CH); 128.28 (CH); 128.38 (C); 128.62 (C); 130.25 (CH); 130.45 (CH); 132.49 (C); 134.26 (CH); HRMS (MALDI-TOF) Calcd for C15H8BrN [M]+, 280.9840; found, 280.9836.
4.3.2 3-Methoxyphenanthrene-9-carbonitrile (P2)
White solid, 75%, mp = 120–122 °C [63]; 1H NMR (300 MHz, CDCl3): δ (ppm): 4.04 (s, 3H, OCH3); 7.29 (dd, J1 = 2.1 Hz, J2 = 8.7 Hz, 1H); 7.71–7.75 (m, 2H); 7.82 (d, J = 9 Hz, 1H); 8.01 (s, 1H, H4); 8.16 (s, 1H, H10); 8.26–8.29 (m, 1H); 8.59–8.62 (m, 1H); 13C NMR (75 MHz, CDCl3): δ (ppm): 55.56 (OCH3); 104.35 (CH); 106.72 (C); 117.74 (CH); 118.15 (CN); 123.05 (CH); 124.57 (C); 126.12 (CH); 127.62 (CH); 128.13 (CH); 129.41 (C); 129.45 (C); 131.05 (CH); 133.65 (C); 135.17 (CH); 161.03 (C-3).
4.3.3 Phenanthrene-3,9-dicarbonitrile (P3)
Yellow solid, 84%, mp > 260 °C [64]; 1H NMR (300 MHz, CDCl3): δ (ppm): 7.86–7.88 (m, 3H); 8.03 (d, J = 8.4 Hz, 1H); 8.27 (s, 1H, H10); 8.36–8.39 (m, 1H); 8.68–8.71 (m, 1H, H5); 9.02 (s, 1H, H4); 13C NMR (75 MHz, CDCl3): δ (ppm): 112.89 (C); 113.10 (C); 117.03 (CN); 118.63 (CN); 123.12 (CH); 126.53 (CH); 128.28 (CH); 129.00 (CH); 129.17 (C); 129.44 (CH); 129.47 (CH); 130.47 (CH); 131.45 (C); 131.84 (C); 134.27 (CH).
4.3.4 2,3-Dimethoxyphenanthrene-9-carbonitrile (P4)
White solid, 65%, mp = 150–152 °C [65]; 1H NMR (300 MHz, CDCl3): δ (ppm): 4.05 (s, 3H, OCH3); 4.13 (s, 3H, OCH3); 7.22 (s, 1H, H1); 7.68–7.72 (m, 2H); 7.95 (s, 1H, H4); 8.10 (s, 1H, H10); 8.26 (d, J = 8.7 Hz, 1H); 8.51 (d, J = 8.7 Hz, 1H); 13C NMR (75 MHz, CDCl3): δ (ppm): 56.06 (OCH3); 56.11 (OCH3); 103.29 (CH); 107.21 (C); 108.82 (CH); 118.20 (CN); 122.53 (CH); 125.11 (C); 126.12 (CH); 127.09 (CH); 127.21 (C); 127.61 (CH); 128.58 (C); 129.40 (C); 134.16 (CH); 150.20 (CO); 152.02 (CO).
4.3.5 2-Methoxyphenanthrene-9-carbonitrile (P5)
White solid, 62%, mp = 171–173 °C; 1H NMR (300 MHz, CDCl3): δ (ppm): 3.97 (s, 3H, OCH3); 7.24–7.26 (t, J = 2.7 Hz, 1H, H1); 7.38–7.42 (dd, J1 = 2.7 Hz, J2 = 9 Hz, 1H, H3); 7.64–7.75 (m, 2H); 8.14 (s, 1H, H10); 8.24–8.27 (dd, J1 = 1.2 Hz, J2 = 7.8 Hz, 1H); 8.54–8.59 (m, 2H); 13C NMR (75 MHz, CDCl3) δ (ppm): 55.54 (OCH3); 109.12 (CH); 109.94 (C); 117.89 (CN); 120.48 (CH); 122.54 (CH); 124.43 (CH); 126.06 (CH); 126.16 (C); 127.04 (CH); 128.02 (C); 128.20 (CH); 130.20 (C); 131.24 (C); 134.84 (CH); 158.94 (C); HRMS (MALDI-TOF) Calcd for C16H11NO [M]+, 233.0840; found, 233.0836.
4.3.6 3-Bromo-6-methylphenanthrene-9-carbonitrile (P6)
White solid, 83%, mp = 186–188 °C; 1H NMR (300 MHz, CDCl3): δ (ppm): 2.66 (s, 3H, CH3); 7.58 (dd, J1 = 0.9 Hz, J2 = 8.1 Hz, 1H); 7.73 (d, J = 0.9 Hz, 2H); 8.07 (s, 1H, H10); 8.14 (d, J = 8.1 Hz, 1H); 8.32 (s, 1H, H4); 8.72 (s, 1H, H5); 13C NMR (75 MHz, CDCl3): δ (ppm): 21.55 (CH3); 109.22 (C); 117.18 (CN); 122.28 (CH); 123.81 (C); 125.25 (CH); 125.45 (CH); 126.57 (C); 127.90 (C); 128.40 (C); 129.95 (CH); 130.18 (CH); 130.24 (CH); 132.18 (C); 133.19 (CH); 138.28 (C); HRMS (MALDI-TOF) Calcd for C16H10NO [M]+, 294.9996; found, 294.9990.
4.3.7 3-Methoxy-6-methylphenanthrene-9-carbonitrile (P7)
White solid, 76%, mp = 180–182 °C; 1H NMR (300 MHz, CDCl3): δ (ppm): 2.65 (s, 3H, CH3); 4.06 (s, 3H, OCH3); 7.31 (dd, J1 = 2.4 Hz, J2 = 8.7 Hz, 1H); 7.53 (dd, J1 = 1.8 Hz, J2 = 8.1 Hz, 1H); 7.77 (d, J = 8.7 Hz, 1H); 7.92 (d, J = 2.4 Hz, 1H); 8.06 (s, 1H, H10); 8.11 (d, J = 8.1 Hz, 1H, H4); 8.32 (s, 1H, H5); 13C NMR (75 MHz, CDCl3): δ (ppm): 21.55 (CH3); 55.11 (OCH3); 103.46 (CH); 105.83 (C); 117.19 (CH); 117.98 (CN); 122.23 (CH); 124.10 (C); 125.38 (CH); 126.72 (C); 128.87 (C); 129.35 (CH); 130.50 (CH); 132.75 (C); 133.76 (CH); 137.17 (C); 160.23 (C-3); HRMS (MALDI-TOF) Calcd for C17H13NO [M]+, 247.0997; found, 247.0991.
4.3.8 6-Methylphenanthrene-3,9-dicarbonitrile (P8)
Yellow solid, 75%, mp = 193–195 °C; 1H NMR (300 MHz, CDCl3): δ (ppm): 2.69 (s, 3H, CH3); 7.67 (d, J = 8.1 Hz, 1H, H7); 7.83 (d, J = 8.1 Hz, 1H); 7.80 (d, J = 8.4 Hz, 1H); 8.16 (s, 1H, H10); 8.23 (d, J = 8.1 Hz, 1H, H2); 8.45 (s, 1H, H5); 8.98 (s, 1H, H4); 13C NMR (75 MHz, CDCl3): δ (ppm): 21.53 (CH3); 104.68 (C); 112.29 (C); 112.41 (C); 116.52 (CN); 118.01 (CN); 122.29 (CH); 125.81 (CH); 126.74 (C); 127.66 (CH); 128.22 (CH); 128.79 (C); 129.77 (CH); 130.58 (CH); 131.50 (C); 132.52 (CH); 139.34 (C); HRMS (MALDI-TOF) Calcd for C17H10N2 [M]+, 242.0843; found, 242.0837.
4.3.9 2,3-Dimethoxy-6-methylphenanthrene-9-carbonitrile (P9)
Yellow solid, 72%, mp = 194–196 °C; 1H NMR (300 MHz, CDCl3): δ (ppm): 2.66 (s, 3H, CH3); 4.06 (s, 3H, OCH3); 4.16 (s, 3H, OCH3); 7.20 (s, 1H, H1); 7.52 (d, J = 8.1 Hz, 1H); 7.91 (s, 1H, H10); 8.04 (s, 1H, H4); 8.15 (d, J = 8.4 Hz, 1H, H8); 8.27 (s, 1H, H5); 13C NMR (75 MHz, CDCl3): δ (ppm): 21.57 (CH3); 55.56 (OCH3); 55.65 (OCH3); 102.52 (CH); 106.41 (C); 108.11 (CH); 117.99 (CN); 121.65 (CH); 124.67 (C); 125.44 (CH); 126.02 (C); 126.31 (C); 128.43 (CH); 128.91 (C); 132.76 (CH); 137.20 (C); 149.39 (CO); 151.15 (CO); HRMS (MALDI-TOF) Calcd for C18H15NO2 [M]+, 277.1102; found, 277.1042.
4.3.10 5-Methylnaphtho[2,1-b]thiophene-8-carbonitrile (P10)
Yellow solid, 55%, mp = 143–145 °C; 1H NMR (300 MHz, CDCl3): δ (ppm): 2.63 (s, 3H, CH3); 7.53 (d, J = 8.4 Hz, 1H); 7.81 (d, J = 5.4 Hz, 1H, H2); 7.99 (d, J = 5.1 Hz, 1H, H3); 8.14 (s, 1H, H9); 8.20–8.24 (m, 2H); 13C NMR (75 MHz, CDCl3): δ (ppm): 21.30 (CH3); 106.29 (C); 117.64 (CN); 121.68 (CH); 123.01 (CH); 125.31 (CH); 126.48 (CH); 126.89 (C); 128.52 (C); 128.73 (CH); 129.81 (CH); 134.98 (C); 137.66 (C); 138.45 (C); HRMS (MALDI-TOF) Calcd for C14H9NS [M]+, 223.0455; found, 223.0449.
Acknowledgments
The authors are grateful to the “Direction générale de la recherche scientifique” (DGRS) of the Tunisian Ministry of Higher Education and Scientific Research for the financial support of this research.