1 Introduction
Helicenes are polycyclic aromatic hydrocarbons made of ortho-fused aromatic rings, forming an inherent regular helical shape, which arises from the steric hindrance between aromatic rings. The surprising combination of exhibiting full delocalization of π electrons with a chiral nonplanar structure has fascinated chemists from the early years of helicene research. Their specific backbone and their structural characteristics make them very stable toward acids, bases, and relatively high temperatures [1].
For many years, a synthetic chemistry research program to develop a new method or to design novel molecules for important photophysics measurements has been reported. Because of their inherent chirality, high optical stability, and their rigid helical framework, they have always brought a large interest from transversal disciplines such as liquid crystals [2], sensors [3], chiral catalysts [4] and ligands [5] for asymmetric synthesis and polymer synthesis [6,7] and molecular motors [8] as they have been used in biology [9].
Hence, the synthesis of new helical molecules remains a tough challenge and exciting field. Helical molecules bearing one or more cyano groups are of considerable interest because of their photophysical properties. The first example is 1,4-dicyano-13-methyl[5]helicene 1 (Fig. 1), which has been reported by Stammel et al. [10]. It has been synthesized in 33% overall yield through a multistep procedure. Sahasithiwat and co-workers [1,11] reported the synthesis of 7,8-dicyano-3,12-dimethoxy[5]helicene 2 and 3,12-dimethoxy-5,6,9,10-tetrahydro-7,8-dicyano[5]helicene 3 in three steps through the Diels–Alder reaction and used them for organic light-emitting diodes. Recently, Ito et al. [12] reported the synthesis of 5,10-dicyano[5]helicene 4 in 67% overall yield, through a double photocyclization reaction of 1,4-bis(2-cyano-2-phenylethenyl)benzene.
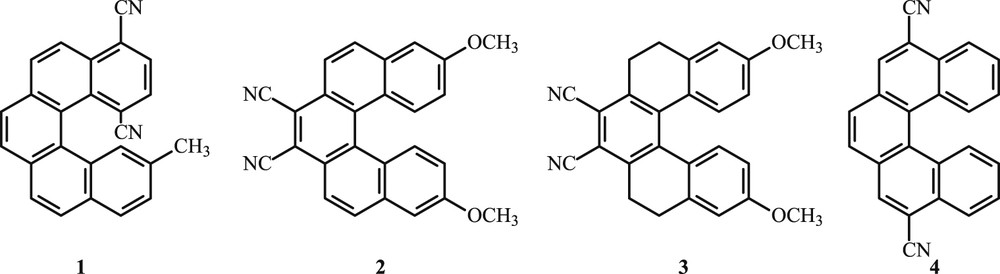
Representative examples of cyanopentahelicenes.
Photochemical reaction appears as a convenient method for the synthesis of large helicenes under mild reaction conditions. By using this method, the preparation of a target helicene from 2-(p-bromophenyl)-3-(m-methoxyphenyl)acrylonitrile should be feasible. In addition, the photochemical approach may sometimes fail with pentahelicenes even under the most drastic conditions. Indeed, attempts at the photochemical synthesis of pentahelicenes have led to benzo[ghi]perylenes as a result of overannulation [10,12].
In this study, a short and efficient synthetic strategy was developed for the synthesis of new pentahelicene. Using Knoevenagel condensation, palladium-catalyzed Heck coupling, and classical oxidative photocyclization reactions, we have successfully synthesized a new target polyfunctional pentahelicene. The method is efficient for the formation of helicenes because of the easy preparation of stilbene-type starting materials via Knoevenagel condensation.
The solubility of helicenes could be increased by adding selected functional groups containing polar unsaturated bonds such as cyano group, which improve the photophysical properties.
In our synthetic approach, a suitable phenanthrene moiety has been used as a key building block for the preparation of appropriately substituted α,β-unsaturated nitrile, then UV-light-promoted photocyclodehydrogenation remains the most widely used route to obtain our helically chiral pentacyclic system.
2 Results and discussion
The synthesis of the phenanthrene-like system 6 began with the Knoevenagel reaction of commercially available (p-bromophenyl)acetonitrile with 3-methoxybenzaldehyde using sodium methoxide as a base in dry methanol (Scheme 1). The reaction mixture was stirred for 4 h at room temperature to give the desired cyanostilbene 5 in 93% yield. The latter compound was irradiated in toluene in the presence of a stoichiometric amount of iodine as oxidizing agent and excess propylene oxide as hydrogen iodide scavenger, to provide the expected 6-bromo-2-methoxyphenanthrene-9-carbonitrile (6) in 68% yield. The ring closure of olefin 5 was not completely regioselective because 6-bromo-4-methoxyphenanthrene-9-carbonitrile (7) was isolated in 15% yield and identified as a byproduct in the reaction mixture (Scheme 1). Compounds 6 and 7 have different polarity and have been successfully separated by column chromatography on silica gel using cyclohexane/AcOEt (95:5) as the eluent.
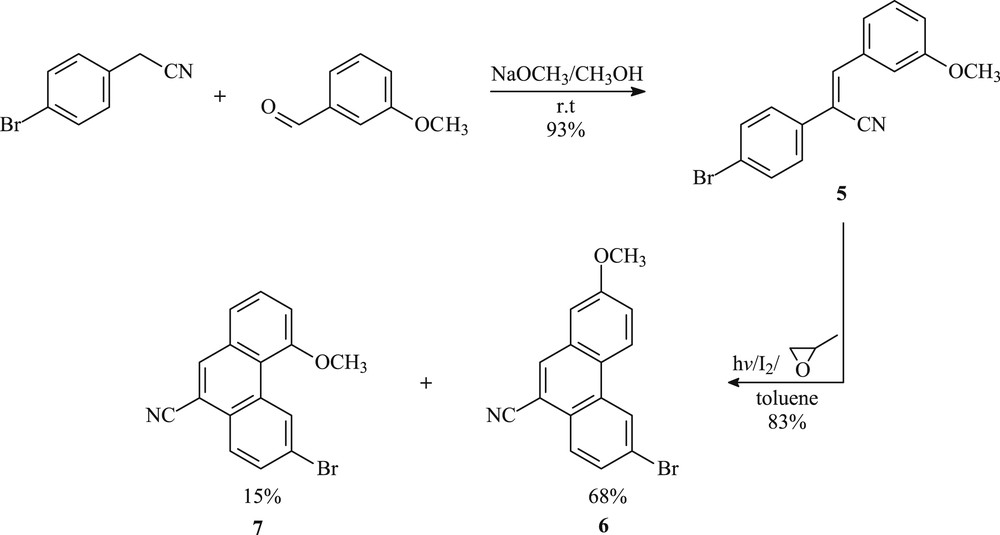
Synthetic pathway for the synthesis of phenanthrenes 6 and 7.
To obtain the helically chiral pentahelicene 9, we envisaged the coupling of the phenanthrene moiety 6 with 4-bromostyrene (1.5 equiv) using 0.5 mol % of Hermann's palladacycle {trans-di(μ-acetato)-bis[o-(di-o-tolylphosphino)benzyl]dipalladium} as the catalyst, sodium acetate as a base, and N,N-dimethylacetamide as a solvent. The desired coupled product 8, possessing (E)-stereochemistry at the double bond, is obtained in 81% yield after 48 h heating at 140 °C according to Scheme 2. To complete the synthesis of the target helicene, precursor 8 was exposed to photocyclization in the presence of a stoichiometric amount of iodine and excess of propylene oxide. Thus, photolysis of 8 was performed in 1.2 L of toluene, on a 150 mg scale per run for about 3 h to afford the expected 13-bromo-3-methoxypentahelicene-6-carbonitrile 9, as a yellow solid in 60% yield and 31% overall yield after purification by column chromatography (Scheme 2).
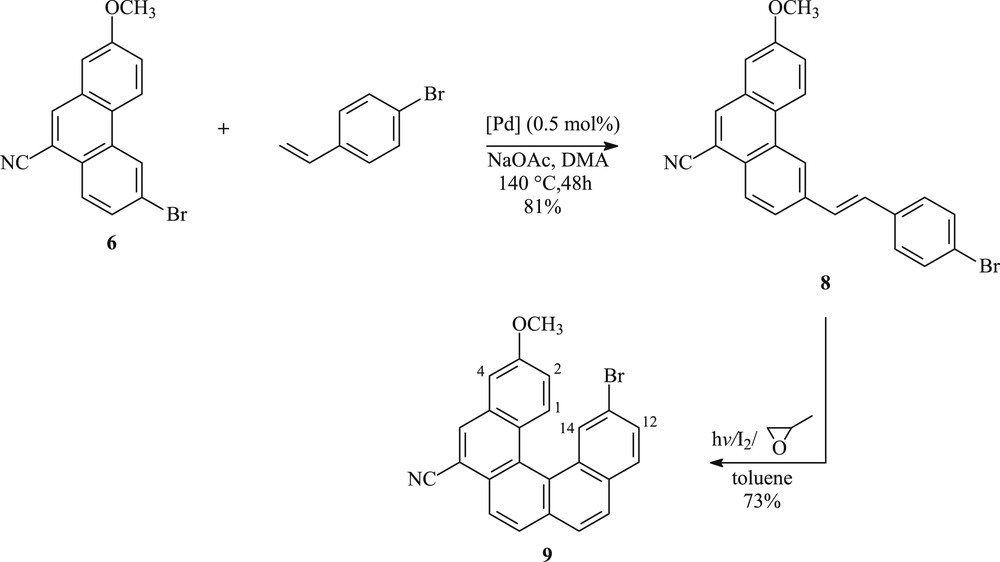
Synthetic route leading to the helically chiral pentacyclic system 9.
After the photocyclization of precursor 8, only the desired 13-bromo-3-methoxypentahelicene-6-carbonitrile (9) was observed as a cyclization product and no other regioisomer, such as 10 (Fig. 2), was isolated from the reaction mixture. The latter would be expected to show characteristic signals for both protons H-7 and H-14 at low field in the 1H NMR spectrum [13].
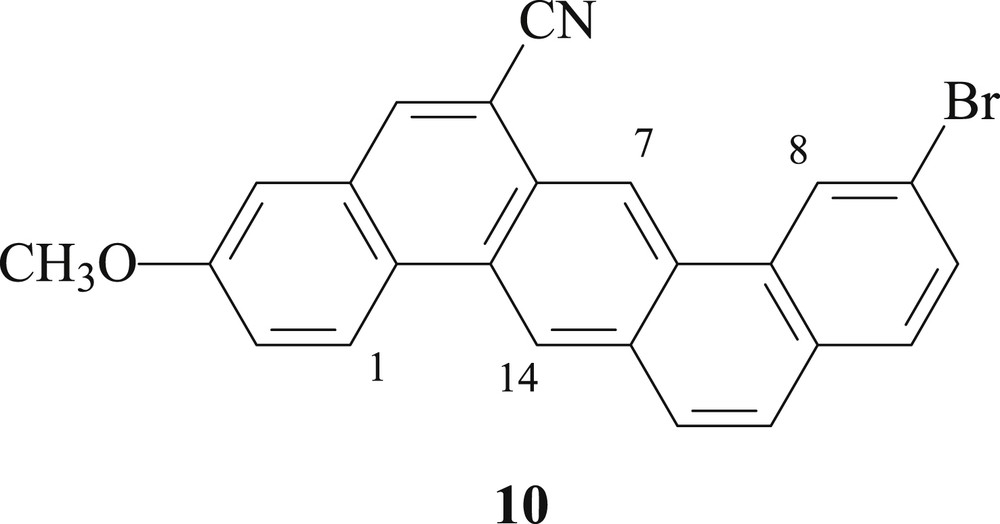
Chemical structure of regioisomer 10.
The obtained helicene was fully characterized by NMR spectroscopy and was found to show good solubility in common organic solvents such as dichloromethane, chloroform, toluene, and ethyl acetate.
The optical properties of the cyanopentahelicene derivative 9 were investigated using UV–vis absorption and photoluminescence (PL) spectroscopies at room temperature and the results are summarized in Table 1. The UV–vis absorption spectrum (Fig. 3), recorded from a dilute solution in dichloromethane (1.7 × 10−6), exhibited a strong absorption in the region of 300–440 nm. Absorption in the high-energy region is well-structured, containing prominent bands including 328, 344, 370, 382, 389, 405. These absorption bands are associated with π–π* and n–π* electronic transitions of the helicene unit. A broad absorption band in the low energy region, which is centered on 438 nm, is also observed. This band exhibits lower extinction coefficient as compared to the absorption bands in the higher energy area. To our knowledge, the absorption in this region does not appear in the case of other pentahelicene derivatives [14,15], but it characterizes the dicyano-substituted ones [10]. This absorption is attributed to the intramolecular charge transfer given that 9 contains a strong electron-withdrawing cyano group [16]. Moreover, the optical energy band gap Eg-op calculated from the absorption band edge of the UV–vis absorption spectrum was approximately 2.83 eV.
Photophysical properties of helicene 9.
Compound | Absorption | PL | |||
a (nm) | λonset (nm) | Eg-opb (eV) | λemsc (nm) | FWHMd (nm) | |
Helicene 9 | 311 | 438 | 2.83 | 539 | 111 |
a Absorption maxima measured in a dichloromethane solution (1.7 × 10−6 mol L−1) at room temperature.
b The optical gap (Eg-op) was estimated from the onset point of the absorption spectra: Eg-op = 1240/λonset.
c Emission maxima measured in a dichloromethane solution (6 × 10−5 mol L−1) at room temperature.
d Spectrum full width at half maximum.
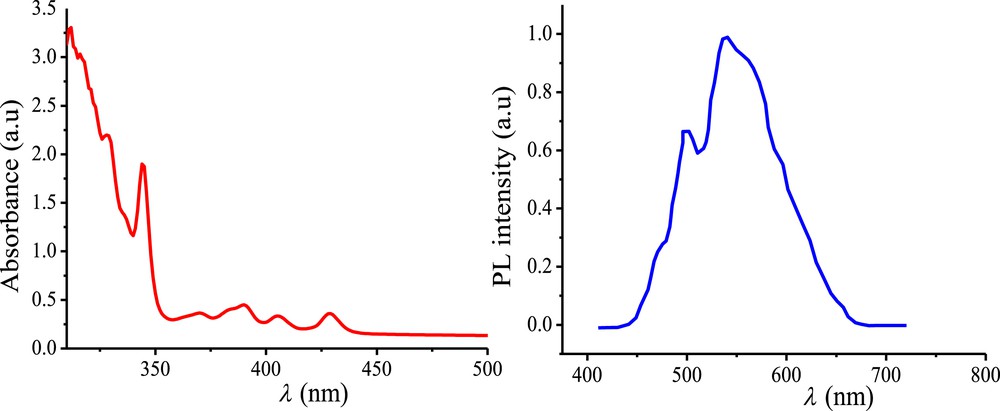
UV–vis absorption spectrum (red line) and PL spectrum (blue line) of compound 9 in dilute dichloromethane solutions.
The helical compound has a typical emission of organic π-conjugated molecules. PL of a dilute solution (6 × 10−5 M) of 9 in dichloromethane, at room temperature, exhibits two main emissions in the green region at 498 and 538 nm with a shoulder peak at 470 nm (Fig. 3).
The electrochemical properties of helicene 9 were investigated using cyclic voltammetry (Fig. 4) and the results are gathered in Table 2. The cyclic voltammetry (CV) has been performed at room temperature in anhydrous dichloromethane solutions containing 0.1 M of tetrabutylammonium perchlorate (n-Bu4NClO4). As shown in the cyclic voltammogram (Fig. 4), compound 9 exhibits an irreversible cathodic peak. The onset of oxidation (Vonset-ox) was found to occur at 1.55 V and the onset of reduction (Vonset-red) was at −1.15 V [vs Saturated calomel electrode (SCE)]. According to an empirical method [17] and by assuming that the energy level of the ferrocene/ferrocenium couple was 4.8 eV below the vacuum level, the highest occupied molecular orbital (HOMO) and lowest unoccupied molecular orbital (LUMO) energy levels were calculated as follows:
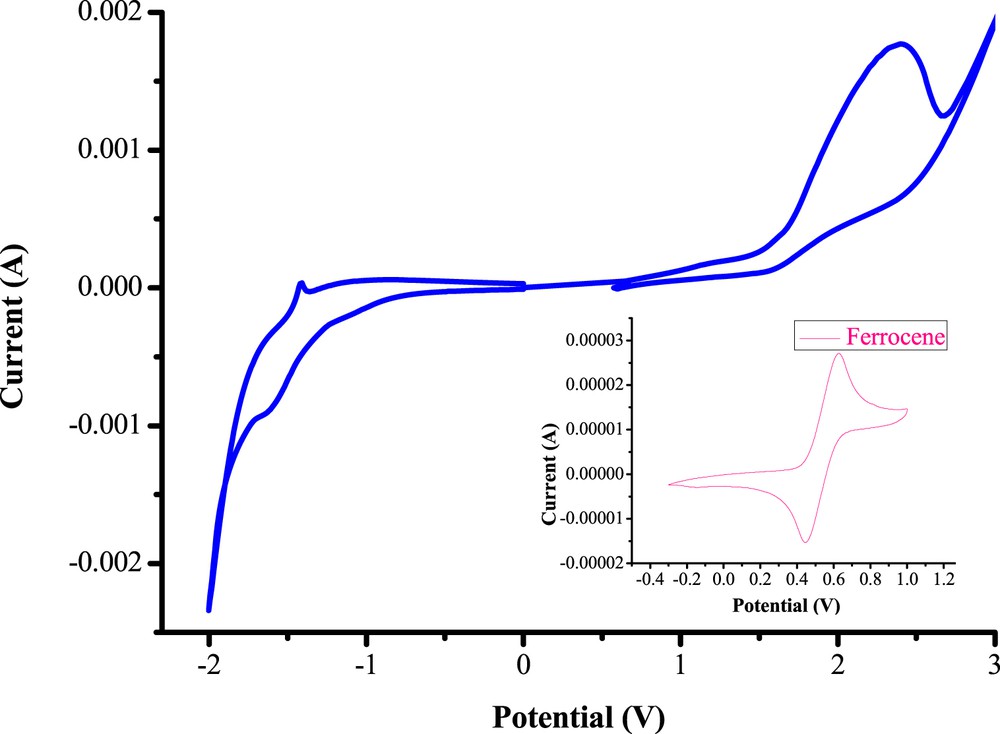
Cyclic voltammogram of pentahelicene 9 in 0.1 M solution of n-Bu4NClO4 in dichloromethane at a scanning rate of 50 mV s−1.
Electrochemical properties of helicene 9.
Compound | Vonset-ox (V) | Vonset-red (V) | EHOMOa (eV) | ELUMOb (eV) | Eg-elc (eV) |
Helicene 9 | 1.55 | −1.15 | −5.77 | −3.07 | 2.70 |
a Calculated from the oxidation potential.
b Calculated from the reduction potential.
c Band gap was calculated from the difference between HOMO and LUMO energy levels.
3 Conclusions
In summary, we have developed a facile and moderately functional-group-tolerant method for the synthesis of a new air-stable pentahelicene derivative. We accomplished the preparation of the target helicene in only four steps with an overall 31% yield. This helicene could be used as a building block for large [n]helicenes, for supramolecular architectures and as a convenient material for optoelectronic applications.
4 Experimental section
4.1 General
All reactions that were carried out under anhydrous conditions were performed under an inert nitrogen atmosphere. All reagents and solvents used in this study were purchased from Sigma–Aldrich unless otherwise noted. Isolated yields reflect the mass obtained after flash column silica gel chromatography. Organic compounds were purified using silica gel obtained from Silicycle Chemical division (40–63 nm; 230–240 mesh). The reactions were monitored by thin-layer chromatography (TLC) using commercial silica-gel plate 60 coated with a fluorescence indicator (Silicycle Chemical division, 0.25 mm, F254.). Visualization of TLC plate was performed by UV light at 254 nm. All mixed solvent eluents are reported as v/v solutions. Melting points were measured on a Bibby Scientific Stuart Digital, Advanced, SMP30. All reported compounds were homogeneous by TLC and 1H NMR. NMR spectra were taken in CDCl3 as a solvent with tetramethylsilane as the internal reference using Bruker AC-300 instruments unless otherwise noted. Signals due to the solvent served as the internal standard (CHCl3: δ 7.26 for 1H, δ 77.16 for 13C). The acquisition parameters are shown on all spectra. The 1H NMR chemical shifts and coupling constants were determined assuming the first-order behavior. Multiplicity is indicated by one or more of the following: s (singlet), d (doublet), t (triplet), q (quartet), m (multiplet), br (broad); the list of coupling constants (J) corresponds to the order of the multiplicity assignment. UV–vis spectra were recorded using a spectrophotometer UV-1600PC. Cyclic voltammetric experiments were performed with a PGSTAT30 Autolabpotentiostat and a μ-Autolab Type III connected to a conventional three-electrode cell, consisting in a silver-wire quasi-reference electrode, a platinum-wire counter electrode, and a platinum working electrode. Potential calibration of the silver wire was performed using a standard ferrocenium/ferrocene (Fc/Fc+) redox couple after the measurements. Investigations have been carried out in millimolar anhydrous dichloromethane solutions containing tetra-n-butylammonium perchlorate (0.1 M) as a supporting electrolyte. An ALS Co. Ltd. Ag+/Ag electrode ([Ag+] = 10−2 M in CH2Cl2) has been used as a reference. This reference electrode was calibrated with respect to the formal potential of the Fc+/Fc couple in CH2Cl2. Values for all potentials have been converted toward SCE using E versus SCE = E versus Ag/Ag+ (10−2) + 298 mV.
4.2 Experimental procedure for the Knoevenagel reaction
A solution of 4-bromophenylacetonitrile (3 g, 15.3 mmol) and 3-methoxybenzaldehyde (1.86 mL, 15.3 mmol) in dry methanol (20 mL) was placed in a two-necked flask fitted with a septum, then degassed and purged with argon and stirred at 0 °C for 5 min. Then, sodium methoxide (1.2 g, 25.9 mmol) was added in a small portion, and the mixture was stirred for 30 min at 0 °C and then 4 h at room temperature. The precipitate was collected using a sintered glass filter, washed with MeOH (5 mL) and then with water, and dried.
4.2.1 (Z)-2-(p-bromophenyl)-3-(m-methoxyphenyl)acrylonitrile (5)
White solid (4.47 g, 93%); mp = 47–49 °C; 1H NMR (300 MHz, CDCl3): δ (ppm): 3.90 (s, 3H, OCH3); 7.04 (s, 1H); 7.37–7.42 (m, 2H); 7.51–7,59 (m, 6H); 13C NMR (75 MHz, CDCl3): δ (ppm): 55.39 (OCH3); 110.88 (C); 113.65 (CH); 117.30 (CH); 117.50 (CN); 122.25 (CH); 123.46 (C); 127.51 (2CH); 129.95 (CH); 132.23 (2CH); 133.54 (C); 134.72 (C); 142.47 (CH); 160.01 (CO).
4.3 General procedure for the photocyclization reactions
Iodine (1.1 equiv) was added to a solution of the olefin in 1.2 L of toluene. The solution was degassed for 20–30 min and propylene oxide (50 equiv) was added. Irradiation was performed using a falling-film photoreactor and a high-pressure Hg vapor lamp (500 W, Hanovia). The argon flow was maintained throughout the irradiation, and the reaction was monitored by TLC. Then the reaction mixture was concentrated in vacuum and the crude product was purified by column chromatography on silica gel.
4.3.1 Irradiation of (Z)-2-(p-bromophenyl)-3-(m-methoxyphenyl)acrylonitrile (5) into phenanthrenes (6) and (7)
The photocyclization of 600 mg (1.9 mmol) of α,β-unsaturated nitrile 5 in toluene yielded 506 mg (83%) of a colorless solid, as a mixture of two regioisomers, after 2 h of irradiation (general procedure). Column chromatography on silica gel (eluent: cyclohexane/EtOAc 95:5) leads to 405 mg of pure 6-bromo-2-methoxyphenanthrene-9-carbonitrile (6) and 89 mg of 6-bromo-4-methoxyphenanthrene-9-carbonitrile (7).
4.3.1.1 6-Bromo-2-methoxyphenanthrene-9-carbonitrile (6)
White solid: 68%; mp = 158–160 °C; 1H NMR (300 MHz, CDCl3): δ (ppm): 4.00 (s, 3H, OCH3); 7.28 (s, 1H); 7.42 (dd, J1 = 2.1 Hz, J2 = 9 Hz, 1H, H3); 7.75 (d, J = 8.7 Hz, 1H); 8.09 (d, J = 8.7 Hz, 1H); 8,15 (s, 1H, H10); 8.46 (d, J = 9 Hz, 1H, H4); 8.70 (s, 1H, H5); 13C NMR (75 MHz, CDCl3): δ (ppm): 55.58 (OCH3); 109.28 (CH); 109.63 (C); 117.36 (CN); 120.75 (CH); 122.90 (C); 124.49 (CH); 124.92 (C); 125.43 (CH); 126.60 (C); 127.57 (CH); 130.23 (CH); 131.62 (C); 131.65 (C); 135.04 (CH); 159.48 (CO).
4.3.1.2 6-Bromo-4-methoxyphenanthrene-9-carbonitrile (7)
White solid: 15%; mp = 80–82 °C; 1H NMR (300 MHz, CDCl3): δ (ppm): 4.19 (s, 3H, OCH3); 7.31 (d, J = 7.8 Hz, 1H, H3); 7.35 (d, J = 7.5 Hz, 1H); 7.63–7.67 (m, 1H); 7.81 (d, J = 8.7 Hz, 1H); 8.14–8.19 (m, 2H); 9.87 (s, 1H, H5); 13C NMR (75 MHz, CDCl3): δ (ppm): 56.40 (OCH3); 109.89 (C); 111.60 (CH); 117.46 (CN); 121.05 (C); 122.31 (CH); 122.54 (C); 126.79 (CH); 127.81 (C); 128.28 (CH); 130.42 (CH); 131.35 (C); 131.63 (CH); 132.45 (C); 136.04 (CH); 158.54 (CO).
4.4 Experimental procedure for the Heck coupling
A solution of 6-bromo-2-methoxyphenanthrene-9-carbonitrile (6) (600 mg, 1.4 mmol) and NaOAc (131 mg, 1.6 mmol) in N,N-dimethylacetamide (4 mL) was placed in a Schlenk tube and degassed and purged with argon five times. 4-Bromostyrene (0.23 mL, 2 mmol) was added and the mixture was heated to 100 °C. Next, a solution of Hermann's catalyst (7 mg, 0.5 mol %) in N,N-dimethylacetamide (2 mL) was added and the mixture was heated to 140 °C for 48 h. After cooling, the solution was poured into water (15 mL) and the organic layer extracted with CHCl3 (3 × 30 mL), dried over anhydrous MgSO4, and the solvent evaporated. The crude product was purified using silica gel column chromatography with cyclohexane/EtOAc (90:10) as eluent to give 644 mg of olefin 8.
4.4.1 (E)-6-(p-bromostyryl)-2-methoxyphenanthrene-9-carbonitrile (8)
Pale yellow solid; 81%; mp = 200–202 °C; 1H NMR (300 MHz, CDCl3): δ (ppm): 3.98 (s, 3H, OCH3); 7.08–7.31 (m, 2H); 7.33–7.54 (m, 6H); 7.83–7.86 (d, J = 8.4 Hz, 1H); 8.10 (s, 1H); 8.19–8.22 (d, J = 8.4 Hz, 1H); 8.55–8.60 (m, 2H); 13C NMR (75 MHz, CDCl3): δ (ppm): 55.61 (OCH3); 109.02 (CH); 109.68 (C); 118.01 (CN); 120.85 (CH); 121.33 (CH); 121.87 (C); 124.44 (CH); 124.59 (CH); 126.04 (C); 126.46 (CH); 127.73 (C); 128.17 (2CH); 128.85 (CH); 129.40 (CH); 130.73 (C); 131.95 (C); 131.95 (2CH); 134.72 (CH); 136.05 (C); 136.82 (C); 159.09 (CO).
4.5 Irradiation of (E)-6-(p-bromostyryl)-2-methoxyphenanthrene-9-carbonitrile (8) into helicene (9)
The photocyclization reaction (general procedure) of 150 mg (0.36 mmol) of olefin 8 in toluene yielded 89 mg of 13-bromo-3-methoxypentahelicene-6-carbonitrile after purification by column chromatography with cyclohexane/AcOEt (95:05) as an eluent.
4.5.1 13-Bromo-3-methoxypentahelicene-6-carbonitrile (9)
Yellow solid: 73%; mp = 267–269 °C; 1H NMR (300 MHz, CDCl3): δ (ppm): 4.00 (s, 3H, OCH3); 7.07–7.11 (dd, J1 = 2.7 Hz, J2 = 9.3 Hz, 1H, H2); 7.28–7.29 (d, J = 2.7 Hz, 1H, H4); 7.58–7.62 (dd, J1 = 1.8 Hz, J2 = 8.4 Hz, 1H, H12); 7.79 (d, J = 8.7 Hz, 1H, H11); 7.84–7.92 (m, 3H, H8,9,10); 8.23 (d, J = 8.4 Hz, 1H); 8.29 (s, 1H, H5), 8.32 (d, J = 9.3 Hz, 1H, H1); 8.49–8.49 (d, J = 1.8 Hz, 1H, H14); 13C NMR (75 MHz, CDCl3): δ (ppm): 55.55 (OCH3); 107.50 (C4); 109.37 (C); 118.04 (CN); 118.95 (C2); 119.16 (C); 124.57 (CH); 125.28 (C); 126.51 (C); 126.64 (CH); 127.46 (C); 127.75 (CH); 128.04 (CH); 128.58 (C); 129.55 (C11); 129.79 (C12); 130.37 (C1); 130.67 (C14); 131.17 (C); 131.84 (C); 132.37 (C); 133.29 (C); 134.57 (C5); 158.80 (CO).
Acknowledgments
The authors are grateful to the “Direction générale de la recherche scientifique (DGRS)” of the Tunisian Ministry of Higher Education and Scientific Research for the financial support of this research. The authors also thank Dr. Mourad Chmekh (Unité de recherche: Matériaux et Dispositifs Electroniques Organiques, UR11ES55, Faculté des Sciences de Monastir-5000, Tunisia) for PL and cyclic voltammetry analysis.