1 Introduction
Precise determination of small amounts of hydrogen peroxide (H2O2) is a very important issue in many fields, such as chemistry, biology, environmental sciences, bioanalytics, and medicine [1]. Among many methods that have been already used for the detection and determination of H2O2 [2–5], electrochemical techniques seem to be the most promising strategies offering high accuracy, reliability, and ability to work in a real time, even in the complex biological systems [6]. Various enzyme-based electrochemical sensors with an excellent detection limit toward H2O2 have been already proposed in the literature [7]. However, significant drawbacks of these systems, such as low chemical stability (susceptibility to denaturation) and relatively low selectivity in the presence of other electroactive species, still hinder their wide practical applications. Therefore, a further development of nonenzymatic electrodes that can be used for the determination of H2O2 is strongly desirable [8,9]. Within this context, a great scientific attention has been focused on nanostructured electrodes that can offer reproducible results and much better stability [10,11]. For instance, we proposed a novel nanostructured electrode based on a dense array of silver nanowires (∼50 nm in diameter) that were electrochemically grown inside the pores of anodic aluminum oxide template. Such kind of an electrode not only showed a rapid and reproducible response toward hydrogen peroxide reduction but also excluded the interference from other substances commonly present in the biological samples [12,13]. Moreover, it is also well known that the significant improvement in the sensitivity of the electroanalytical method can be achieved by increasing the surface to volume ratio of the electrode material [14]. Very recently we reported that nanoporous Ag electrodes can be successfully obtained by simple electrodeposition of Ag–Zn followed by selective dealloying of Zn, and such kind of the sponge-like Ag electrode can be also used as an amperometric H2O2 sensor [15]. On the other hand, it was also reported that selective dealloying of one component can be a quite useful strategy for the fabrication of the nanoporous metallic nanowires [16,17].
Therefore, we decided to combine both approaches, and here we present for the first time a simple template-assisted fabrication of rough silver nanorod (Ag NR) arrays via electrochemical deposition of Ag–Zn alloy followed by Zn dealloying in 5 wt % H2SO4. As-obtained rough Ag NRs were investigated as amperometric sensors for the detection and determination of hydrogen peroxide. As far as we know, the codeposition of Ag–Zn inside porous templates has not been reported yet, especially in the context of fabrication of rough Ag NRs.
2 Materials and methods
The experimental procedure used for the fabrication of rough Ag NRs is schematically shown in Fig. 1. Polycarbonate (PC) membranes (Cyclopore, Whatman) were used as templates for the fabrication of Ag–Zn NRs (Fig. 1A). Before electrodeposition, a thin Ag layer (∼30 nm in thickness) was sputtered on one side of the membrane (Fig. 1B). A cyanide-based alkaline solution containing Ag+ and Zn2+ ions (1:3 molar ratio) was used as an electrolyte for electrodeposition of Ag–Zn alloy (for details see our recent work [15])—Fig. 1C. The process was carried out at the constant potential of −1.7 V for 5 min in a typical three-electrode system with Pt plates serving as both reference and counter electrodes. Just after electrodeposition, samples were rinsed with distilled water and ethanol, and PC membranes were dissolved in chloroform (Fig. 1D). As-obtained Ag–Zn NRs were subjected to selective dealloying of zinc in 5 wt % H2SO4 at room temperature for 6 h (Fig. 1E). The morphology and chemical composition of the NRs, before and after chemical etching, were characterized by using a field-emission scanning electron microscope and energy dispersive X-ray spectroscopy (FE-SEM/EDS, Hitachi S-4700 with a Noran System 7).

Schematic representation of the experimental procedure.
The electrochemical measurements were performed with a potentiostat/galvanostat (Reference 3000, Gamry) in a conventional three-electrode cell with the rough Ag NR array serving as the working electrode, a Pt counter electrode, and a saturated calomel electrode (SCE) as a reference. The amperometric response of the NR electrode to hydrogen peroxide was investigated at room temperature by the successive addition of 0.5 mM H2O2 to a continuously stirred 25 ml of 0.01 M phosphate buffer solution (pH 7.4) at the potential of −0.2 V versus SCE.
3 Results and discussion
The SEM images of Ag–Zn NRs after the dissolution of the PC template are shown in Fig. 2 A–C together with the corresponding EDS analysis (Fig. 2D).
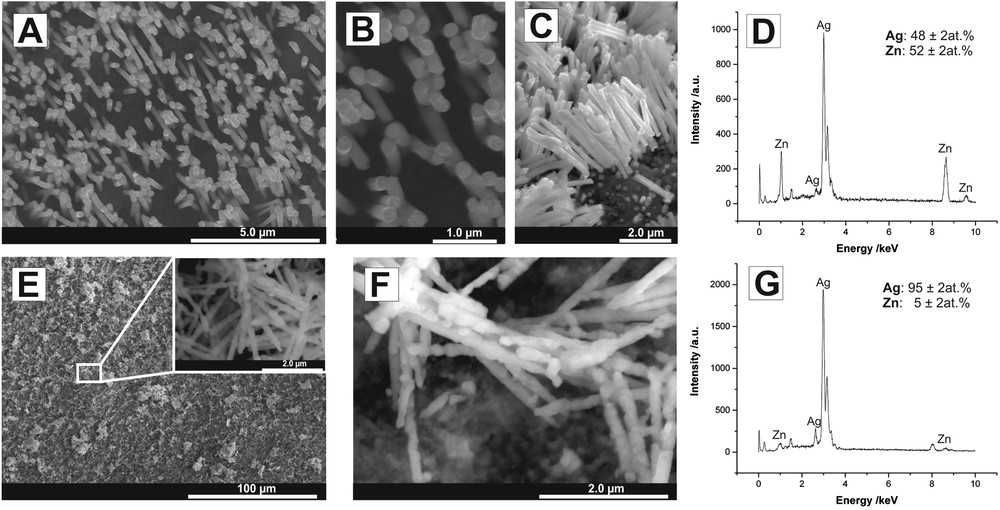
SEM images of Ag–Zn NR arrays after removal of the PC template before (A–C) and after (E, F) selective dealloying of Zn in 5 wt % H2SO4 together with the corresponding EDS spectra (D, G, respectively).
As can be seen, an array of vertically aligned NRs with the length between 2 and 3 μm was successfully obtained. The average NR diameter estimated from SEM images was about 250 nm. In addition, metallic NRs are continues, homogeneous in shape, and their walls are quite smooth and uniform. The EDS analysis (see Fig. 2D) confirmed the presence of both silver and zinc with an average atomic ratio of about 1:1 in the synthesized NRs. On the other hand, after 6 h of chemical etching in 5 wt % H2SO4, sidewalls of NRs became apparently rough (see Fig. 2F). This was accompanied by a significant decrease in Zn content to about 5 at.% (see Fig. 2G) as a result of selective dissolution of Zn from the Ag–Zn surface. In consequence, a slight decrease in the average NR diameter to about 200 nm was also noticed.
It should be mentioned that similar behavior of Ag–Zn thin films exposed to a sulfuric acid solution was reported in our previous article [15]. As can be seen, such selective dealloying of Ag–Zn NRs is also an effective method to further increase the surface area of NR-based electrodes. This effect can be very desirable, especially from the point of view of potential applications of such electrodes for electrochemical sensing. To confirm this effect, we examined the electrocatalytic activity of as-obtained NR electrodes toward reduction of hydrogen peroxide. Four different types of electrodes were examined: (sample 1) Ag–Zn NRs just after electrodeposition (NRs trapped inside the PC membrane); (sample 2) Ag–Zn NRs after removal of the PC template; (sample 3) Ag–Zn NRs inside the PC membrane after 6 h of chemical etching in 5 wt % H2SO4; and (sample 4) rough Ag NRs after dissolution of the PC membrane and dealloying of Zn. The amperometric response of all electrodes during the successive addition of 0.5 mM H2O2 aliquots at the potential of −0.2 V was recorded and the results are shown in Fig. 3A.
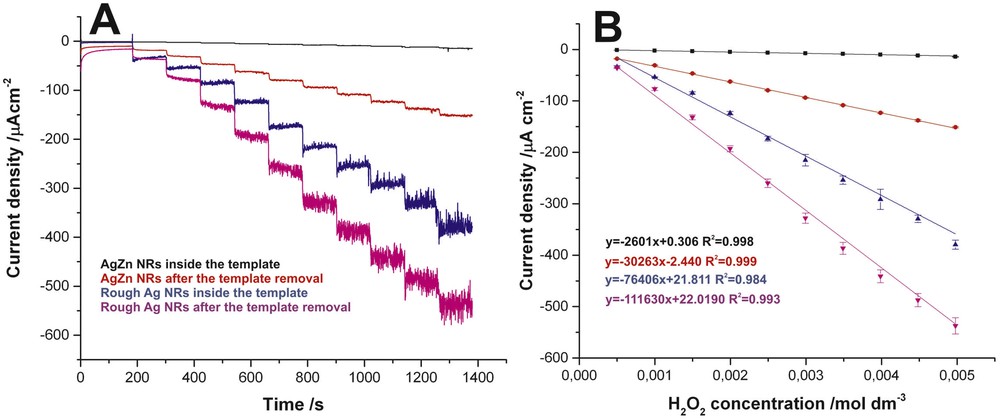
Amperometric response of AgZn electrodes before and after Zn dealloying upon successive additions of 0.5 mM H2O2 at the potential of −0.2 V versus SCE in a continuously stirred 0.01 M phosphate buffer solution with pH 7.4 (A). The corresponding calibration curve (B).
It is clearly visible that the addition of each portion of hydrogen peroxide results in a stepwise increase of cathodic current density, independently of the electrode used (current density was calculated with respect to a geometrical surface area of the active part of the electrode). However, it is evident that almost negligible current changes with increasing H2O2 concentration were observed for sample 1 (unetched Ag–Zn NRs inside the PC template). This can be attributed to the fact that a relatively low surface area of NRs was exposed to the electrolyte. As can be seen, the chemical dissolution of the PC membrane resulted in a significant improvement in the electrode response (see the red line in Fig. 3A) as a result of direct access of the electrolyte to the sidewalls of NRs. Surprisingly, much better electrochemical performance was observed for the NRs trapped in the PC membrane but subjected to the dealloying of Zn (see the blue line in Fig. 3A). It can be related to a decrease in the NR diameter, caused by chemical etching, that allows for a contact between the electrolyte and the NR sidewalls (even if NRs are still inside the PC template), and to the increased surface area of individual NRs. Finally, a further improvement in the electrode response can be achieved by the removal of the PC template (see the pink line in Fig. 3A) resulting in the formation of the electrode with the highest surface area exposed to the electrolyte. The corresponding calibration curves over the H2O2 concentration range 0.5–5.0 mM for all studied electrodes are presented in Fig. 3B. The sensitivity, limit of detection, and limit of quantification were also determined for all studied electrodes and the results are presented in Table 1. It is clearly visible that the best sensing performance was observed for the electrode based on the rough template-free Ag NRs (sample 4).
Sensitivity, limit of detection (LOD), and limit of quantification (LOQ) determined for all studied electrodes.
Sample number | Sensitivity (μA cm−2 mM−1) | LOD (mM) | LOQ (mM) |
1 | 2.6 ± 0.1 | 0.06 ± 0.01 | 0.21 ± 0.04 |
2 | 30.3 ± 0.3 | 0.06 ± 0.02 | 0.21 ± 0.06 |
3 | 76.4 ± 4.1 | 0.16 ± 0.04 | 0.54 ± 0.12 |
4 | 111.6 ± 3.1 | 0.08 ± 0.01 | 0.28 ± 0.05 |
However, it should be mentioned that as-formed nanostructured electrodes, especially after dealloying and template removal, are often relatively fragile. As can be seen in Fig. 2, some wires are ripped off from the supporting Ag film. This issue can significantly limit the long-term use of the electrode. Therefore, some additional research is still required to optimize the fabrication procedure to ensure the strong mechanical stability and integrity of the NR array. Among many strategies that can be used to improve the durability of such kind of rough nanostructured electrodes, the use of templates with crossing channels instead of parallel pores seems to be quite promising [18].
4 Conclusions
The following conclusions can be drawn from this study:
- 1. Ag–Zn NRs with an average diameter of ∼250 nm and the atomic ratio of about 1:1 can be easily obtained via simple electrochemical deposition inside the pores of commercially available PC membranes.
- 2. Selective etching of Zn from Ag–Zn NRs in 5 wt % H2SO4 results in the formation of NRs with a smaller diameter, rough sidewalls, and a much lower Zn content.
- 3. As-obtained rough Ag NR electrode exhibits quite promising electrocatalytic activity toward reduction of hydrogen peroxide and can be successfully used for amperometric detection of H2O2.
We believe that the proposed concept will be a promising strategy for the fabrication of nanostructured silver electrodes offering excellent electrochemical sensing properties.
Acknowledgments
This work was partially supported by the National Science Centre, Poland (grant decision no. DEC-2012/07/N/ST5/00155). The research was partially carried out with the equipment purchased. Thanks to the financial support of the European Regional Development Fund in the framework of the Polish Innovation Economy Operational Program (contract no. POIG.02.01.00-12-023/08). The SEM imaging was performed in the Laboratory of Field Emission Scanning Electron Microscopy and Microanalysis at the Institute of Geological Sciences, Jagiellonian University, Poland.