1 Introduction
Oxazol-5-ones (oxazolones) 1 and 4-arylmethyleneoxazolones (Erlenmeyer azlactones) 2 are versatile organic compounds with applications in different fields of research [1].
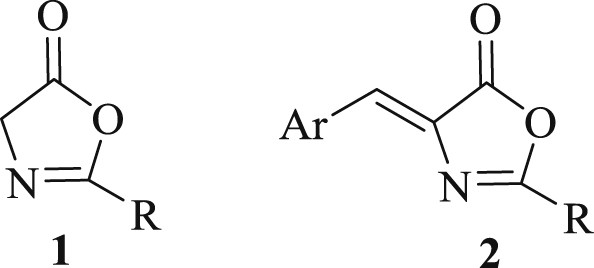
Oxazolone derivatives possess important biological activities [1,2]. Some prepared oxazolones showed inhibition of tyrosinase [3] and Human NK1 receptor antagonist [4]. Also, they are used as antioxidant and anticorrosive additives for lubricant oils [5]. In addition, oxazolones are used as versatile building blocks in synthetic organic chemistry for the synthesis of several heterocycles and biologically active molecules [6].
According to their importance, many efforts made for the synthesis of oxazolones and azlactones have been reported [7]. The general method for the preparation of oxazolones involves the classical cyclization of an N-acyl-α-amino acid with acetic anhydride under reflux conditions, where N-acyl-α-amino acids are prepared separately from acyl chlorides and glycine [8].
In a pioneering work, Erlenmeyer described the synthesis of azlactone by condensation of benzaldehyde with N-acetylglycine in the presence of acetic anhydride and sodium acetate [9]. To avoid the acidic and harsh conditions using acetic anhydride [10], other dehydrating agents such as carbodiimides [11], ethyl chloroformate [12], and arylsulfonyl chloride [13] have been reported.
Some drawbacks in these methods are multistep reactions from carboxylic acid to oxazolones, the need for low or high temperatures, lower yield of the products, harsh conditions, unnecessary waste, and chromatographic separations of products.
Chloromethylenedimethylammonium chloride (Vilsmeier reagent) 3 is easily prepared by the reaction of DMF and oxalyl chloride or thionyl chloride in dry dichloromethane [14]. This reagent has been identified as a formylating agent [15], a coupling reagent in the synthesis of esters [16], amides [17], acid chlorides [18], β-sultams [19], β-lactams [20], diacylhydrazines [21], 1,3,4-oxadiazoles [22], and 1,3,4-thiadiazoles [23].
To the best of our knowledge, the Vilsmeier reagent has not been used for the synthesis of oxazolones. Hence, in this article, we discussed the application of the Vilsmeier reagent for the conversion of carboxylic acid to oxazolones and Erlenmeyer azlactones.
2 Results and discussion
2.1 Synthesis
Hippuric acid 4a used as a starting material was simply prepared from benzoic acid and glycine in the presence of the Vilsmeier reagent. The prepared hippuric acid 4a was stirred with the Vilsmeier reagent in the presence of triethylamine in DMF to yield 2-phenyloxazol-5-one 5a in 66% yield. The progress of the reaction was monitored by thin-layer chromatography (TLC) until all hippuric acid 4a was consumed in the reaction. The reaction mixture was then quenched with water and ethyl acetate. The organic layer was washed with saturated sodium bicarbonate and dried over Na2SO4. After removal of the solvent and recrystallization from ethanol, 2-phenyloxazol-5-one 5a was obtained as pale yellow crystals.
To optimize the reaction conditions for the synthesis of 2-phenyloxazol-5-one 5a from hippuric acid 4a, various solvents and different molar amounts of the Vilsmeier reagent as well as temperature were investigated (Table 1). It was found that the yield of 5a in chloroform was higher than in other solvents. In addition, the best temperature for this reaction was room temperature giving highest yield of the desired product. As it is shown in Table 1, an equimolar amount of the Vilsmeier reagent was sufficient for completion of the reaction.
Vilsmeier reagent-mediated cyclodehydration of 4a.
Entry | Solvent | Temperature | Vilsmeier reagent (mmol) | Yield (%) |
1 | DMF | rt | 1.0 | 66 |
2 | CH2Cl2 | rt | 1.0 | 76 |
3 | Benzene | rt | 1.0 | 34 |
4 | CH3CN | rt | 1.0 | 58 |
5 | CHCl3 | rt | 1.0 | 81 |
6 | DMSO | rt | 1.0 | 61 |
7 | CHCl3 | rt | 0.8 | 55 |
8 | CHCl3 | rt | 1.3 | 76 |
8 | CHCl3 | 0 °C | 1.0 | –a |
9 | CHCl3 | 61 °C | 1.0 | 70 |
a Trace amount of the product was observed on TLC monitoring but it was not isolated.
Treatment of N-acyl-α-amino acids 4a–l with the Vilsmeier reagent and triethylamine in chloroform at room temperature gave oxazol-5-ones 5a–l with good to excellent yield after crystallization from ethanol (Scheme 1, Table 2). The structures of all compounds 5a–l were confirmed by elemental analysis and spectroscopic methods (IR, 1H NMR, and 13C NMR).
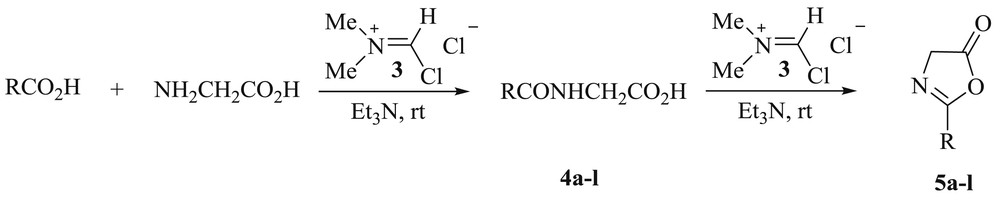
Synthesis of oxazol-5-ones 5a–l from N-acyl-α-amino acids 4a–l.
Synthesis of N-acyl-glycines 4a–l and oxazol-5-ones 5a–l using the Vilsmeier reagent.
Entry | R | RCONHCH2CO2H | Isolated yield (%) | Oxazol-5-one | Isolated yield (%) | Isolated yield (%) (one-pot synthesis) |
1 | Ph | 4a | 85 | 5a | 81 | 86 |
2 | 4-ClC6H4 | 4b | 83 | 5b | 88 | 89 |
3 | 4-MeOC6H4 | 4c | 75 | 5c | 78 | 84 |
4 | 4-NO2C6H4 | 4d | 90 | 5d | 86 | 91 |
5 | 4-MeC6H4 | 4e | 83 | 5e | 82 | 80 |
6 | C6H5CH2 | 4f | 79 | 5f | 72 | 78 |
7 | 4-(Me2N)C6H4 | 4g | 74 | 5g | 77 | 73 |
8 | 4-Pyridyl | 4h | 72 | 5h | 70 | 79 |
9 | 2-MeOC6H4 | 4i | 81 | 5i | 86 | 88 |
10 | 3-ClC6H4 | 4j | 84 | 5j | 85 | 90 |
11 | 2-Naphthyl | 4k | 89 | 5k | 87 | 92 |
12 | CH3CHCH | 4l | 74 | 5l | 79 | 76 |
Analysis of the IR spectrum of oxazol-5-ones 5a–l showed two characteristic signals at about 1650 and 1800 cm−1, which were ascribed to the presence of CN and CO bonds, respectively. Of course, the NH, CO amide, OH, and CO carboxylic acid peaks of N-acyl-α-amino acids 4a–l had vanished. Furthermore, the 1H NMR and 13C NMR spectra showed characteristic CH2, CN, and CO peaks.
Transformation of oxazol-5-ones and aldehydes to Erlenmeyer azlactones in chloroform has been previously reported [8d]. Then, oxazol-5-one 5a was converted to Erlenmeyer azlactone 6a in the presence of benzaldehyde and of the Vilsmeier reagent in 84% yield (Scheme 2).
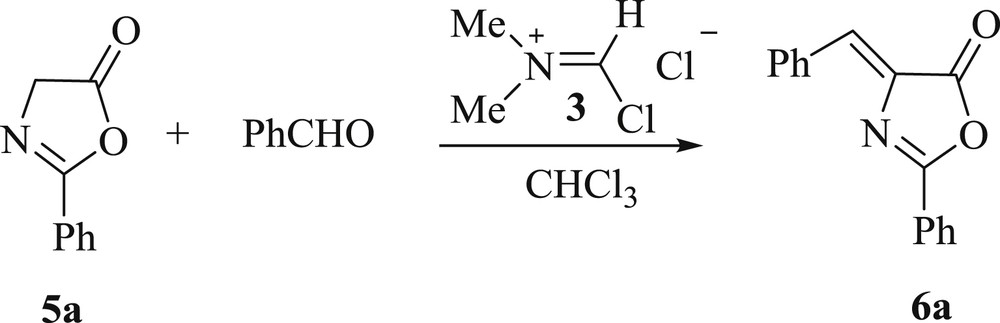
Transformation of oxazol-5-ones to Erlenmeyer azlactones.
These results prompted us to develop an easy method for the one-pot synthesis of oxazol-5-ones and azlactones from carboxylic acids. Reaction of benzoic acid (1.0 mmol) with glycine (1.0 mmol) in the presence of the Vilsmeier reagent 3, triethylamine, and benzaldehyde (1.0 mmol) in dry chloroform at room temperature for 3 h allowed direct conversion into the crude azlactone 6a. The mixture was washed with saturated NaHCO3 solution. Crystallization from 70% ethanol gave pure azlactone 5a as a yellow solid. In the absence of benzaldehyde, oxazol-5-one 5a was obtained. Then, using similar procedures, various pure oxazol-5-ones 5a–l and azlactones 6a–m were prepared directly from carboxylic acids (Scheme 3, Tables 2 and 3).

One-pot synthesis of oxazol-5-ones and azlactones from carboxylic acids.
One-pot synthesis of azlactones 6a–m from carboxylic acids using the Vilsmeier reagent.
Entry | R1 | R2 | Product | Isolated yield (%) |
1 | Ph | Ph | 6a | 92 |
2 | Ph | 4-ClC6H4 | 6b | 94 |
3 | Ph | 4-MeOC6H4 | 6c | 87 |
4 | Ph | CH3 | 6d | 69 |
5 | 4-MeOC6H4 | 2-Furyl | 6e | 82 |
6 | 4-ClC6H4 | 4-MeOC6H4 | 6f | 88 |
7 | 4-NO2C6H4 | 4-NO2C6H4 | 6g | 85 |
8 | C6H5CH2 | 4-MeC6H4 | 6h | 90 |
9 | 4-(Me2N)C6H4 | Ph | 6i | 87 |
10 | 4-(Me2N)C6H4 | 4-ClC6H4 | 6j | 94 |
11 | 4-Pyridyl | 4-MeOC6H4 | 6k | 66 |
12 | 2-Naphthyl | 4-MeC6H4 | 6l | 85 |
13 | CH3CHCH | Ph | 6m | 78 |
The synthesized compounds were characterized by IR, 1H NMR, 13C NMR, and elemental analysis.
The method was clean and convenient giving moderate to excellent isolated yields. In addition, the byproducts (DMF and salts) could be easily removed from the reaction mixture by aqueous work up.
3 Conclusion
To sum up, we have demonstrated the effectiveness and efficiency of the Vilsmeier reagent for the synthesis of oxazol-5-ones (oxazolones) and 4-arylmethyleneoxazolones (Erlenmeyer azlactones) proceeding via the cyclization of N-acyl-α-amino acids. Ready availability, high reactivity, and low cost make the Vilsmeier reagent a versatile and useful reagent for these reactions. In addition, we have developed facile and efficient methods for the synthesis of aliphatic, (hetero)-aromatic oxazol-5-ones, and 4-arylmethyleneoxazolones directly from carboxylic acids in a one-pot procedure. This methodology offers the advantage of mild reaction condition, good to excellent yield of products, and simplicity of work. Pure products were obtained by simple aqueous work up and crystallization.
4 Experimental section
4.1 Chemical reagents
All needed chemicals were purchased from Merck, Fluka, and Acros chemical companies. IR spectra were run on a Shimadzu FT-IR 8300 spectrophotometer. 1H NMR and 13C NMR spectra were recorded in DMSO-d6 and CDCl3 using a Bruker Avance DPX instrument (1H NMR 250 MHz, 13C NMR 62.9 MHz). Chemical shifts were reported in ppm (δ) downfield from tetramethylsilane. All of the coupling constants (J) are in Hertz. The mass spectra were recorded on a Shimadzu GC-MS QP 1000 EX instrument. Elemental analyses were run on a Thermo Finnigan Flash EA-1112 series. Melting points were determined in open capillaries with Buchi 510 melting point apparatus. TLC was carried out on silica gel 254 analytical sheets obtained from Fluka.
4.2 General procedure for the conversion of N-acyl-α-amino acids 4a–l to oxazol-5-ones 5a–l
A solution of N-acyl-α-amino acids 4a–l (1.0 mmol), Vilsmeier's reagent 3 (1.0 mmol), and Et3N (2.0 mmol) in dry chloroform (15 mL) was stirred at room temperature for 1.5 h. Water (15 mL) was added and the organic layer was separated. The organic layer was washed with brine (20 mL), dried (Na2SO4), filtered, and the solvent was removed under reduced pressure to give the crude products. The crude residues were purified by crystallization from 95% ethanol. Spectral data for 5a–f and 5i–j have been previously reported [24].
4.2.1 2-(4-(Dimethylamino)phenyl)oxazol-5(4H)-one (5g)
Yellow solid, mp 129–131 °C. IR (KBr) cm−1: 1802 (CO), 1631 (CN). 1H NMR (CDCl3) δ 3.17 (2 Me, s, 6H), 4.35 (CH2, s, 2H), 6.84 (ArH, d, 2H, J = 7.6), 7.47 (ArH, d, 2H, J = 7.6). 13C NMR (CDCl3) δ 44.8 (Me), 57.6 (CH2), 109.4, 125.2, 129.9, 154.6 (aromatic carbons), 164.5 (CN), 176.2 (CO). Anal. Calcd for C11H12N2O2: C, 64.69; H, 5.92; N, 13.72. Found: C, 64.81; H, 6.08; N, 13.66.
4.2.2 2-(Pyridin-4-yl)oxazol-5(4H)-one (5h)
Pale-yellow solid, mp 113–115 °C. IR (KBr) cm−1: 1811 (CO), 1634 (CN). 1H NMR (CDCl3) δ 4.18 (CH2, s, 2H), 7.33 (ArH, d, 2H, J = 7.9), 8.46 (ArH, d, 2H, J = 7.9). 13C NMR (CDCl3) δ 55.9 (CH2), 119.7, 134.1, 151.5 (aromatic carbons), 167.1 (CN), 178.4 (CO). Anal. Calcd for C8H6N2O2: C, 59.26; H, 3.73; N, 17.28. Found: C, 59.35; H, 3.84; N, 17.31.
4.2.3 2-(Naphthalen-2-yl)oxazol-5(4H)-one (5k)
Orange solid, mp 154–156 °C. IR (KBr) cm−1: 1805 (CO), 1639 (CN). 1H NMR (CDCl3) δ 4.53 (CH2, s, 2H), 7.26–7.29 (ArH, m, 2H), 7.60–7.65 (ArH, m, 3H), 7.72–7.75 (ArH, m, 2H). 13C NMR (CDCl3) δ 53.7 (CH2), 108.4, 114.7, 119.4, 121.2, 125.9, 127.5, 128.3, 131.7, 138.6, 144.9 (aromatic carbons), 166.2 (CN), 174.5 (CO). Anal. Calcd for C13H9NO2: C, 73.92; H, 4.30; N, 6.63. Found: C, 74.02; H, 4.44; N, 6.55.
4.2.4 2-(Prop-1-en-1-yl)oxazol-5(4H)-one (5l)
Colorless viscous oil. IR (KBr) cm−1: 1808 (CO), 1626 (CN). 1H NMR (CDCl3) δ 1.39 (Me, m, 3H), 5.26 (vinylic H, d, 1H, J = 15.7), 5.94 (vinylic H, m, 1H). 13C NMR (CDCl3) δ 20.6 (Me), 56.1 (CH2), 116.8, 138.5 (CC), 159.4 (CN), 177.1 (CO). Anal. Calcd for C6H7NO2: C, 57.59; H, 5.64; N, 11.19. Found: C, 57.51; H, 5.71; N, 11.16.
4.3 General procedure for one-pot synthesis of oxazol-5-ones 5a–l from carboxylic acids
Glycine (1.0 mmol) was added to a solution of carboxylic acid (1.0 mmol), Vilsmeier's reagent (2.0 mmol), and Et3N (4.0 mmol) in dry chloroform (15 mL) at room temperature. The mixture was stirred at room temperature 2 h. Saturated NaHCO3 (20 mL) was added and the organic layer was separated. It was washed with brine (20 mL), dried (Na2SO4), filtered, and the solvent was removed under reduced pressure to give the crude products. The crude residues were purified by crystallization from 95% ethanol.
4.4 General procedure for one-pot synthesis of azlactones 6a–m from carboxylic acids
The corresponding aldehyde (1.0 mmol) was added to a solution of the corresponding carboxylic acid (1.0 mmol), Vilsmeier's reagent (3.0 mmol), and Et3N (5.0 mmol) in dry chloroform (20 mL) at room temperature. After 2 h, saturated NaHCO3 (20 mL) was added and the organic layer was separated. It was washed with brine (20 mL), dried (Na2SO4), filtered, and the solvent was removed under reduced pressure to give the crude products. The crude residues were purified by crystallization from 70% ethanol. Spectral data for 6a–g, 6i, and 6m have been previously reported [8d,25].
4.4.1 2-Benzyl-4-(4-methoxybenzylidene)oxazol-5(4H)-one (6h)
Orange powder, mp 173–175 °C. IR (KBr) cm−1: 1631 (CN), 1793 (CO). 1H NMR (CDCl3) δ 3.11 (CH2, s, 2H), 3.57 (OMe, s, 3H), 7.06 (ArH, d, 2H, J = 7.9), 7.28–7.32 (ArH, m, 5H), 7.73 (ArH, s, 1H), 7.87 (ArH, d, 2H, J = 7.9). 13C NMR (CDCl3) δ 33.1 (CH2), 55.3 (OMe), 112.6, 114.9, 119.0, 122.4, 123.0, 124.7, 130.1, 138.3, 143.4, 154.8 (CC, aromatic carbons), 162.8 (CN), 166.4 (CO). Anal. Calcd for C18H15NO3: C, 73.71; H, 5.15; N, 4.78. Found: C, 73.81; H, 5.29; N, 4.83.
4.4.2 4-(4-Chlorobenzylidene)-2-(4-(dimethylamino)phenyl)-oxazol-5(4H)-one (6j)
Bright yellow solid, mp 186–188 °C. IR (KBr) cm−1: 1637 (CN), 1801 (CO). 1H NMR (CDCl3) δ 3.06 (Me, s, 3H), 6.91 (ArH, d, 2H, J = 8.1), 7.33–7.39 (ArH, m, 4H), 7.77–7.81 (ArH, m, 3H). 13C NMR (CDCl3) δ 42.5 (Me), 111.2, 115.7, 119.3, 124.4, 126.1, 127.5, 129.8, 133.6, 145.3, 151.7 (CC, aromatic carbons), 161.3 (CN), 165.8 (CO). Anal. Calcd for C18H15ClN2O2: C, 66.16; H, 4.63; N, 8.57. Found: C, 66.11; H, 4.71; N, 8.54.
4.4.3 4-(4-Methoxybenzylidene)-2-(pyridin-4-yl)oxazol-5(4H)-one (6k)
Orange solid, mp 174–176 °C. IR (KBr) cm−1: 1630 (CN), 1791 (CO). 1H NMR (CDCl3) δ 3.69 (OMe, s, 3H), 7.17 (ArH, d, 2H, J = 7.7), 7.49 (ArH, d, 2H, J = 7.7), 7.75–7.81 (ArH, m, 3H), 8.30 (ArH, d, 2H, J = 7.6). 13C NMR (CDCl3) δ 56.5 (OMe), 110.6, 114.1, 117.8, 122.6, 127.3, 132.4, 133.9, 147.5, 155.3 (CC, aromatic carbons), 162.6 (CN), 167.3 (CO). Anal. Calcd for C16H12N2O3: C, 68.56; H, 4.32; N, 9.99. Found: C, 68.64; H, 4.43; N, 10.05.
4.4.4 4-Benzylidene-2-(naphthalen-2-yl)oxazol-5(4H)-one (6l)
Orange solid, mp 195–197 °C. IR (KBr) cm−1: 1636 (CN), 1797 (CO). 1H NMR (CDCl3) δ 7.29–7.37 (ArH, m, 5H), 7.44–7.47 (ArH, m, 2H), 7.71–7.76 (ArH, m, 4H), 7.98–8.01 (ArH, m, 2H). 13C NMR (CDCl3) δ 107.4, 111.8, 120.6, 123.1, 125.8, 126.1, 126.5, 127.9, 128.5, 132.6, 132.9, 134.7, 138.4, 142.0, 146.2, 147.9 (CC, aromatic carbons), 164.3 (CN), 166.1 (CO). Anal. Calcd for C20H13NO2: C, 80.25; H, 4.38; N, 4.68. Found: C, 80.36; H, 4.53; N, 4.60.
Acknowledgments
Support for this work by the Marvdasht Branch of Islamic Azad University is gratefully acknowledged.