1 Introduction
Ultraviolet (UV) curable films find increasing applications in coating industries because of their rapid cure, solvent-free characteristics, application versatility, low energy requirements, and low-temperature operation [1]. Depending on the final application, it is desirable to formulate polymer film systems with low shrinkage, high flexibility, decreased viscosity, good adhesion, and low sedimentation, and syneresis risk for pigmented systems [2,3].
The UV-curing technique can be successfully used to perform the in situ intercalative polymerization obtaining crosslinked nanocomposites typically in the form of coatings. The clays can be dispersed in the liquid monomers and upon irradiation polymeric thermoset matrices can be built through a fast process, without the use of solvents and without heating the system [4]. Keller et al. have already reported the clay-nanocomposite formation accomplished by the UV curing of acrylic formulations [4–6]. The polymer–clay nanocomposites feature a remarkable dispersing effect, and they can effectively prevent pigment and filler from setting and agglomerating in long time storage [6].
Mostly nanoclays are “organically modified” clays that are produced by reacting organic cations, such as a quaternary ammonium chloride with refined bentonite. The reaction changes the nature of the clay from hydrophilic to hydrophobic. Clay modification allows one to make the phases compatible and avoid base ingredient sedimentation.
Clay–polymer nanocomposites offer low shrinkage and low sedimentation and syneresis risk for pigmented systems [2]. These properties make nanoclay-reinforced polymers attractive in a number of coating applications, as well as in medicines and cosmetics. As coatings in cosmetics for natural nail aesthetic and protective function are mostly pigmented, the main value for nanoclay addition could be decrease in sedimentation and syneresis risk of pigments [1,2].
The polymer–clay nanocomposites consist of clay nanolayers dispersed in a polymeric matrix. Two main types of structures can be obtained when the layered clays are present at a nanoscale level within the polymer: the intercalated structure, in which the polymer chains are intercalated between the silicate layers resulting in an ordered multilayer morphology built up with alternating polymeric and inorganic layers; and the exfoliated structure, in which the silicate layers are completely and uniformly dispersed as single platelets in a continuous polymer matrix [4].
The most widely used materials from the nanoclay group are the family of smectites with particular impact of montmorillonite and hectorite minerals. Smectites are the best known in industry as bentonites. When physically processed these minerals can provide strong thixotropic and shear thinning behavior to the systems where they are included. The main uses of nanoclays in paints and coatings are based in the supply of shear thinning (pseudoplastic) and thixotropic behavior to coating systems either in water, or in solvent and resins. These properties specifically improve the stability and application properties of these systems. This is transformed into clear benefits to settling and sag resistance, whereas it modifies viscosity and allows a good leveling and application [3].
It is observed that nanoclays maintain the strongest pseudoplastic behavior in comparison with associative thickeners and traditional cellulosic thickeners. Nanoclays generate high viscosities at low shear stress where gravity forces are operating and affecting properties as settling of fillers and pigments as well as sagging behavior and gel contraction processes. However, viscosity decays very fast as shear rate grows and therefore makes easy application and pumping operations of the paint. On the other hand, their contribution to paint consistency and viscosity in a solvent/resin–based coatings is very small. A flexible rheological system will combine special clays and organic thickeners with a less pseudoplastic behavior. Newtonian thickeners will be more recommended to control high shear rate processes as the application by roller without spattering, whereas clays will control sag and settling [3]. The second rheological characteristic, thixotropy, will be very important to get a good sag resistance while little affecting the leveling behavior because of the right recovery speed of the gel structure [3].
Clays are most widely used as rheology modifiers for paints and coatings [3,7], and only some research has been done on the effect of clay on UV-cured coatings [1,2,4–6]. Uhl et al. [7] studied organically modified montmorillonites in UV curable urethane acrylate films to improve thermal and mechanical properties. The 3% use of clay as a nanoreinforcement material led to enhancements in Young's modulus [8].
The present study describes the preparation of crosslinked polymers by photopolymerization of urethane acrylates containing organically modified clays. Comparing the different systems, the effect of the clay on the unreacted composite characteristics (viscosity and pigment suspension stability) and reacted film characteristics (modulus of elasticity, elongation at break, and film adhesion force) are discussed.
2 Materials and methods
2.1 Formula preparation
Base oligomers, urethane dimethacrylate, urethane diacrylate, tetrahydrofurfuryl acrylate, and photoinitiator (Table 1), were mixed (100–300 rpm) at room temperature in yellow light (589 nm) for 30 min. Cellulose acetate butyrate (CAB with molecular weight Mn=30,000 g/mol and butyryl content 52%) was dissolved in hydroxypropyl methacrylate and mixed (500 rpm) for 30 min. The clay composition was made from butyl acetate, water, and one of the clays mentioned in Table 1. The clay particle size diameter was 75 μm (75% of the particle size distribution). Clays were stirred (500 rpm) for 30 min and then all ingredients were mixed (300 rpm) together for 10 min. Uncured gels were stored at T = 40 °C for 24 h to remove trapped air. Afterward pigments was added and mixed (500 rpm) for 4 min.
Materials used to prepare clay–polymer composite systems.
Function | Chemical name | Trade name | Supplier | Concentration (wt % in formula) |
Base oligomer 1 | Urethane dimethacrylate | Exothane 8 | Esstech | 13 |
Base oligomer 2 | Urethane diacrylate | Ebecryl 1258 | Arkema | 28.5–34.5 |
Reactive monomer 1 | Hydroxypropyl methacrylate (HPMA) | HPMA | Esstech | 9 |
Reactive monomer 2 | Tetrahydrofurfuryl acrylate | SR285 | Sartomer | 19 |
Photoinitiator | Ethyl (2,4,6-trimethylbenzoyl) phenylphosphinate (TPO_L) | LucirinTPO_L | Basf | 2.5 |
Rheology modifier | CAB | CAB-551-0.2 | Eastman | 2 |
Solvent for clay implementation | Butyl acetate (BA) | BA | Brenntag | 15 |
Clay 1 | Stearalkonium bentonite | Tixogel VZV | BYK Additives | 1–3 |
Clay 2 | Stearalkonium bentonite | Tixogel LGM | BYK Additives | 1–3 |
Clay 3 | Stearalkonium hectorite. | Bentone 27V | Elementis Additives | 1–3 |
Pigment | TiO2 (CI 77891) | TiO2 | Durlin | 2 |
Pigment | Red 6 (CI 15850) | Red 6 | Durlin | 0.01 |
Water for clay activation | Water | – | 2 |
2.2 Coating formation
The coating was formed on the surface of acetone-treated Teflon sheets with a Byko drive from BYK Gardner applicator with 10 mm/s. The coating of 200 μm was cured under a UV lamp (KP800LED, luminous intensity 130 mW, λ = 405 nm) (YI Liang Electron Technology Co., China) for 30 s. After polymerization, the oxygen inhibition layer was removed with cotton pad soaked in 90% acetone.
2.3 Test methods
The effect of clay on the urethane acrylate mixture viscosity was determined using a Brookfield viscometer DV-II + Pro (Brookfield Engineering Laboratories, USA). The 64th needle was chosen. Measurements were taken in a flask with diameter 50 mm. Three parallel measurements for each composition were made at a speed of 60 rpm (for thixotropic observation experiment were used shear rates from 1 to 60 rpm). Measurement was read after 1 min. The viscosity of pigmented systems was determined after 24 h at thermostatically stable temperature (T = 20 °C).
To evaluate the effect of clay on the pigment sedimentation stability, tests on pigmented uncured mixtures were performed with 2% white pigment TiO2 and 0.01% red pigment RED 6 in a thermostatically controlled environment at the temperature of 40 °C for 4 weeks. After each 7-day period, the pigment sedimentation was evaluated optically. The test was considered to be passed (or the pigment system was regarded as stable) if after 4 weeks there was no difference in the color and homogeneity of the mixture and pigment sedimentation had not been observed.
The mechanical properties of UV-cured films were determined by standard tensile stress–strain tests to measure ultimate tensile strength (σ), modulus of elasticity (E), and elongation at break (ε). The formula used was E = σ/ε. Standard tensile stress–strain experiments were performed at room temperature on tensile tester BDO FB020TN (ZwickRoell, Germany) according to the requirements of LVS EN ISO 527-3:2000 [8] (sample shape, double blade films; the speed of the upper crosshead, 10 mm/min). Samples were placed in a universal testing facility holder and deformed at a rate of 25 mm/min. The results are the mean values of five independent measurements.
For pull-off adhesion tests, uncured systems were applied on glass panels of thickness 200 μm, cured under UV lamp KP800LED (λ = 405 nm) for 30 s, and surface cleaned with 90% acetone to remove the unreacted monomer layer.
The pull-off adhesion test method according to ISO 4624 was used. The coating was applied on glass panels (as it was the most even surface with highest test repeatability compared with plastic, metal, and natural keratin surfaces), cured, cleaned, and left for 12 h to polymerize. The coating was slightly ground with sandpaper and cleaned with 90% acetone solution. The same was done with the contact surface of a metal dolly (D = 20 mm). The contact surface of dolly was coated with superglue and put on the top of the polished coating, pressed against the glass, and retained for 30 s. Dollies were left on the coatings for 30 min. After 30 min a knife was used to cut a circle around the dolly. The pull tester was screwed on top of the dolly and twisted clockwise until it extracted the dolly from the surface (as in Fig. 1). The test result was read at the moment when the pull of tester broke away from the surface.
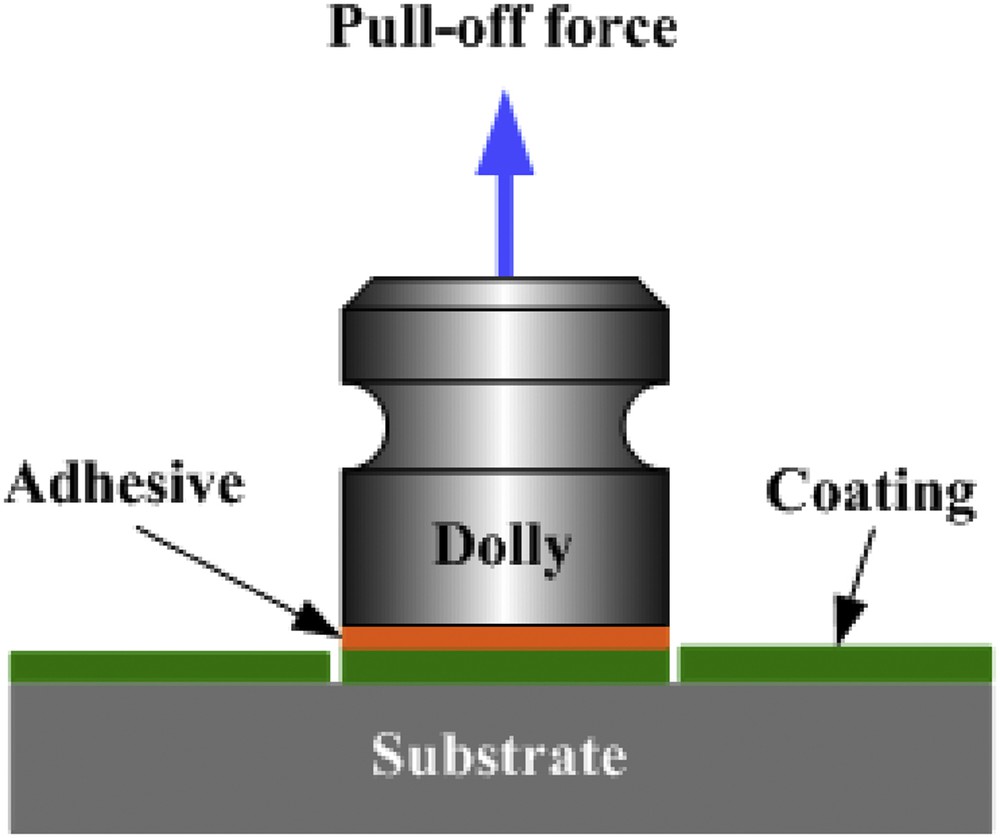
Pull-off adhesion tester.
3 Results and discussion
3.1 Pigmented system stability
All compositions were tested with white and red pigment dispersions, and after 4-week stability tests, (40 °C) the sedimentation and syneresis were observed for most of systems (Fig. 2). 1% and 1.5% addition of clays cannot eliminate pigment sedimentation and syneresis, but already 2% concentration of Tixogel clays is an optimal amount to reach stable pigmented compositions, tested for 4 weeks at 40 °C. Compositions with different clays (Tixogel VZV, Tixogel LGM, and Bentone 27V), which were stable (unchanged comparing with original sample) are highlighted in Fig. 3, where the effect of clay on systems' viscosity, which is one of acceptance criteria for nail coating applications, was evaluated. Industry request [1] is viscosity in the range from 3 to 4.4 Pa s.
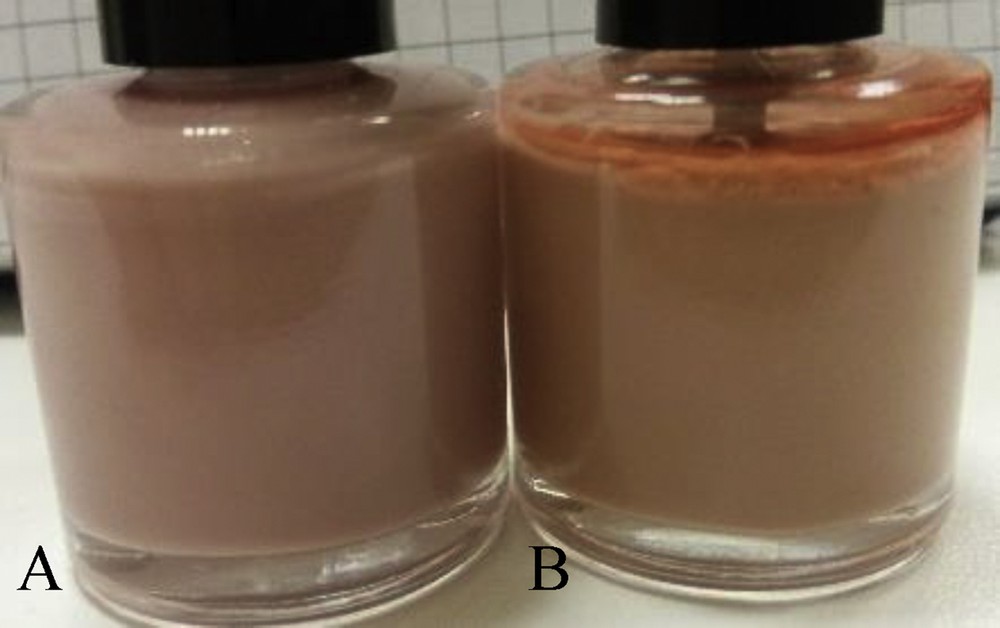
Pigmented systems before (A) and after (B) stability tests (4 weeks in 40 °C). Composition with 1.5% Tixogel.
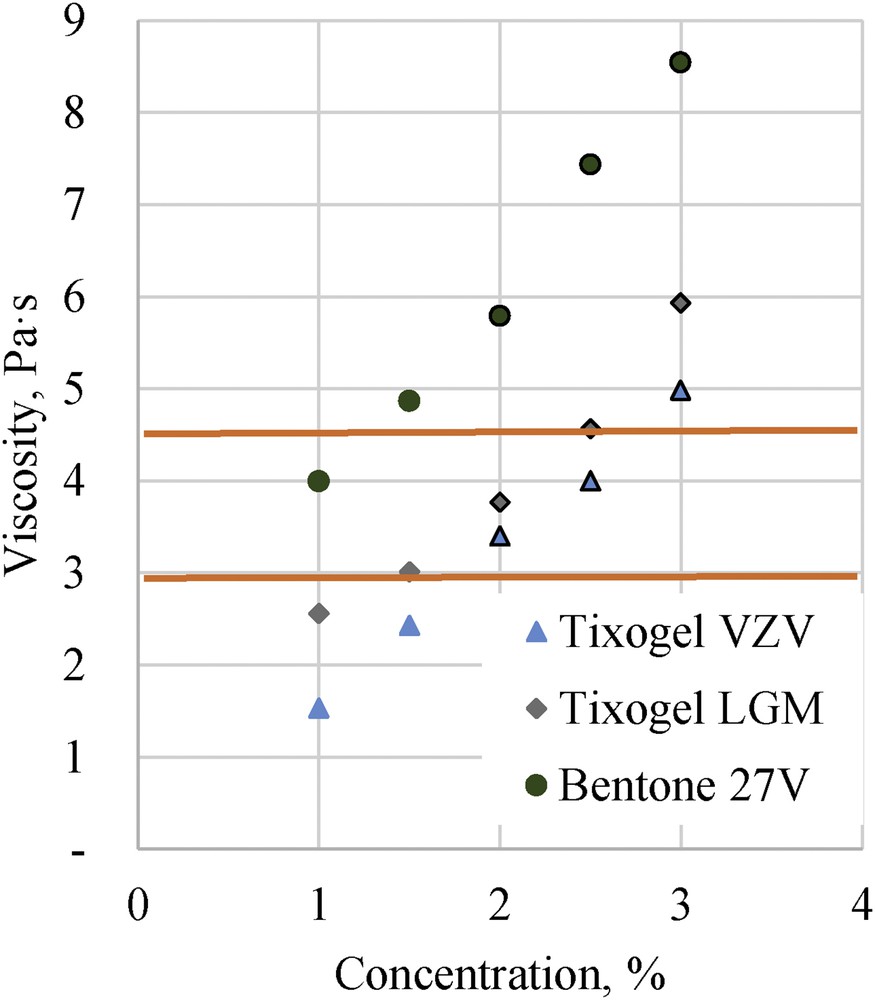
Composition viscosity dependence on clay concentration for three different clay additives with highlighted stable pigmented systems after 4-week stability tests. Lines indicate the permissible viscosity area.
3.2 System viscosity and stability
The required uncured composition steady-state viscosity for cosmetic applications as nail coatings should be between 3 and 4.4 Pa s. Addition of Bentone 27V drastically increased systems' viscosity, where system without clays had viscosity 0.74 Pa s, and with 2.5% of Bentone 27V the viscosity increased to 8.54 Pa s.
Only stable formulations in the required viscosity range were chosen for further testing. These were systems with 2% and 2.5% with Tixogel VZV and Tixogel LGM.
The systems were mixed (300 rpm) again for 5 min and their viscosities were determined in a steady state after measured period of time (1–10 min). Fig. 4 shows that after 7 min the system reached its steady-state viscosity. Also our previous research results with bentonite clays [1] confirm that in 10 min acrylate oligomer systems with clays recover its initial viscosity. This characteristic allows us to apply the coating on small surfaces without leakage and also helps to keep the pigments well dispersed in the system, decreasing agglomeration risk.
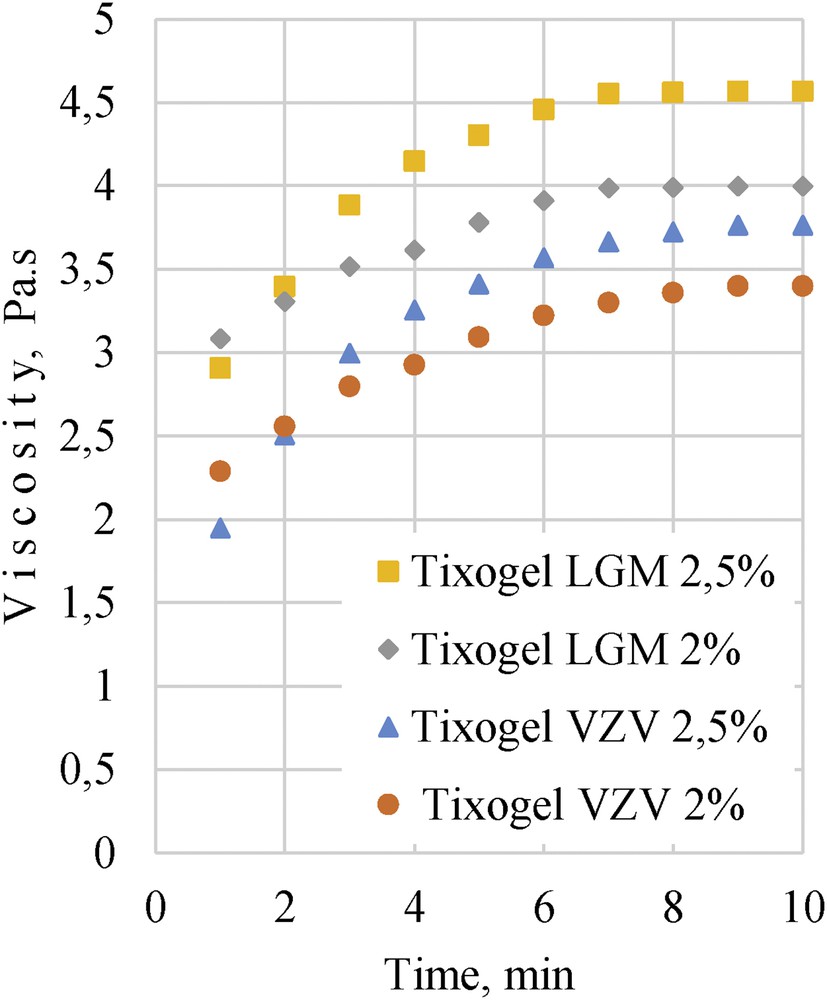
Composition viscosity dependence on steady-state time for different clay additives and their concentrations.
Measuring viscosity with different shear rates (from 1 to 60 rpm)—results indicate that all systems with clays had the thixotropic behavior (Fig. 5). Systems with higher concentration of clays showed more pronounced thixotropic behavior than systems with lesser concentrations [9–11].
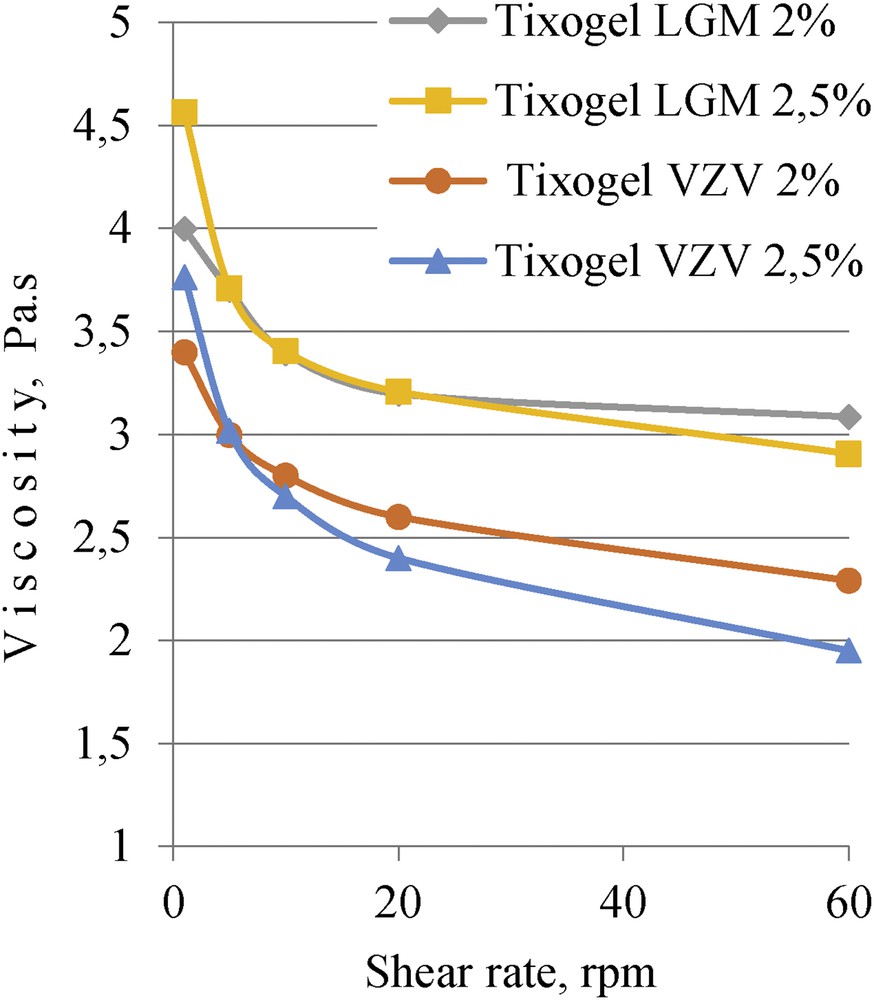
Composition viscosity dependence of shear rate for different clay additives and their concentrations.
Of the tested systems the most appropriate composition for application would be system with 2.5% Tixogel VZV, as it showed the most prominent thixotropic behavior. These systems have the highest thixotropic index, what can be seen as the difference between the viscosity measurements at low and high shear rates for this system: viscosity at 2.5 rpm is 3.72 Pa s and at 60 rpm is 1.94 Pa s.
In addition, clay producer indicates that Tixogel VZV is an organically modified bentonite designed for use in cosmetic systems to develop thixotropic rheological control and to impart a high degree of gelling efficiency, whereas Tixogel LGM is designed specifically for use in nail lacquers and intended to be thixotropic thickener for rheological control and highly efficient gellant and antisettling agent. Still the producer recommendations should be verified with each system individually, and after these experiments we can conclude that Tixogel VZV has the better compatibility with used oligomer/monomer system.
3.3 Physicomechanical characteristics
Young's modulus of natural nail at relative humidity 55% is 2.32 GPa. Deformation characteristics of the coating on the nail should be as close as possible to those of the natural nail to ensure good coating adhesion [8]. As natural nail can be deformed because of the humidity, and physical force-coating should move together with substrate.
Three of the tested compositions reached such a high modulus of elasticity as required for this type of application (400–900 MPa) [8]. Films containing 2% of clay additive Tixogel VZV reached the highest modulus values (697.62 MPa) and the highest elongation at break (82.32%) (Table 2). The addition of clays deteriorated the mechanical properties of the coating (E for coating without clays is 863 MPa and ε = 94%), but the impact of Tixogel VZV was smaller than the impact of Tixogel LGM.
Cured film adhesion force, modulus of elasticity, and elongation at break values for systems containing different clays.
Composition | Adhesion force (MPa) | Ultimate modulus, (MPa) | Elongation at break (%) |
Without clay additives | 3.0 | 863 | 94 |
Tixogel VZV 2% | 2.5 | 698 | 82 |
Tixogel VZV 2.5% | 2 | 684 | 60 |
Tixogel LGM 2% | 1 | 485 | 61 |
Tixogel LGM 2.5% | 0.5 | 264 | 41 |
Adhesion test results correlated with stress–strain test values (Table 2), showing that addition of clays decreased the mechanical characteristics of the coating, but still the best composition values were reached with 2% of Tixogel VZV, where adhesion force from glass substrate was 2.5 MPa.
3.4 Discussions
Researchers from Tolsa group studied the effect of clay on pigmented system rheology and stability and reported that to produce gel structures that are capable of behaving as shear thinning and thixotropic fluids, it is very important to find the right processing conditions [2]. We observed that, using Stearalkonium Hectorite (Bentone 27V), differentiation of processing conditions could lead to more successful results. This property is what makes them different in comparison with other rheological additives used in paints, and it is key to supply good antisettling and antisagging characteristics to paints and coatings either in water or in solvent systems [10–13]. In our study, we reached the effect of reinforcement, as systems with clay additives were able to keep pigment particles stable and not sedimented after 4 weeks at 40 °C; it also showed thixotropic effect of clay–polymer compositions confirms system homogeneity. Specifically Stearalkonium Hectorite has a great effect on the increase in system viscosity. Hectorites usually should be processed with the addition of polar activator [14] and as studied systems already contain water-based oligomers, this could be the reason for even better clay particle dispersion, which results in strongly increased viscosity and ability to keep pigment particles stable in the system without agglomeration and sedimentation.
The dispersions of bentonites (Tixogel VZV and Tixogel LGM) are therefore thixotropic: when undisturbed they increase in viscosity over time, and under a constant shear rate they decrease in viscosity over time. Bentonite dispersions are also pseudoplastic, because increasing the rate of applied shear (thereby increasing structure breakdown) results in decreasing viscosities. The yield point (resistance of the structure to breakdown) assumes that the solid disperse phase remains stable because it would need to exert a certain force (yield value) to move through the dispersion medium. Consequently, the greater the yield value, the more stable the suspension. On the other hand, thixotropy is an important feature for health care and cosmetic suspensions, because apparent viscosity decreases with increase in shear rate and increases again on standing, requiring a certain time for the structure to rebuild [12].
Because of the microsizes of clay particles, coating mechanical characteristics have been decreased. Probably better composition treatment with higher shear rates or under additional ultrasound sonification could decrease this negative effect.
4 Conclusions
The rheological and stability properties of uncured pigmented UV polymer films containing clays as a reinforcement material were studied. Both test results allowed finding the optimal concentration of clays to reach a stable pigmented system in the required viscosity range with thixotropic behavior. The use of clay as a reinforcement material led to small decreases in modulus of elasticity, elongation at break, and adhesion force.
Acknowledgments
The research was supported by Central Finance and Contracting Agency of the Republic of Latvia (CFLA) [project No 1.2.1.1/16/A/005].