1 Introduction
Nowadays, membranes have been widely used as physical barriers in the membrane gas–liquid separation process to separate the gas component using a liquid solvent. In this process, the pores of the membrane should be hindered from liquid intrusion to promote high diffusivity of gas molecules [1]. Conventionally, hydrophobic membranes were used, allowing only gas molecules to pass through the pores at an operational pressure lower than the liquid entry pressure. Nevertheless, it may be susceptible to pore wetting at an increased operational pressure, leading to a partially or completely wetted condition. This wetting phenomenon imposes significant resistance to the gas transport, which subsequently deteriorates the gas removal efficiency in liquid-occupied pores [2]. It is possible to mitigate the tendency of membrane wetting by increasing the surface hydrophobicity up to a superhydrophobic state. A superhydrophobic membrane with a water contact angle exceeding 150° offers an extreme water-repellency property. It allows for a higher diffusion flux and operational stability than a hydrophobic membrane in the gas–liquid separation process [3].
Many studies have emphasized on the enhancement of surface roughness and reduction in surface energy to endow superhydrophobicity on the membrane surface [4]. One of the methods to achieve these superhydrophobic properties is to incorporate hydrophobic nanoparticles in the polymer dope solution [5,6]. Most of the nanoparticles are intrinsically hydrophilic in nature. However, they can be functionalized by silane to reduce the water-reactive hydroxyl groups on the surface of nanoparticles to impart hydrophobicity. During the immersion precipitation process, the nanoparticles tend to embed in the polymer matrix, resulting in a hierarchical surface roughness and reduction in the surface energy.
The objective of this study was to develop a superhydrophobic membrane with enhanced water resistance. In this study, a superhydrophobic polyvinylidene fluoride-co-hexafluoropropylene (PVDF-HFP) membrane was synthesized by dispersing nano-SiO2 particles into the polymer dope solution via a nonsolvent-induced phase inversion process (NIPS). The SiO2 nanoparticles were grafted with silane, forming a long chain of nonpolar Si–O–Si chemical bonds to induce steric hindrance in the structure. The membrane properties including morphology, surface roughness, surface energy, and water contact angle were characterized.
2 Experimental section
2.1 Materials and membrane preparation
Commercial PVDF-HFP (Mw 4.0 × 105 g mol−1, Mw/Mn = 3.08) polymer pellets were purchased from Sigma-Aldrich, USA. Hydrophobic SiO2 nanoparticles supplied with courtesy from Wacker Chemie, Germany were used as solvent additives. The superhydrophobic membrane was fabricated by a NIPS process. PVDF-HFP polymer pellets and SiO2 nanoparticles were dried at 60 °C in a vacuum oven for 10 h to remove moisture. Specific amounts of hydrophobic SiO2 nanoparticles (e.g., 0, 1, 1.5, 2, 2.5, 3, 3.5 wt %) were added into 75 wt % (or 9.07 ml) of an NMP solvent and sonicated for 1 h. PVDF-HFP polymer pellets (15 wt % or 1.65 g) were dissolved in a mixture at 200 rpm and 70 °C for 24 h. The resulting solution was degassed for 5 h and left standing overnight. The dope solution was cast on a glass plate at 200 μm thickness gap and immersed in nonsolvent, ethanol, for 24 h. The membrane film was peeled from the glass plate and dried at room temperature for 48 h.
2.2 Membrane characterization
The surface microstructure of the membranes was observed using the Tabletop Microscope TM3030 (Hitachi, Japan). All samples were sputtered with a thin film of gold to render conductivity. The images of the membranes were taken at 15 kV accelerating voltage and 1000× magnification. Energy-dispersive X-ray spectroscopy (EDX) analysis was performed to quantify the weight percent of Si elemental composition on the membrane surface. The membrane hydrophobicity was examined via contact angle measurement using a goniometer (Rame-hart 260, USA). A 13-μL water droplet was dropped through a microsyringe onto the surface of the membrane under ambient conditions. The average value of each membrane was obtained from six measurements at different points. The geometric mean approach was used to determine the surface energy of the membrane [4]. In this method, contact angle of water and diiodomethane on the membrane surface was determined at room temperature. The surface energy was calculated from a set of two first-order linear equations. The surface roughness of the membrane was analyzed using a Phenom Desktop Scanning Electron Microscopy system with three-dimensional (3D) roughness reconstruction application. The images were reconstructed into 3D topography to determine the average surface roughness, Ra. The topographies of the membrane were measured at a scan area of 50 μm × 50 μm. For each membrane, three samples were analyzed, and the average was reported.
3 Results and discussion
3.1 Scanning electron microscopy
The membrane microstructural morphology was visualized by SEM. As illustrated in Fig. 1(a), crystallization-dominated structure composed of interconnected globules was observed in the pristine membrane without silica nanoparticles. Such morphology was induced by the polymer crystallization process in a soft precipitation bath (ethanol). During immersion precipitation process, the semicrystalline PVDF-HFP polymer induced the growth of crystallites in the membrane bulk [7]. The crystallizable segments of the polymer nucleated to similar globular size and merged together to form the interlinked spherulitic microparticles.
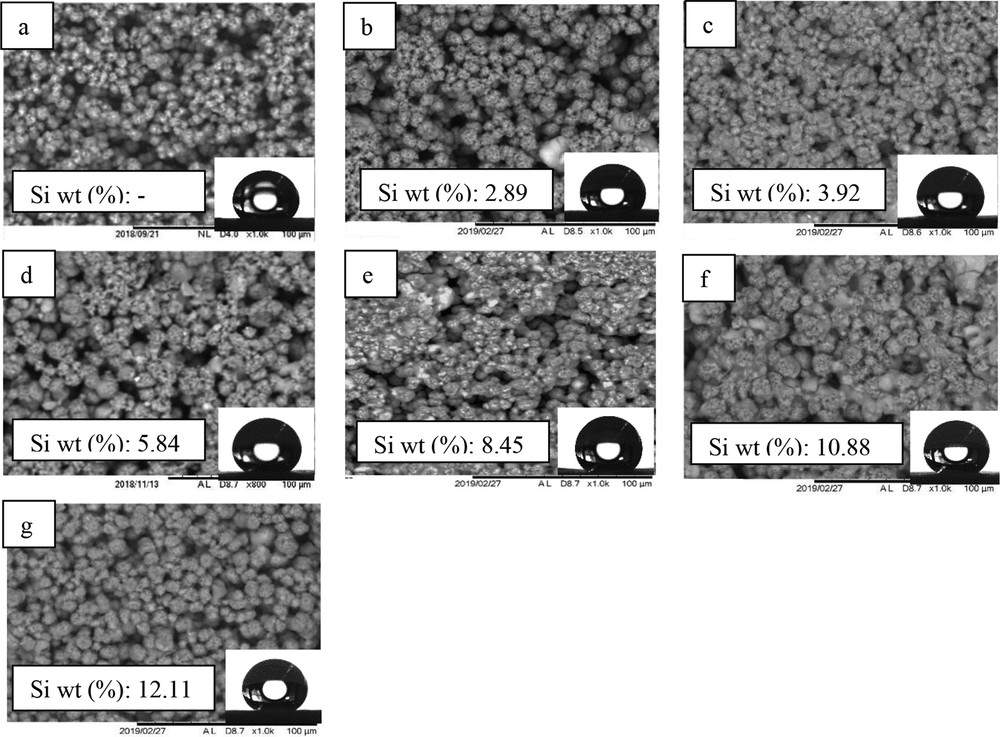
SEM micrographs and Si composition of membranes with SiO2 concentrations at (a) 0, (b) 1, (c) 1.5, (d) 2, (e) 2.5, (f) 3, and (g) 3.5 wt %. Insets show water droplet images of the respective membrane samples.
The surface morphology changes associated with the addition of nano-SiO2 particles are shown in Fig. 1. It was observed that the globular crystallites of the nano-SiO2 blended membranes appeared to be more closely packed with fewer micropores. According to Sukitpaneenit and Chung [8], the size of globules is dependent on the time allowed for crystallization, whereby longer time promoted the formation of bigger globules. The addition of hydrophobic SiO2 increased the viscosity of the polymer dope solution. This intensifies the delayed phase inversion and allows for a greater extent of polymer crystallization to occur in the coagulation bath. Subsequently, the top microstructure of the membrane becomes denser and more compact. The presence of nanoparticles on the membrane surface was examined by EDX analysis. As depicted in Fig. 1, the weight percentage of Si was increased from 2.89% to 12.11% with increased nanoparticles concentration from 1 to 3.5 wt %. This indicated the enhanced embedment of SiO2 nanoparticles in the polymer matrix.
Fig. 2 shows the 3D surface images and average surface roughness, Ra. For the SiO2-free pristine membrane, the Ra was lowest at 1.80 ± 0.37 μm due to low surface protrusion. Upon addition of nanoparticles, SiO2-blended membranes induced the formation of hierarchical structures with multilevel roughness on the membrane surface, which results in increased protrusions and valley regions. With the increase in nanoparticles' concentration up to 3.5 wt %, the Ra was improved by 49.7% to 3.58 ± 0.39 μm. The significant changes in the Ra may be attributed to the localization of PVDF-HFP spherulites and nanoparticles. A similar observation in surface roughness was reported by Ghaee et al. [6]. This indicates that the nanoparticles have a positive impact on membrane surface roughness.
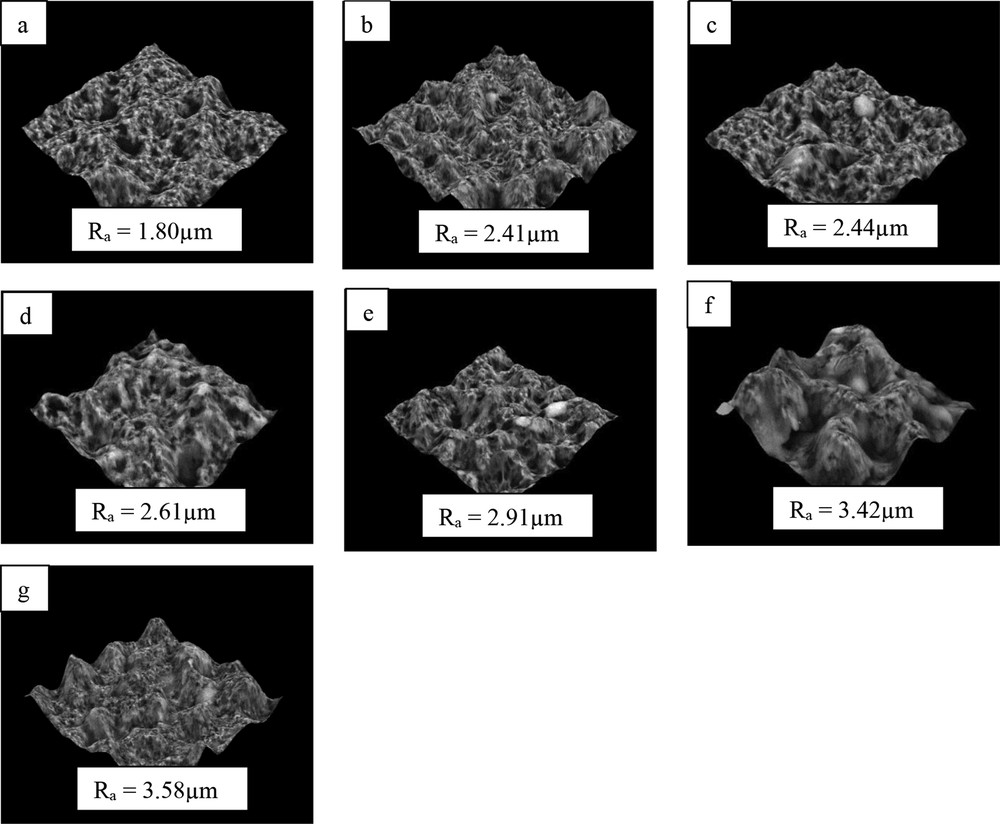
Three-dimensional images and average surface roughness of membranes with SiO2 concentrations at (a) 0, (b) 1, (c) 1.5, (d) 2, (e) 2.5, (f) 3, and (g) 3.5 wt %.
The surface energy of the membranes is shown in Fig. 3. The pristine membrane exhibited the highest surface energy of 9.56 mN m−1, indicating the presence of the highest dispersion force and polarity on the surface. Therefore, the pristine membrane is highly prone to interact with water molecules and cause wetting. By increasing the SiO2 content from 1 to 3.5 wt %, the surface energy was reduced from 9.29 to 3.49 mN m−1. This was consistent with the Si elemental composition results. The reduction in surface energy was attributed to the presence of a nonpolar Si–O–Si chain from hydrophobic SiO2 nanoparticles, which induced the steric hindrance effects in the structure. Therefore, nano-SiO2 blended membranes were hindered from interacting with polar molecules and thus led to enhanced antiwettability properties.
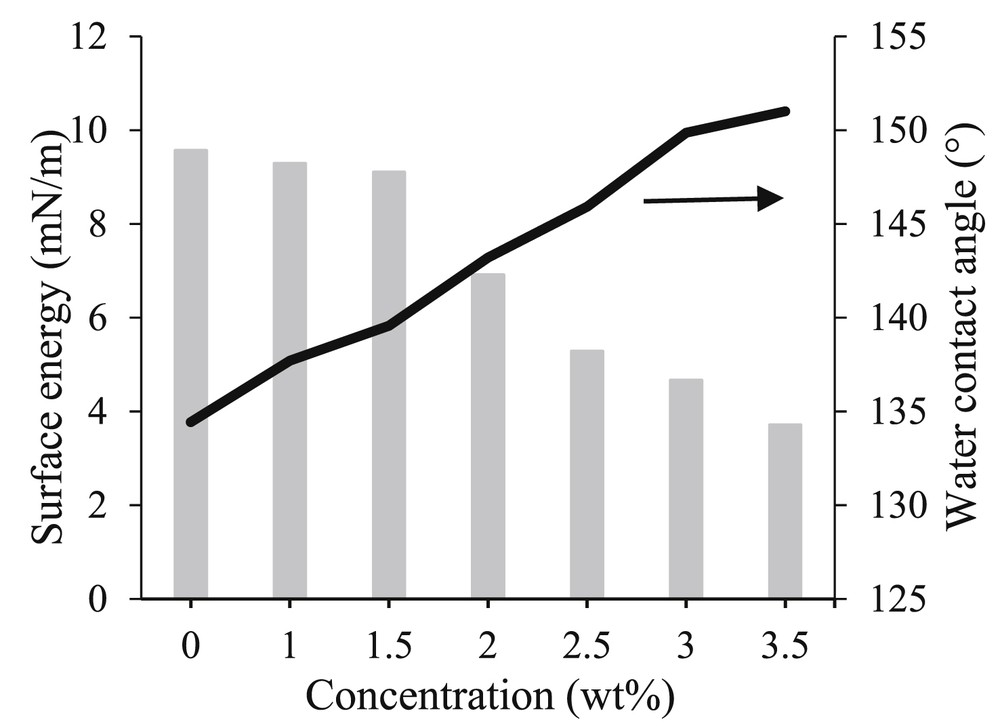
Water contact angle and surface energy of the synthesized membrane.
The wetting behavior of the resulting membrane is illustrated via water contact angle measurement in Fig. 3. The pristine membrane showed the lowest water contact angle of 135°. The development of a superhydrophobic surface was hindered by high surface energy and low surface roughness. After blending of nanoparticles, all the nano-SiO2 blended membranes revealed an increase in contact angle to the range of 138°–151°, which corresponds to a vast improvement in membrane hydrophobicity. The highest water contact angle of 151° was achieved in the membrane with 3.5 wt % of SiO2, demonstrating superhydrophobicity. As shown in Fig. 1(g), water droplets on this superhydrophobic surface are almost spherical in shape, due to the poor adsorption of water molecules onto the surface. According to the Wenzel model, such deviation in water contact angle was attributed to the significant increase in surface roughness and reduction in surface energy [9]. The textured surface trapped air pockets between the patchwork of hierarchical surface protrusions. Meanwhile, low surface energy decreased the adhesion of water molecules on the surface of the membrane. Therefore, water molecules have less direct contact with the membrane, rendering the surface superhydrophobic.
4 Conclusion
A superhydrophobic PVDF-HFP membrane was synthesized via a simple and facile NIPS. The results showed that the hydrophobicity of the nano-SiO2 blended membranes improved with increasing the concentration of hydrophobic SiO2. A superhydrophobic membrane with a water contact angle exceeding 150° was achieved at an SiO2 concentration of 3.5 wt %. Such excellent water repellency was attributed to reducing the membrane surface energy and enhancing its surface roughness. The synthesized superhydrophobic PVDF-HFP membrane of great potential can be used in the membrane gas–liquid separation application for improved water repellency.
Acknowledgments
This research work was supported by Universiti Teknologi PETRONAS under Fundamental Research Grant Scheme (FRGS) grant no. FRGS/1/2018/TK02/UTP/02/3 (Cost centre 015MA0-003) by the Ministry of Education, Malaysia.