1 Introduction
Dye-sensitized solar cells (DSSCs) otherwise known as Gratzel solar cells are third-generation solar cells. DSSCs are regarded as a potential alternative for first-generation Si wafer–based solar cells and second-generation thin film solar cells. Most of the material used for fabrication of DSSCs is inexpensive. The main advantages of DSSCs are low cost, ease of fabrication, relatively high efficiency, and its ability to work in low-light condition [1–3].
Synthetic Ru dyes such as N719 or black dye have high absorption spectrum and conversion efficiency but are not widely used owing to their high cost; one of the main advantages of DSSCs is their low–cost. Thus, natural dye-based DSSCs can be considered as the potential alternative for Ru-based commercial dyes because of their low cost. Natural dye pigments such as chlorophyll [1–3], anthocyanins [2,4], carotenes [5,6], betacyanins [7,8], and curcumin [9] can be used as a sensitizer source for DSSCs; do not require a complex extraction process and can be extracted from natural resources such as flower, plant, leaves, etc.; and do not require toxicity testing [10]. But one limiting parameter of natural dye-based DSSCs is that they yield low cell efficiency.
The cell performance of the natural dye-based DSSC depends on the absorption of photon energy from the sun. But the single individual dye-sensitized DSSC has low absorbance. To have an utmost response, a wide range of light absorption and higher absorbance over the entire visible region and near infrared (IR) spectrum can be achieved by using a combination of two (or more) dyes [11]. DSSCs sensitized with the combination of dyes show enhanced photovoltaic performance than the single individual dye-sensitized DSSCs [11]. Different chemical methods have been used to prepare a nanocrystalline TiO2 semiconductor layer on transparent conduction glass ITO (indium tin oxide)/FTO (fluorine doped tin oxide) [12–19], and treating with TiCl4 also shows improved cell efficiency compared with efficiency achieved without TiCl4 treatment [4,11]. Introducing TiCl4 into the cell (FTO/TiO2) forms a potential barrier at the transparent conducting oxide (TCO)/electrolyte interface and thus reduces charge carrier recombination, charge leakage, and charge trapping in the cell. Furthermore, the blocking layer (TiCl4-treated FTO/TiO2) increases absorption of the dye molecules in the cell [4,20–22].
In this present work, the effect of the combination of natural red dye extracted from red spinach (Amaranthus dubius) and yellow dye extracted from turmeric (Curcuma longa) on TiO2-based DSSC has been observed. In addition, the effect of post-TiCl4 treatment in improving cell efficiency has been studied for DSSC application. Furthermore, the effect of water content in the combination of dyes has been investigated.
2 Experimental section
2.1 Preparation of the anode
All chemicals were procured from Merck, Germany, and Dyesol, Australia, and used without any further purification. The anode was prepared as mentioned in an earlier publication [11]. A homogeneous mixture of 1.0 g of Degussa P25 (TiO2), 3 ml of 0.1 M citric acid (C6H8O7), 0.005 ml of Triton X-100(C8H17C6H4[OCH2CH2]), 0.01 ml of polyethylene glycol (HO[C2H4O]nH), and 0.01 ml of titanium IV isopropoxide (C12H28O4Ti) was prepared for TiO2 paste. An FTO glass substrate (surface resistivity = 8 Ω/sq. and transmittance >80%) was thoroughly cleaned to remove any organic or inorganic contaminants or adhesive on its surface. Prepared TiO2 paste was doctor bladed on the conducting side of the FTO glass substrate. Active cell area was 2 × 2 cm2. A single-layered TiO2 nanoporous film was air-dried and sintered at 450 °C in a muffle furnace for 60 min and left for 6 h to cool it down. Thickness of the single-layered TiO2 film was around 8–12 μm. Two different sets of anodes were prepared: (1) FTO/TiO2 and (2) TiCl4-treated FTO/TiO2. For the TiCl4-treated FTO/TiO2, the FTO/TiO2 was treated in 0.05 M TiCl4 solution by keeping the FTO/TiO2 in TiCl4 solution for 60 min at 70 °C [4]. Then, the TiCl4-treated FTO/TiO2 device was cleaned with deionized (DI) water, air-dried, and sintered at 450 °C in a muffle furnace for 60 min and left for 12 h to cool it down [4].
2.2 Preparation of the dye sensitizer
Natural red and yellow dyes were extracted from red spinach (A. dubius) and turmeric (C. longa), respectively. These plants were procured from the local market from Dhaka city, Bangladesh.
The red dye was obtained according to the following steps: First, fresh red spinach plants (except root) were cut into small pieces. Red spinach and distilled water in a ratio of 10 g: 1 ml were taken in a beaker and heated at 60 °C for about an hour. Then, the leaves and stems were filtered out. The (red) colored solution was collected and cooled in the absence of light (to avoid photodegradation of red dye). Anthocyanin was present in the solution with other pigments such as chlorophyll, less polar or non-polar flavonoids, and other compounds. To separate anthocyanin, a liquid-liquid separation technique was followed. In a separatory funnel, 50 ml of chloroform and 50 ml of dye solution were taken and mixed properly. After a few minutes (about 5 min), two different layers were formed in the funnel. In the bottom layer, chlorophyll, less polar or non-polar flavonoids, and other compounds were present and the solvent was chloroform water. This bottom part was filtered out. The top part of the solution, the aqueous solution of anthocyanin, was used as a sensitizer for DSSCs. The concentration of red dye was approximately 0.2767 g/10 ml in aqueous solution.
To prepare ethanol-extracted natural yellow dye, the skin of the turmeric was peeled off and washed carefully in DI water. Then, the turmeric was cut into small pieces and vacuum dried for 12 h to remove moisture. By using a mortar and pestle, dried turmeric was crushed into pieces. Powdered turmeric of 2 g/10 ml was added to a 99.9 wt % ethanol solution and kept without exposure to direct sunlight for 24 h. Finally, solid residues were filtrated out and used as the sensitizer for DSSCs. The concentration of yellow dye was 160 mg/10 ml ethanol solution.
Individual red and yellow dyes were used for single dye-sensitized DSSCs.
- • Red dye (R) = 20 ml of red dye (100% red dye)
- • Yellow dye (Y) = 20 ml of yellow dye (100% yellow dye)
Five different combinations of dyes (red + yellow) were prepared by combining these two dyes at different volume ratios as follows:
- • Combination 1 (C1) = 20 ml of red dye + 5 ml of yellow dye (80% red + 20% yellow)
- • Combination 2 (C2) = 15 ml of red dye + 10 ml of yellow dye (60% red + 40% yellow)
- • Combination 3 (C3) = 12.5 ml of red dye + 12.5 ml of yellow dye (50% red + 50% yellow)
- • Combination 4 (C4) = 10 ml of red dye + 15 ml of yellow dye (40% red + 60% yellow) and
- • Combination 5 (C5) = 5 ml of red dye + 20 ml of yellow dye (20% red + 80% yellow)
All these five combinations of dyes were used as the dye sensitizer source for DSSCs. To remove the possible effect of water (as DI water was the extracting solvent used for red dye), five new different combinations were prepared by keeping the volume of red dye fixed at 20 ml and changing the volume of yellow dye from 5 ml to 80 ml in the dye mixture as follows:
- • Combination 6 (C6) = 20 ml of red dye + 5 ml of yellow dye (80% red + 20% yellow)
- • Combination 7 (C7) = 20 ml of red dye + 13.3 ml of yellow dye (60% red + 40% yellow)
- • Combination 8 (C8) = 20 ml of red dye + 20 ml of yellow dye (50% red + 50% yellow)
- • Combination 9 (C9) = 20 ml of red dye + 30 ml of yellow dye (40% red + 60% yellow) and
- • Combination 10 (C10) = 20 ml of red dye + 80 ml of yellow dye (20% red + 80% yellow)
All these combinations of dyes were also used as the sensitizer source for DSSCs.
2.3 Electrolyte preparation
A homogenous mixture of 0.83 g of potassium iodide (KI), 0.127 g of iodine (I2), and 10 ml of ethylene glycol (C2H6O2) was used as I−/I3− redox electrolyte solution. All chemicals were of laboratory grade and purchased from Merck, Germany. This homogenous iodine electrolyte solution was kept in a dark container in the absence of any light [11].
2.4 Cathode preparation and cell assembly
The FTO glass was covered with carbon made by candle nip technique for the cathode. Both the FTO/TiO2 and TiCl4-treated FTO/TiO2 devices were soaked in the dye sensitizer for 60 min to fabricate a working photoanode [11]. The dye-absorbed anode (both FTO/TiO2 and TiCl4-treated FTO/TiO2) or photoanode was cleaned with distilled water to remove (if any) unbound natural dye pigments [3]. Then, the photoanode was dried in an oven at 40 °C for 60 min. The photoanode and cathode were assembled into a sandwich-type cell, and the liquid electrolyte was inserted into the cell.
3 Results and discussion
3.1 Scanning electron microscopy
Fig. 1(a) shows the scanning electron microscopy (SEM) image of the FTO/TiO2 anode and pores in the film due to manual coating. Fig. 1(b) shows the SEM image of the TiCl4-treated FTO/TiO2 anode, and the number of pores has been reduced because of post-TiCl4 treatment.

SEM image of (a) FTO (fluorine doped tin oxide)/TiO2 anode and (b) TiCl4-treated FTO/TiO2 anode. SEM, scanning electron microscopy.
3.2 Fourier-transform infrared characterization
To investigate the chemical structure and absorbance of the natural dye pigments absorbed by TiO2, FTIR 7800 Spectrometer was employed in the region of 4000–400 cm−1 (shown in Fig. 2).

FT-IR absorption spectra of red (R), yellow (Y), combination C4, and combination C10 dyes absorbed by the TiO2 film. FT-IR, Fourier-transform infrared characterization.
For the TiO2 film sensitized with natural red dye (anthocyanin dye), the absorption of Ti–O–Ti vibration appeared at the peak at 611 cm−1 [23]. The peaks at 788 cm−1, 1053 cm−1, 1358 cm−1, and 1626 cm−1 were attributed to the stretching of strong bending vibration of alkene (=C–H), stretching vibration of ether (CO–O–CO) [24,25], stretching of amine (C–N) [26], and variable stretching vibration of alkene (CC) [27] functional groups, respectively. The peaks at 2862 cm−1 and 2972 cm−1 were attributed to the strong asymmetric stretching vibration of alkane (C–H) functional group [28]. The broad and wide adsorption peak at 3283 cm−1 was attributed to the broad and strong stretching vibration of the alcohol (–OH) group [24,29].
For the TiO2 film sensitized with natural yellow dye (curcumin dye), the absorption of Ti–O–Ti vibration appeared at a peak at 680 cm−1 [23]. The peaks at 784 cm−1, 1053 cm−1, 1311 cm−1, and 1626 cm−1 were attributed to the stretching of strong bending vibration of alkene (=C–H), stretching vibration of ether (CO–O–CO) [24,25], stretching of amine (C–N) [26], and variable stretching vibration of alkene (CC) [27] functional group, respectively. The peaks at 2855 cm−1 and 2930 cm−1 were attributed to the strong asymmetric stretching vibration of alkane (C–H) functional group [28]. The broad and wide adsorption peak at 3276 cm−1 was attributed to the broad and strong stretching vibration of the alcohol (–OH) group [24,29].
For the TiO2 film sensitized with the C4 combination of dyes (10 ml of red +15 ml of yellow dye), no new bond was formed. A few left (blue) and right (red) shifts were observed when the TiO2 film was sensitized with the combination of dyes, and the value of transmittance changed. But these two different dyes coexist with each other without any kind of chemical reaction or bond. The absorption of the Ti–O–Ti vibration appeared at a peak at 639 cm−1 [23]. The absorption peak of stretching of strong bending vibration of alkenes (=C–H) appeared at 771 cm−1 [24]. The absorption peak of stretching vibration of ether (CO–O–CO) was visible at 1032 cm−1 [24,25]. The stretching of amine (C–N) appeared at 1315 cm−1 [26]. The variable stretching vibration of alkene (CC) appeared at 1642 cm−1 [27]. The strong asymmetric stretching vibration of the alkane (C–H) functional group was visible at 2924 cm−1 [28]. The broad and strong stretching vibration of the alcohol (–OH) group was attributed to the adsorption peak at 3349 cm-1 [24,29].
Similar to the TiO2 film sensitized with the C4 combination of dyes (10 ml of red +15 ml of yellow), for the TiO2 film sensitized with the C10 combination of dyes (20 ml of red +80 ml of yellow), no new bond was formed. In addition, a few left (blue) and right (red) shifts were observed. For the TiO2 film sensitized with C10 combination of dyes (20% red +80% yellow), the absorption of Ti–O–Ti vibration appeared at a peak at 632 cm−1 [23]. The peaks at 767 cm−1, 1355 cm−1, and 1633 cm−1 were attributed to the stretching of strong bending vibration of alkene (=C–H), stretching vibration of ether (CO–O–CO) [24,25], stretching of amine (C–N) [26], and variable stretching vibration of alkene (CC) [27] functional group, respectively. The peaks at 2862 cm−1 and 2964 cm−1 were attributed to the strong asymmetric stretching vibration of the alkane (C–H) functional group [28]. The broad and wide adsorption peak at 3287 cm-1 was attributed to the broad and strong stretching vibration of the alcohol (–OH) group [24,29].
3.3 UV-vis spectroscopy
Fig. 3 illustrates the absorption spectra of individual red (R) dye, yellow (Y) dye, and the combinations of dyes (C4 and C10), which were measured using a UV-vis spectrophotometer (V650; Jasco) in the region of 350 nm–800 nm. Curcumin is the main chemical ingredient in turmeric extract and appears in bright yellow. The absorption spectrum of ethanol-extracted yellow dye was found in the range of 350–520 nm which has a strong absorption peak at 427 nm. Red spinach is recognized to be rich in anthocyanin dye. The absorption spectrum of DI water-extracted red dye was found in the range of 400–600 nm, which has a peak of absorption at 524 nm. The C4 combination of dyes has higher absorbance and cumulative absorption properties over the entire visible region, which indicates that the combination of dyes absorbs light better than the individual red or yellow dyes. The C4 combination of dyes has an absorption range of 350–600 nm, and the absorption peak shifted to 430 nm [11]. Similar to the absorption spectrum of the C4 combination of dyes, the C10 combination of dyes also shows cumulative absorption properties over the entire visible region of the individual red and yellow dyes. The C10 combination of dyes has an absorption range of 350–600 nm, and the absorption peak shifted to 421.2 nm.
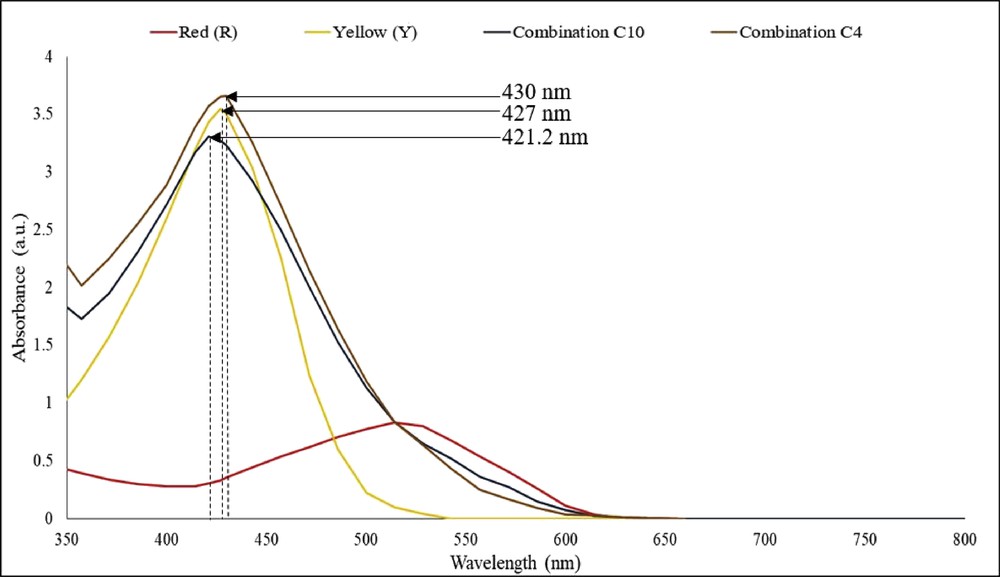
UV-visible absorption spectra of red (R), yellow (Y), combination C4, and combination C10 dyes.
Fig. 4 illustrates the UV-vis absorption spectra of the individual red dye, individual yellow dye, combination C4, and combination C10 dyes absorbed by the TiO2 film under visible wavelength in the region of 350 nm–800 nm. New bonds were formed between the dye molecules and TiO2 film. As a result, the number of obtainable electrons was increased and less energy is required to transfer an electron from the highest occupied molecular orbital (HOMO) to the lowest unoccupied molecular orbital (LUMO) [29]. Because of less energy requirement, absorption peak for the TiO2 film that absorbed red dye (from 524 nm to 580 nm), the C4 combination of dyes (from 430 nm to 432 nm), and the C10 combination of dyes (from 421.2 nm to 424 nm) was shifted to the right (redshift).
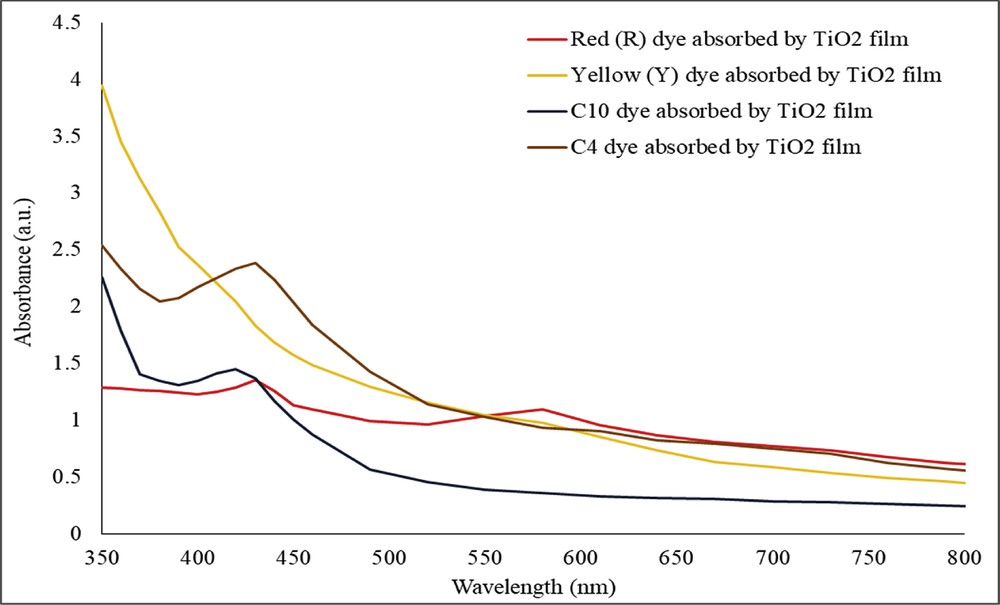
UV-visible absorption spectra of red (R), yellow (Y), combination C4, and combination C10 dyes absorbed by the TiO2 film.
3.4 Photovoltaic properties of DSSCs
I-V performance of DSSCs (fabricated with both FTO/TiO2 and TiCl4-treated FTO/TiO2) sensitized with individual red (R) dye and individual yellow (Y) dye and cosensitized with 10 other combinations of red and yellow dyes was measured using a calibrated solar simulator with a 500 W Xenon arc lamp and a light intensity of 100 mW/cm2 at AM 1.5G. The short-circuit current (Isc) and open-circuit voltage (Voc) were measured experimentally. Pmax (Eq. 1) and fill factor (FF) (Eq. 2) were measured from the I−V characteristics curve, and the cell efficiency was measured by using Eq. 3 [11,30]:
Pmax = Vmax × Imax | (1) |
(2) |
and
(3) |
Table 1 lists all the cell parameters such as Isc, Voc, FF, and η of DSSCs (fabricated with bare FTO/TiO2, i.e. without TiCl4 treatment) sensitized with red dye and yellow dye and cosensitized with five different combinations of red and yellow dyes (C1, C2, C3, C4, and C5). From Table 1, DSSCs sensitized with the combinations of dyes have higher Isc, Voc, and FF than the DSSCs sensitized with individual red or yellow dyes. From Table-1, DSSCs sensitized with 100% natural red (R) dye and yellow (Y) dye show Isc of 2.493 mA and 3.778 mA, respectively. By increasing the percentage of yellow dye in the combination, the Isc of DSSCs can be increased until the value of Isc reaches the maximum. Every combination of dye-sensitized DSSCs has a higher Isc than the 100% red dye-sensitized DSSCs. However, the highest Isc of 5.343 mA was achieved when DSSCs are cosensitized with the optimum dye combination, C4 (40% red +60% yellow). The value of FF varied from 0.442 to 0.507. Individual red dye-sensitized DSSCs (FTO/TiO2) had the lowest cell efficiency of 0.416% compared with other (yellow and C1–C5 dyes) dyes–sensitized DSSCs. DSSCs cosensitized with combinations of dyes (C1–C5) showed higher photovoltaic performance than DSSCs sensitized with the individual natural red (R) dye. Individual yellow dye-sensitized DSSCs (bare FTO/TiO2) have a cell efficiency of 0.921%. By combining yellow dye with red dye, the maximum cell efficiency of 1.267% was achieved for the optimized combination of dyes (C4), which is about 1.3 and 3 times higher than that of the individual yellow dye- and red dye-sensitized DSSCs, respectively.
I-V performance of DSSCs fabricated with FTO/TiO2 (without water effect).
Dye/Combination of dyes | Voc (mV) | Isc (mA) | FF | η% |
R | 356.2 ± 19.3 | 2.493 ± 0.035 | 0.469 ± 0.002 | 0.416 ± 0.030 |
C1 | 375.7 ± 26.5 | 3.643 ± 0.052 | 0.447 ± 0.004 | 0.612 ± 0.058 |
C2 | 384.2 ± 25.4 | 4.438 ± 0.063 | 0.464 ± 0.002 | 0.791 ± 0.067 |
C3 | 431.9 ± 18.7 | 4.925 ± 0.065 | 0.442 ± 0.003 | 0.940 ± 0.060 |
C4 | 493.1 ± 17.1 | 5.343 ± 0.081 | 0.481 ± 0.002 | 1.267 ± 0.069 |
C5 | 481.6 ± 20.5 | 4.753 ± 0.089 | 0.446 ± 0.001 | 1.020 ± 0.065 |
Y | 512.3 ± 12.2 | 3.778 ± 0.056 | 0.476 ± 0.005 | 0.921 ± 0.045 |
Table 2 lists all the cell parameters such as Isc, Voc, FF, and η of DSSCs (fabricated with TiCl4-treated FTO/TiO2) sensitized with red dye and yellow dye and cosensitized with five different combinations of red and yellow dyes (C1, C2, C3, C4, and C5). Comparing Tables 1 and 2, a considerable amount of enhancement is observed in Isc (about 9.1–15.4% improvement) and FF (around 8.4–14.5% improvement). Treating FTO/TiO2 with TiCl4 can reduce electron recombination of the redox couple of liquid electrolyte (I−/I3−) at the TCO/electrolyte interface [20–22,30–33]. In addition, using post-TiCl4 treatment on the FTO/TiO2 substrate can reduce the electron charge carrier leakage and also decrease the amount of confined electrons. As a result, photogenerated electron collection can be increased significantly, and thus, the value of Isc is increased. In addition, post-TiCl4 treatment on the FTO/TiO2 substrate increases absorption of the dye molecules in the photoanode [20–22]. Comparing Tables 1 and 2, TiCl4-treated FTO/TiO2 results in about 24–31.3% improvement in cell performance.
I-V performance of DSSCs fabricated with TiCl4-treated FTO ( Fluorine doped tine oxide )/TiO2 (without water effect).
Dye/combination of dyes | Voc (mV) | Isc (mA) | FF | η% |
R | 358.3 ± 28.4 | 2.876 ± 0.074 | 0.518 ± 0.001 | 0.534 ± 0.058 |
C1 | 380.5 ± 14.6 | 4.156 ± 0.035 | 0.488 ± 0.004 | 0.772 ± 0.042 |
C2 | 392.6 ± 19.5 | 5.028 ± 0.083 | 0.502 ± 0.002 | 0.993 ± 0.070 |
C3 | 438.8 ± 17.9 | 5.378 ± 0.112 | 0.503 ± 0.001 | 1.189 ± 0.076 |
C4 | 498.5 ± 19.3 | 5.829 ± 0.049 | 0.541 ± 0.001 | 1.572 ± 0.077 |
C5 | 487.3 ± 22.6 | 5.385 ± 0.026 | 0.510 ± 0.003 | 1.340 ± 0.077 |
Y | 516.9 ± 12.5 | 4.174 ± 0.035 | 0.519 ± 0.003 | 1.121 ± 0.043 |
Table 3 lists all the cell parameters such as Isc, Voc, FF, and η of DSSCs (fabricated with bare FTO/TiO2) sensitized with red dye and yellow dye and cosensitized with five different combinations of red and yellow dyes (C6, C7, C8, C9, and C10) by keeping the amount of red dye fixed (20 ml) and changing the volume of yellow dye from 5 ml to 20 ml to study the possible effect of water content. Comparing Tables 1 and 3, it is apparent that all the cell parameters (Isc, Voc, and FF) were decreased. Although the combination ratio of the red and yellow dyes is the same (Tables 1 and 3), by keeping the amount of red dye fixed in the combination, the cell performance is greatly decreased. The cell performance was reduced by about 29.7%–35.3%, and this may be due to the effect of water. From Table 3, it is evident that as the volume of the yellow dye is increased in the combination, the cell efficiency is also increased. From Table 3, the maximum cell efficiency of 0.935% was obtained for the C10 dye combination.
I-V performance of DSSCs fabricated with FTO/TiO2 (with water effect).
Dye/combination of dyes | Voc (mV) | Isc (mA) | FF | η% |
R | 349.6 ± 12.5 | 2.473 ± 0.076 | 0.463 ± 0.002 | 0.400 ± 0.028 |
C6 | 361.3 ± 16.3 | 2.673 ± 0.063 | 0.427 ± 0.006 | 0.412 ± 0.034 |
C7 | 374.2 ± 09.2 | 3.032 ± 0.068 | 0.452 ± 0.003 | 0.512 ± 0.027 |
C8 | 419.5 ± 08.3 | 3.336 ± 0.059 | 0.473 ± 0.001 | 0.661 ± 0.026 |
C9 | 457.8 ± 15.3 | 3.726 ± 0.067 | 0.492 ± 0.006 | 0.839 ± 0.054 |
C10 | 488.2 ± 24.3 | 4.025 ± 0.073 | 0.476 ± 0.008 | 0.935 ± 0.084 |
Y | 505.4 ± 10.4 | 3.734 ± 0.081 | 0.486 ± 0.001 | 0.917 ± 0.041 |
Table 4 lists all the cell parameters such as Isc, Voc, FF, and η of DSSCs (fabricated with TiCl4-treated FTO/TiO2) sensitized with red dye and yellow dye and cosensitized with five different combinations of red and yellow dyes (C6, C7, C8, C9, and C10). With water effect, the maximum cell efficiency of 1.171% was observed for the C10 combination of dyes. From Tables 3 and 4, it is evident that the TiCl4-treated FTO/TiO2 results in about 16–26% improvement in the cell performance compared with the bare FTO/TiO2 DSSCs or FTO/TiO2 DSSCs without TiCl4 treatment. Similar to Table 3, from Table 4, it is observed that as the volume of yellow dye is increased in the combination, the cell efficiency is also increased. Comparing Tables 2 and 4, in TiCl4-treated FTO/TiO2 DSSCs sensitized with the combination of dyes, the cell performance is reduced by about 12%–33.5% because of the effect of water. However, DSSCs fabricated with TiCl4-treated FTO/TiO2 show the less water effect compared with the DSSCs fabricated with bare FTO/TiO2.
I-V performance of DSSCs fabricated with TiCl4-treated FTO/TiO2 (with water effect).
Dye/combination of dyes | Voc (mV) | Isc (mA) | FF | η% |
R | 348.2 ± 15.2 | 2.892 ± 0.042 | 0.502 ± 0.007 | 0.500 ± 0.037 |
C6 | 372.8 ± 13.5 | 2.936 ± 0.087 | 0.513 ± 0.003 | 0.561 ± 0.041 |
C7 | 378.2 ± 19.3 | 3.422 ± 0.072 | 0.515 ± 0.002 | 0.666 ± 0.051 |
C8 | 426.4 ± 14.6 | 3.926 ± 0.053 | 0.529 ± 0.002 | 0.885 ± 0.046 |
C9 | 468.2 ± 11.5 | 4.162 ± 0.037 | 0.537 ± 0.006 | 1.046 ± 0.047 |
C10 | 498.1 ± 17.5 | 4.344 ± 0.051 | 0.541 ± 0.004 | 1.171 ± 0.064 |
Y | 512.5 ± 09.4 | 4.162 ± 0.046 | 0.521 ± 0.006 | 1.111 ± 0.046 |
4 Conclusions
In this study, DSSCs were sensitized with natural red and yellow dyes as well as with five different combinations of these two dyes. From the FT-IR absorption spectroscopy, for the combination of red and yellow dyes, it is obvious that no new bond was formed. In this case, in the combination of dyes, both anthocyanin and curcumin dye molecules coexist with each other without any kind of chemical reaction. From the UV-vis absorption spectroscopy, the combination of dyes has higher absorbance and cumulative absorption properties over the entire visible region, indicating that the combination of dyes absorbs light better than the individual yellow or red dyes. Natural red dye- and natural yellow dye-sensitized DSSCs have the cell efficiency of 0.416% and 0.921%, respectively. DSSCs sensitized with the combinations of dyes showed higher cell parameters than the DSSCs sensitized with individual yellow or red dye sensitization. The cell efficiency increased significantly when the DSSCs were cosensitized with the combination of dyes. The maximum cell efficiency of 1.267% was observed for the optimized combination of 10 ml of red + 15 ml of yellow dyes (60% yellow + 40% red), which is about 1.3 and 3 times higher than that of the individual yellow dye- and individual red dye-sensitized DSSCs, respectively. In addition, the effect of post-TiCl4 treatment in the cell was observed. From the experimental data, post-TiCl4-treated FTO/TiO2 results in a noticeable increase in the cell parameters such as the short-circuit current (about 9.1–15.4% improvement), FF (around 8.4–14.5% improvement), and cell efficiency (about 24–31.3% improvement). DSSCs fabricated with TiCl4-treated FTO/TiO2 has the maximum cell efficiency of 1.572% obtained for the optimized dye combination C4 (40% red +60% yellow). Finally, the effect of water content in the combination of dyes was observed. There is a significant effect of the presence of water (in the dye combination), which affects the performance of DSSCs. Around 12%–36% less cell efficiency has been observed because of the presence of water in the dye combination.
Acknowledgements
This research work is financed by the Ministry of Science & Technology, Government of the Peoples Republic of Bangladesh, under special Allocation Research project for Science & Technology (Ref. No.: 39.009.006.01.00.057.2015-2016/PHY’S-370/1299 Date: 08/12/2015).