1. Introduction
Microtubules (MTs) are dynamic cytoskeletal polymers that are involved in intracellular trafficking, cell shape establishment, cell movements and the segregation of condensed chromosomes during mitosis. They are composed of α–β tubulin heterodimers and their polymerization exhibits non-equilibrium dynamics, characterized by periods of polymerization and of depolymerization.
The organization and stability of MTs is tightly regulated by numerous cellular factors such as XMAP215/Dis1/TOGp, MCAK, MAP4, end-binding proteins or Op18/stathmine [1, 2, 3]. Targeted perturbation of this finely tuned process constitutes a major therapeutic strategy. Drugs that interfere with tubulin and MTs are, indeed, key components of combination chemotherapies for the treatment of carcinomas [4, 5, 6]. Vinca-alkaloids and taxanes are among the microtubule targeting drugs (MTD) commonly used in cancer chemotherapy [4, 7].
Drugs that interfere with the tubulin/MT system are roughly classified into MT stabilizing agents such as taxanes, and MT destabilizing agents, such as combretastatin and vinblastine. Regarding MT destabilizing agents, different binding sites located on the β-tubulin subunit have been identified for colchicine, vinca-alkaloids and maytansine [7]. The colchicine site is located at the intradimer interface [8, 9, 10]. It is a large site, mostly buried in the β-tubulin subunit. Besides colchicine, numerous drugs bind to this site, such as nocodazole or combretastatin, but no ligand is large enough to occupy the entire site [6]. Colchicine-site ligands destabilize MTs by preventing the curved-to-straight conformational transition within the α–β tubulin heterodimer [7, 11]. The vinca site is located at the inter-dimer interface between two longitudinally aligned tubulin dimers. Vinca-site agents destabilize MTs by introducing a wedge at the interface between two longitudinally aligned tubulin dimers at the tip of MTs, or by stabilizing assembly-incompetent ring-like oligomers of tubulin [7, 12]. On the contrary, taxanes bind to a pocket of β-tubulin located on the luminal side of microtubules [7, 13, 14].
Recently, we have described two original assays that allow the quantitative evaluation of the depolymerizing or stabilizing effect of MT targeting drugs (MTDs) on cellular MTs [15, 16]. These assays are different from the biochemical tests classically performed in vitro on purified tubulin. They have the advantage of providing information on the effects of MTDs in a cellular context. They allow the quantification of the cell MT mass which is measured by immunoluminescence after the use of a particular lysis buffer, which eliminates free tubulin and preserves intact cellular MTs. These assays are simple to use since they are based on a luminescence reading. Thus, for these assays, only a microplate reader is required and no microscopic analysis is needed.
We have recently conducted a systematic comparative analysis of the effect of four well-characterized MTDs on the kinetics of in vitro tubulin assembly, on the quantity of cellular MTs in HeLa cells (a cervical cancer cell line) using our assay and on HeLa cell viability. We found that there was no significant correlation between the activity of the different drugs on tubulin assembly in vitro and their activity in cells, indicating that the effect of drugs on pure tubulin may be different from their effect on MTs in the cell context. In contrast, we observed a striking similarity between the profile of the viability curves and that of the curves measuring the stability of cellular MTs. This strongly suggests that the cytotoxic effect of the drugs is due to their depolymerizing effect on cellular MTs [15]. These results highlight the value of these cellular microtubule assays for predicting drug therapeutic activity.
Here, we used these cellular MT assays to compare the effect of MTDs, used in cancer chemotherapy, on 3 human breast cell lines known to differ in invasiveness. The cell lines used were MCF-10A cells, isolated from human fibrocystic breast tissue [17], MCF-7, a poorly invasive cell line [18], and MDA-MB-231, an invasive breast cancer cell line [19] (Table 1).
Characteristics of the cell lines used in this study
Cell line | MCF-10A | MCF-7 | MDA-MB-231 |
---|---|---|---|
Disease | Fibrocystic disease | Adenocarcinoma | Adenocarcinoma |
Origin of cells | Spontaneously immortalized cell line | Metastasis (pleural effusion) | Metastasis (pleural effusion) |
Cell type | Epithelial cell | Epithelial cell | Epithelial cell |
Phenotype | Normal-like | Luminal A | Claudin-low |
ER | No | Yes | No |
PR | No | Yes | No |
HER2 amplification | No | No | No |
Tumorigenic in mice | Non-tumorigenic | Yes, with estrogen supplementation | Yes |
Appearance | Groups of adherent epithelial cells | Loosely attached three-dimensional clusters | Epithelial-like somewhat spindle-shaped |
Model | Non-malignant breast cell line | Transformed estrogen responsive breast cancer cell line | Late-stage breast cancer |
ER, estrogen receptor; PR, progesterone receptor; HER2, human epidermal growth factor receptor 2.
The assayed drugs (Figure 1) are two MT depolymerizing drugs, vinblastine and combretastatin-A4 (CA-4) and a MT stabilizing drug, paclitaxel (PTX).
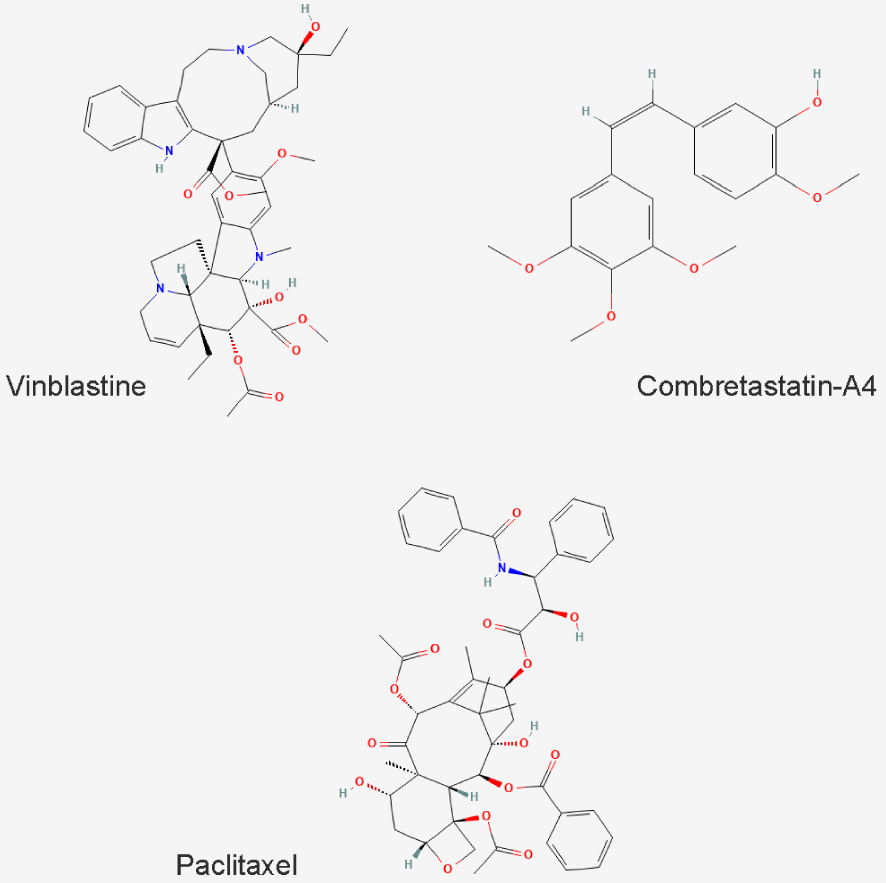
Chemical structure of the MTDs used in this study.
Vinblastine binds to the vinca site of tubulin, whereas CA-4 binds to the colchicine site and PTX to the taxane site.
Although these compounds all target microtubules, they differ in their applications: whereas vinblastine and PTX are used in antitumor chemotherapy [4, 7, 20], CA-4 is an anti-neoangiogenesis agent [21].
2. Material and methods
2.1. Chemical reagents and cells
MCF-10A (CRL-10317), derived from fibrocystic breast tissue, and MCF-7 (HTB-22) and MDA-MB-231 (HTB-26) cells, derived from the pleural effusion of a metastatic breast adenocarcinoma, were obtained from the American Type Culture Collection (ATCC, Gainthersburg, MD, USA). Cell culture was performed according to the recommendations of the American Type Cell Culture. MCF-10A cells were grown in DMEM/F12 (Gibco 10565-018) supplemented with 5% horse serum (Gibco 16050-122), EGF (Peprotech AF-100-15) at 0.1 mg/ml, hydrocortisone (H0888) at 1 mg/ml, cholera toxin (C8052) at 1 mg/ml, insulin (I1882) at 10 mg/ml and 1% penicillin/streptomycin. MCF-7 cells were grown in MEM medium (Gibco 31095-029) supplemented with insulin at 0.1 mg/ml, 1% penicillin/streptomycin and 10% FBS. MDA-MB-231 cells were grown in DMEM Glutamax medium (Gibco 31966-021) and supplemented with 10% FBS and 1% penicillin/streptomycin. Cells were maintained in a humid incubator at 37 °C in 5% CO2.
All media were purchased from Gibco Invitrogen (Carlsbad, CA, USA). All chemicals, except those for which it is specified, were purchased from Sigma-Aldrich (Saint-Quentin-Fallavier, France).
Combretastatin-A4 (C7744), vinblastine (V1377) and paclitaxel (T7402) were prepared at a 10 mM stock solution in Dimethyl sulfoxide (DMSO, #4540) aliquoted and stored at −20 °C.
2.1.1. Quantitative assay of the effect of depolymerising agents on the cellular microtubule content
Cells were seeded in 96-well microplates (#655086, Greiner bio One, Courtaboeuf, France) at the density of 5000 MCF-10A cells, 10,000 MCF-7 cells and 7500 MDA-MB-231 cells per well in 100 μl of complete medium and then incubated at 37 °C in 5% CO2 for 24 h. Cells were then treated for 30 min at 37 °C with the compounds at concentrations ranging from 1 to 5000 nM (1 microplate per molecule, 1 concentration per column), with 0.1% DMSO used as positive control (6 wells per microplate). After medium aspiration, treated cells were permeabilized for 10 min using 100 μl per well of warmed (37 °C) OPT buffer (80 mM Pipes, 1 mM EGTA, 1 mM MgCl2, 0.5% Triton X-100, and 10% glycerol, pH 6.8). Cells were fixed for 10 min at room temperature using 100 μl per well of 4% formaldehyde (Sigma Aldrich, #252549, Saint-Quentin-Fallavier, France) in PBS. Cells were washed 3 times in PBS (100 μl per well), then 50 μl of primary anti-alpha-tubulin antibody (clone α3A1 [22], 1:5000 in PBS pH 7.4, 0.1% Tween-20 2% Bovine Serum Albumin (BSA)) was added for 45 min. Cells were washed twice again and secondary anti-mouse antibody coupled to HRP (1:2000 in PBS pH 7.4, 0.1% Tween-20 2% BSA, #715-035-150, Jackson Immuno-Research Laboratories, Cambridgeshire, UK) was added for 45 min. Then, cells were washed again with PBS and 100 μl of ECL substrate (#170-5061, Bio-Rad Laboratories Inc., USA) were injected in each well using the FLUOstar OPTIMA Microplate Reader (BMG Lab technology, Champagny-sur-Marne, France). The luminescent signal was read 5 min after ECL injection. IC50s, i.e., drug concentrations able to reduce the amount of cellular microtubules by half, were calculated for each independent experiment using GraphPad Prism software and are presented in the text as means ± SEM.
2.1.2. Quantitative assay of the stabilizing effect of paclitaxel on cellular microtubule
The same quantity of cells was seeded in microplates as for the quantitative assay of the effect of depolymerizing agents on the cellular microtubule. 24 h after seeding, cells were treated with paclitaxel at different concentrations (range 0–5000 nM). DMSO alone at 0.25% was used as positive controls (6 wells per microplate and per control), respectively. Then combretastatin-A4 was added to each well at 50 nM final concentration for 30 min. After medium aspiration, treated cells were permeabilized for 10 min using 100 μl per well of OPT buffer (80 mM Pipes, 1 mM EGTA, 1 mM MgCl2, 0.5% Triton X-100, and 10% glycerol, pH 6.8) pre-warmed to 37 °C. After buffer aspiration, cells were fixed for 10 min at room temperature using 100 μl per well of 4% formaldehyde in PBS pH 7.2. Cells were washed 3 times with PBS 0.1% Tween-20 (150 μl per well), then 50 μl of α3A1 anti-tubulin antibody (1:5000 in PBS pH 7.4, 0.1% Tween-20 2% BSA) were added for 45 min. After washing of cells as described above, 50 μl of anti-mouse antibody coupled to HRP (1/2000 in PBS pH 7.4, 0.1% Tween-20 2% BSA) were added for 45 min. Then cells were washed again and, 50 μl of ECL Western blotting substrate (Pierce #32106) were added to each well and the luminescent signal was read after 5 min of incubation.
2.1.3. Immunofluorescence
Cells at a density of 30,000 cells per well for MCF-10A, 60,000 for MCF-7 and 50,000 for MDA-MB-231 were grown for 48 h on glass coverslips placed in a 24-well microplate. When cells reached 70% confluence, the medium was replaced with a fresh one supplemented with DMSO (0.005% or 0.01%) or the test compound at 50 nM for vinblastine and CA-4 or 1000 nM for PTX. After 30 min of incubation, cells were permeabilized in warm OPT buffer (80 mmol/L Pipes, 1 mol/L EGTA, 1 mol/L MgCl2, 0.5% Triton X-100 and 10% Glycerol, pH 6.8) and fixed for 6 min in −20 °C methanol (Carlo ERBA SAS, #414855, Val-de-Reuil, France). After washing and saturation with a specific blocking buffer (3% BSA), 10% Goat serum (Gibco Invitrogen, #16210064, Carlsbad, CA, USA) in PBS, cells were incubated for 45 min at room temperature (RT) with anti-alpha-tubulin antibody (clone α3A1 [22] in blocking buffer). Cells were washed twice again and subsequently incubated with Alexa 488 conjugated anti-mouse antibody (1:500 in blocking buffer, #115-545-166, Jackson immune-research laboratory, Cambridgeshire, UK) for 30 min at RT. Coverslips were mounted on glass slides with Moviol 4–88.
Images were captured with a Zeiss AxioimagerM2 microscope equipped with the acquisition software AxioVision (Marly-le-Roi, France).
3. Results
3.1. Effect of the depolymerizing agents Vinblastine and CA-4 on the MT contents of breast cancer cell lines
We first compared the effect of different doses of vinblastine and CA-4 on cellular interphase MTs. After having determined a seeding density adapted to each cell line, we used our recently developed cell-based assay that quantifies intact MTs in cells [15, 16]. The principle of this assay is based on the use of a particular lysis buffer, which eliminates free tubulin and preserves intact cellular MTs, which are then quantified by immunoluminescence. The detailed protocol is given in the methods section.
As shown on Figure 2, both compounds induced a dose-dependent depolymerization of cellular MTs, on the 3 cell lines. The IC50 for CA-4 was in the same range for MCF-10A and MDA-MB-231, i.e., 6.6 ± 0.4 nM and 4.8 ± 0.3 respectively. This IC50 was found much higher in MCF-7 cells, i.e., 31.1 ± 7.9 nM. Similar results were obtained for vinblastine: the IC50 was 2.8 ± 0.4 for MCF-10A, 67.7 ± 15.4 for MCF-7 and 4.4 ± 0.4 for MDA-MB-231.

Comparison of the MT destabilizing effect of Vinblastine and CA-4. Different doses of compounds were applied to the different cells in microplates and their MT destabilizing effect was assessed after a 30 min incubation, using the luminescent assay, as described in the Materials and Methods section. Results are expressed as % of resistant MTs, with 100% corresponding to cells treated with DMSO only, without the depolymerizing agent. Datapoints are means ± SEM from three independent experiments.
Interestingly, in MCF-10A and MDA-MB-231 cells, the sigmoid curve of CA-4 has a much steeper slope than that of vinblastine, indicating that a small variation of the concentration around the IC50 can lead to a complete depolymerization of the microtubule network. The effect of increasing concentrations of vinblastine is much more gradual on these cell lines.
On MCF-7 cells, both compounds behave similarly. On this cell line, unlike the other two cell lines, a complete depolymerization of the MT network was not observed, even at the highest doses (5000 nM) assayed.
3.2. Effect of the stabilizing agent paclitaxel on the microtubule contents of breast cancer cell lines
To measure the effect of PTX on cellular MTs, we used a variant of the test presented above for depolymerizing agents. The assay probes a PTX-induced resistance of the MT network to a depolymerization provoked by CA-4. CA-4 binds free tubulin dimers and prevents their incorporation into MTs, leading to a progressive loss of the polymerized MT network. Stabilized MTs with slow dynamics have reduced exchanges of their tubulin content with the free tubulin pool, and are thus less sensitive to CA-4-induced depolymerization [23]. After treatment with the drugs and before fixation, the same lysis buffer as described above is used in order to eliminate free tubulin and to preserve the cellular MTs, which are then quantified by immunoluminescence. The detailed protocol is given in the methods section. This assay is sensitive as it allows to detect a weak stabilizing effect, quantitative and statistically robust [16].
Figure 3 shows the dose-effect curves of the sensibility of the MT network of the different cell lines to the stabilizing effect of PTX against a depolymerization induced by 50 nM CA-4. This concentration of CA-4 is able to induce the depolymerization of the entire MT network in MCF-10A and MDA-MB-231 cells and only 50% of the network in MCF-7 cells (see blue curves in Figure 2, and ordinate at the origin in Figure 3). For MCF-10A and MDA-MB-231 cells, a similar dose of PTX, 246.9 ± 83.9 nM and 234.3 ± 32.5 nM respectively, is required to achieve 50% stabilization of the microtubule network. Regarding MCF-7, 16.3 ± 9.0 nM of PTX induced 50% of the effect. For the latter cell line, we tested the stabilizing effect of PTX against a depolymerization induced by 100 times more CA-4, i.e., 5000 nM (not shown). As shown in Figure 2, however, such a high concentration of CA-4 does not fully depolymerize the network. Under these conditions, a similar dose-dependent profile of stabilization by PTX is obtained, with 50% of the stabilizing effect achieved for a PTX concentration of 15.3 ± 6.9 nM.
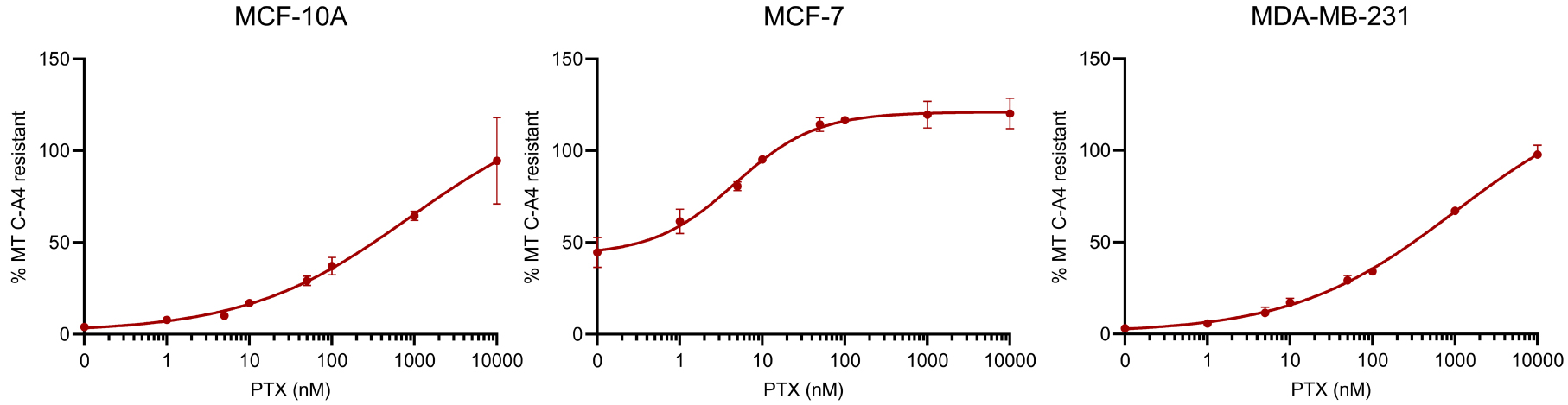
Comparative analysis of the effect of PTX on MT dynamics in breast cancer cells. Different doses of PTX were applied for 2 h to cells in microplates. Thirty minutes before the end of the PTX treatment 50 nM CA-4 was added. At the end of the PTX treatment, its MT stabilizing effect was assessed using the luminescent assay described in the material and methods section. The analyses were performed with a PTX concentration in 0.1% DMSO at the solubility limit for not inducing an additional toxicity due to DMSO. Results are expressed as % of MTs resistant to CA-4-induced depolymerization, with 100% corresponding to cells treated with DMSO without CA-4. Datapoints are means ± SEM from three independent experiments. Masquer
Comparative analysis of the effect of PTX on MT dynamics in breast cancer cells. Different doses of PTX were applied for 2 h to cells in microplates. Thirty minutes before the end of the PTX treatment 50 nM CA-4 was added. At the ... Lire la suite
3.3. Immunofluorescence analysis of the effect of the different drugs on cellular MTs
Using immunofluorescence, we checked that the luminescent values do reflect the state of cellular MTs. As shown in Figure 4, a decrease in the density of the MT network is observed in MCF-10A and MDA-MB-231 cells treated with 50 nM of vinblastine or CA-4, as well as typical microtubule bundle reorganization with 1 μM Paclitaxel treatment. Changes in the shape and density of the MT network are more difficult to observe in MCF-7 cells, which grow in clusters.
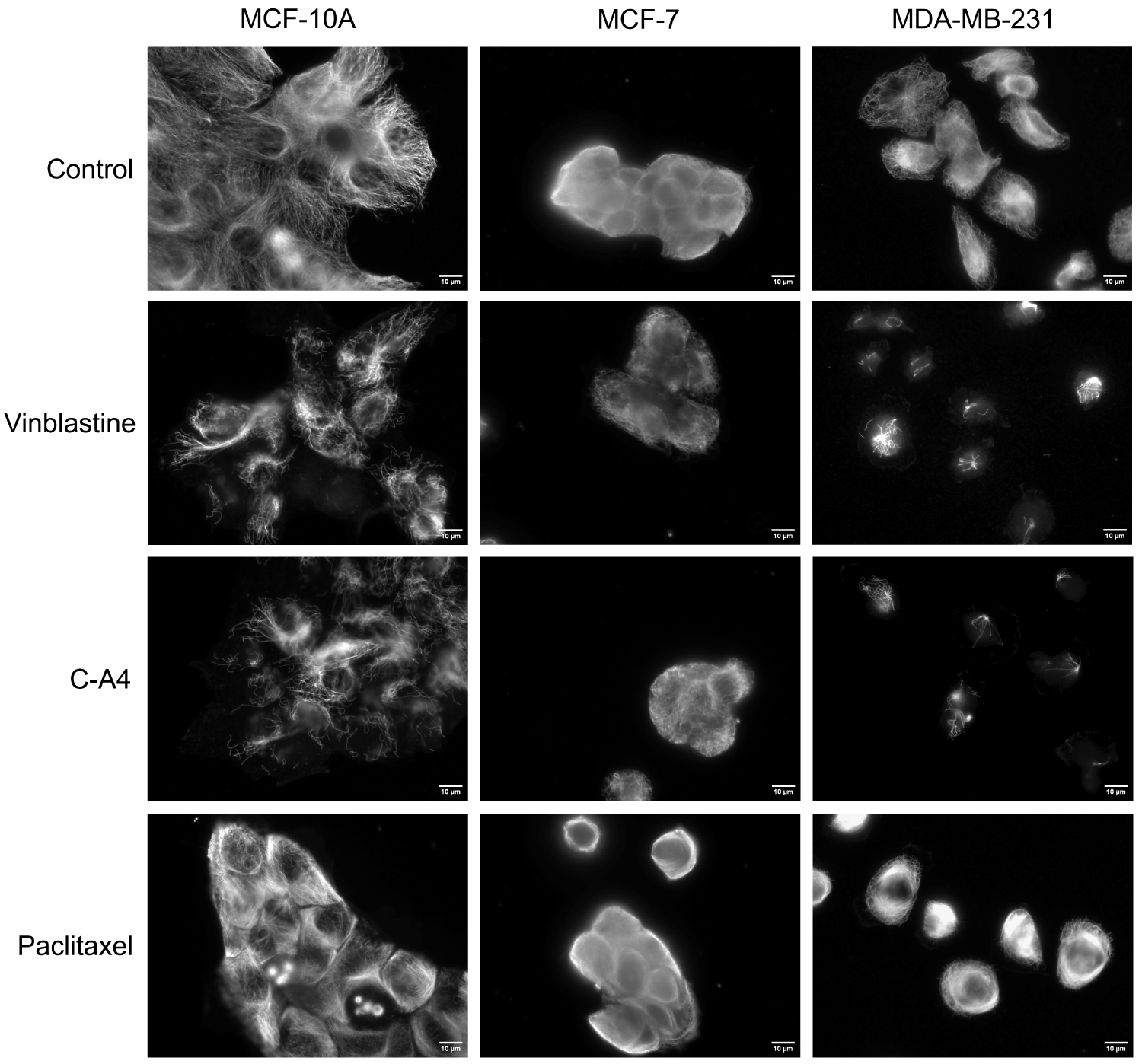
Immunofluorescence analysis of the effect of the different compounds on the MTs of the different breast cancer cells. Cells were incubated for 30 min with 50 nM Vinblastine, 50 nM CA-4, 2 h with 1 μM PTX or with DMSO (control). Cells were then permeabilized with OPT buffer, fixed, and processed for immunofluorescence using an anti-α-tubulin antibody. Scale bars, 10 μm.
4. Discussion and conclusion
The development of agents targeting MTs remains an area of intense research. In this context, quantitative assessment of the compounds’ effect on cellular MTs is essential. With this study, we showed that the quantitative assays we had initially developed using HeLa cells could be easily adapted, for other types of adherent cells by modifying only the density of cell seeding. This allows the investigation of the effect of MTDs on other types of cancer. However, this study highlighted a potential limitation concerning the cell types that can be studied. For example, we could not observe complete depolymerization of the microtubular network with two different drugs on MCF-7 cells. MCF-7 cells are known as drug-sensitive cells, which do not overexpress drug efflux pumps [24]. The reduced efficiency of depolymerizing drugs to act on the microtubule network is therefore not the result of the activation of efflux pumps. One possible reason is that this is a result of poor penetration of the drugs, even when applied at high concentrations, due to the fact that the cells grow in clusters. Increasing the incubation time of the cells with the drugs, which implies additional methodological validations, could be a way to achieve complete MT depolymerization.
Despite this difficulty in achieving complete depolymerization of MTs in MCF-7 cells, this cell line appears to be particularly sensitive to the stabilizing action of PTX, as measured in our assay. Indeed, it requires about 15 times more PTX to stabilize 50% of the MT network for MCF-10A and MDA-MB-231 cells.
We observed that MCF-10A and MDA-MB-231 show similar sensitivity to MTDs, indicating that the invasiveness of MDA-MB-231 does not influence the drug sensitivity of this cell line. This cell line was established from a single sample of pleural effusion obtained from a 51-year-old woman, who had previously a right radical mastectomy for a poorly differentiated tumor. This patient received a systemic treatment with 5-FU and prednisone which were ineffective. Then a combined chemotherapy (cyclophosphamide, adriamycin, and amethopterin) was administered, with no success [19]. Interestingly, this patient has never been treated with drugs targeting MTs, and therefore could not develop resistance, explaining the sensitivity of MDA-MB-231 to MTDs.
It should be noted that the test duration is very short (2 h) and therefore has no detectable effect on cell viability. A limitation of the assay for measuring the effect of depolymerizing agents is that a decrease in luminescent signal can be observed not only when microtubules are depolymerized, but also if the agents tested have an effect on cell adhesion, thus reducing the number of stained cells. False positives of this kind can be identified by checking the effect of the drugs on the cells under the microscope. In this case, measuring the value of the luminescent signal in ratio to the number of residual adherent cells, as measured by nucleus labelling, e.g. with Hoechst, can provide information on the depolymerizing effect alone [25].
Here, we have shown that the quantitative analysis of the effect of MTDs can be easily performed on various cell lines. Ideally, this assay could be used not only for the development of new MTDs [26, 27], but also for the comparative analysis of the response of human tumor samples to different drugs, in order to optimize therapeutic treatment.
Conflicts of interest
Authors have no conflict of interest to declare.
Vous devez vous connecter pour continuer.
S'authentifier