1 Introduction
Recently, photochemical and photoelectrochemical properties of fullerene (C60) have been widely studied [1]. Photoinduced electron-transfer reactions of donor-C60 linked molecules have been also reported [1–7]. In a series of donor-C60 linked systems, some of the compounds show novel properties, which accelerate photoinduced charge separation and decelerate charge recombination [3–5]. Those properties have been explained by the remarkably small reorganization energy in their electron-transfer reactions. The porphyrin-C60 linked compounds, where the porphyrin moieties act as both donors and sensitizers, have been extensively studied [3–5].
It has been reported that C60 and its derivatives formed optically transparent microscopic clusters in mixed solvents [8,9]. The clustering behavior of C60 is mainly associated with the strong three-dimensional hydrophobic interactions between the C60 units. Photoinduced electron-transfer and photoelectrochemical reactions using the C60 clusters have been extensively reported because of the interesting properties of C60 clusters [8,9].
Magnetic field effects (MFEs) on the reaction kinetics or yields of photochemical reactions in the condensed phase have been studied [10–13]. The MFEs have been powerful for verifying the mechanism of photochemical reactions. Previously, we have obtained photogenerated triplet biradicals of donor–acceptor linked compounds, and found that the lifetimes of the biradicals are remarkably extended in the presence of magnetic fields up to 1 T with electromagnet [14–20].
High MFEs on the dynamics of radical pairs or biradicals generated by photochemical reactions have been extensively investigated to establish the measurement of MFEs under high magnetic fields above 1 T [12,13,21–29]. The reverse phenomena in the MFEs have been reported in some radical pairs or biradicals. The reverse phenomena of the MFEs on the lifetime, in the linked biradicals, have been observed only in high magnetic fields above 1 T [23–28].
As to the MFEs in donor-C60 linked systems, C60 has interesting properties. First, C60 has no magnetic nuclei based on hydrogen, and thus there are little hyperfine interactions due to the C60 radical contributing to the MFEs. Second, C60 is a highly symmetric molecule, and therefore anisotropic Zeeman interaction, due to the C60 radical, is very small. Third, the g-value (g = 1.9982) [30] of C60 is smaller than that of the donor radical such as phenothiazine cation radical (g = 2.0052) [31]. Therefore, the difference of g-values between the donor and C60 is expected to be large. On the basis of the three points, the MFEs in various donor-C60 linked compounds are expected to provide useful information for studying the spin chemistry of C60. From these considerations, we recently examined photoinduced electron-transfer reactions and MFEs on the photogenerated biradicals in phenothiazine (Ph)-C60 linked compounds and found the MFEs on the triplet biradicals which were photogenerated from intramolecluar electron-transfer reactions from the Ph to the triplet excited state of C60 (3C60*) in benzonitrile [16–18]. In addition, we have also investigated the MFEs on the photogenerated biradical in a zinc-tetraphenylporphyrin (ZnP)-C60 linked compound. The MFEs verified that the triplet biradicals were generated by the intramolecular electron-transfer from the triplet excited state of ZnP to C60 and/or from ZnP to 3C60* in benzonitrile. Especially, the reverse phenomena in the MFEs occurring around 0.1 T have been found in benzonitrile [19]. Similar reverse phenomena were observed in Ph-C60 linked compounds [17,18]. These unique phenomena may be explained by the contribution of the anisotropic Zeeman interaction to the spin-lattice relaxation (SLR) of the biradical.
The MFEs on the decay of the radical pair between a C60 cluster anion and a pyrene cation have been observed in a micellar system [29]. However, the MFEs on the photoinduced electron-transfer reactions using the C60 cluster in mixed solvents have been studied yet. In the present paper, we have examined photoinduced electron-transfer reaction and MFEs on the dynamics of radical pair generated by the intermolecular electron-transfer reaction between C60 cluster and methyl phenothiazine (MePH).
2 Experimental
Synthetic procedure of a C60 derivative containing a positive charge (C60N+) was prepared according to the previous paper [32] and is shown in Scheme 1. The structure of C60N+ was confirmed by 1H-NMR and MALDI-TOF MS spectra and elemental analysis.
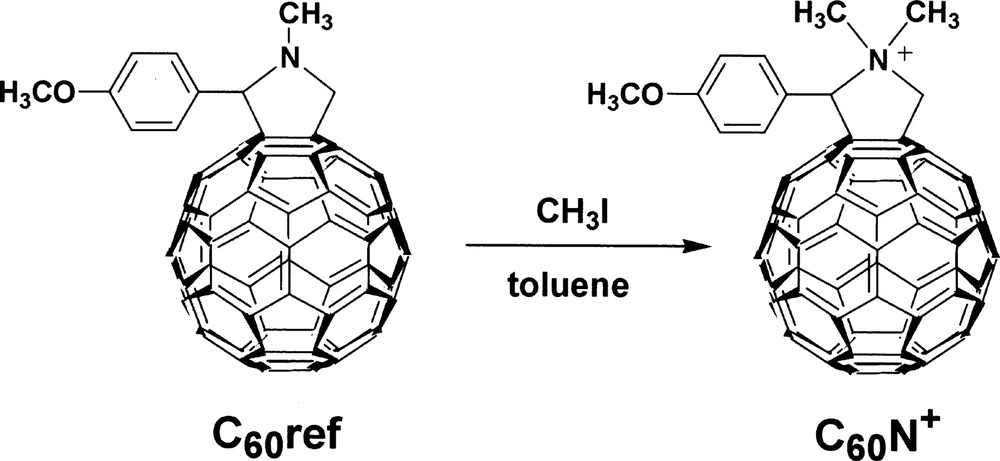
1H-NMR, steady-state absorption, and transient absorption spectra, and MFEs were measured according to the previous papers [16–19]. Methylphenothiazine (MePH) (Wako, extra pure), THF (Dojindo, spectrosol), and DMSO (Dojindo, spectrosol) were used as received.
Clusters of C60N+ were prepared by solving in THF–H2O mixed solvent. C60N+ forms optically transparent clusters by the following procedure. First, the suspension of C60N+ and MePH were sonicated in THF for 15 min. Next, H2O was injected into the suspension by a syringe. After sonication for 15 min, the suspension became optically transparent mixed solvent (THF/H2O = 2:1) solution. The diameter of C60N+ clusters were measured using AFM. The THF–H2O mixed solution of C60N+ or both C60N+ and MePH was dropped on freshly cleaved mica.
After drying at ambient temperature, the AFM images of C60N+ clusters were recorded in tapping mode using a Nanoscope IIIa (Digital Instrument).
For laser photolysis experiments, a THF–H2O mixed solution of C60N+ (0.1 mM), MePH (1 mM), or C60N+ (0.1 mM) and MePH (1 mM) in a cylindrical quartz cell (3.5 cm optical path length) with a water jacket was deoxygenated by passing argon streams. The cell was placed in the gap of an electromagnet and was irradiated by the 532- or 355-nm laser light of a Nd–YAG laser (Continuum Surelite I-10, pulse width 4–6 ns). The transient signals from a photomultiplier (R928 Hamamatsu Photonics Co., Ltd.) with a wide band preamplifier (NF Electronic Instruments BX-31) were recorded by a digital storage oscilloscope (Tektronix TDS350P). The signals were analyzed by a microcomputer.
3 Results and discussion
3.1 Characteristic of the C60N+ clusters
The absorption spectrum of C60N+ in DMSO is shown in Fig. 1a. The spectrum in the region of 400-800 nm is essentially identical with 6,6-closed mono-functionalized C60 derivative [1]. On the contrast, the absorption spectrum of C60N+ in THF/H2O (2:1) mixed solvent (Fig. 1b) was different from that in Fig. 1a. The absorption bands in the 400–800 nm region in the mixed solvent were broadened and became featureless as comparison with that in DMSO (Fig. 1a). The results suggested the formation of cluster of C60N+ occurred in the THF/H2O mixed solvent. In addition, the absorption spectrum of C60N+ (0.1 mM) and MePH (1 mM) in THF/H2O (2:1) mixed solvent was similar to that in Fig. 1b. The result indicated similar formation of cluster of C60N+ occurred in the addition of MePH.
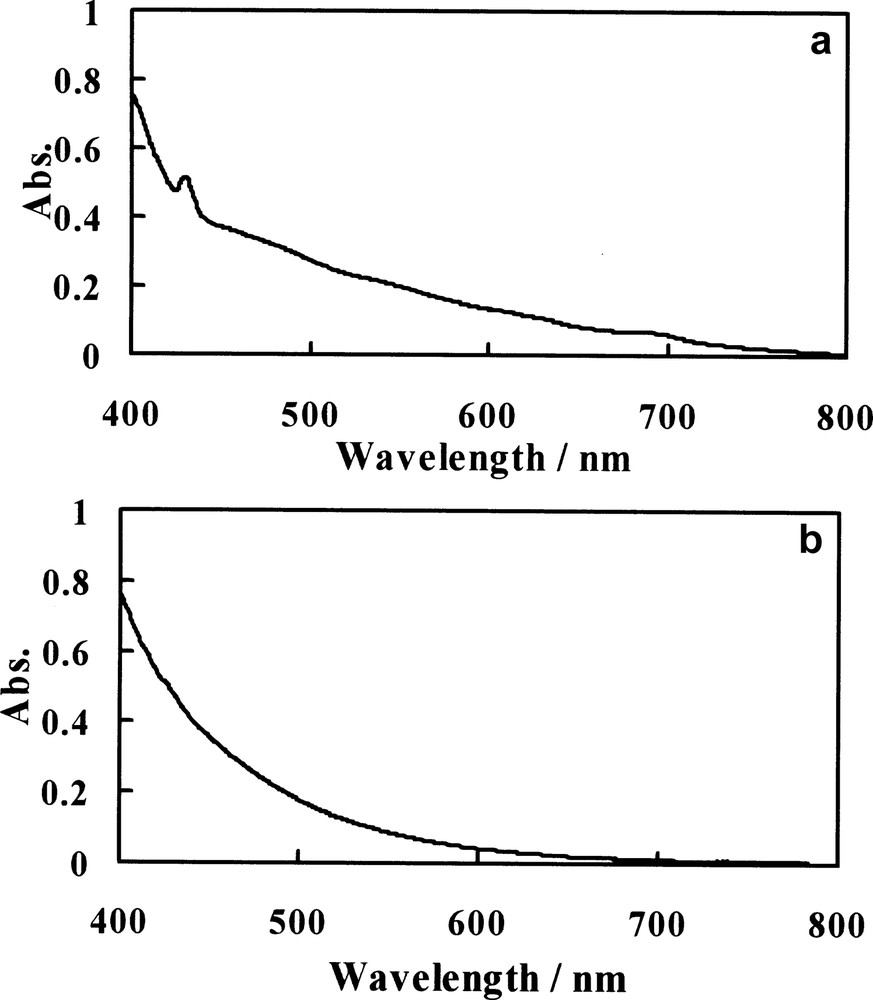
Absorption spectra of (a) C60N+ (0.1 mM) in DMSO and (b) C60N+ (0.1 mM) in THF/H2O (2:1) mixed solvent at room temperature.
The formations of clusters of C60N+ were examined by AFM measurement. From the AFM image (Fig. 2), the diameters of the clusters of C60N+were estimated to be ca. 100 nm. In the case of C60N+ (0.1 mM) and MePH (1 mM) in THF/H2O (2:1) mixed solvent, similar AFM image was observed. The estimated diameters by AFM are similar to those estimated by using of dynamic light scattering (DLS) measurement. On the basis of the absorption spectra, AFM, and DLS, the formations of clusters of C60N+ were confirmed in the THF/H2O (2:1) mixed solution of only C60N+ or C60N+ and MePH.
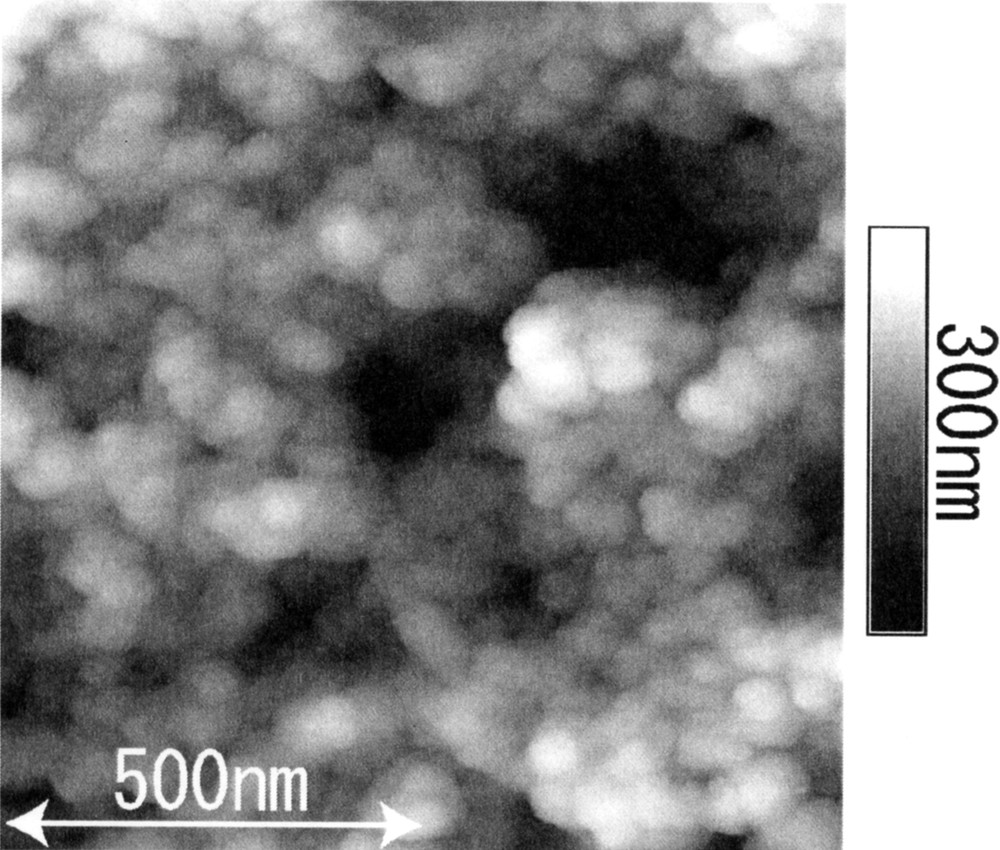
A AFM image of C60N+ cluster obtained from C60N+ (0.1 mM) in THF/H2O (2:1) mixed solvent on a mica surface.
When the DMSO solution of C60N+ was dropped on freshly cleaved mica, the formation of clusters (ca.100 nm) as shown in Fig. 2 was not observed. The result is in agreement with that in the absorption spectrum (Fig. 1a).
3.2 Photoinduced electron transfer in C60N+ clusters–MePH system
Transient absorption spectra of the C60N+ clusters–MePH system were measured following laser excitation at 532 or 355 nm in THF/H2O (2:1) mixed solvent at 298 K. At first, we attempted to measure transient absorption spectrum by the second harmonic light (532 nm). However, no significant transient absorption was observed in nanosecond time-region.
Next, we attempted to measure transient absorption spectra of C60N+ clusters–MePH system in the THF/H2O (2:1) mixed solvent by the third harmonic light (355 nm). The significant transient absorption spectra were clearly observed as shown in Fig. 3a. The broad absorption band in the 450–800-nm region was observed at 1 μs after laser excitation. As a reference system, the transient absorption spectra of MePH in the THF/H2O (2:1) mixed solvent were measured at the same experimental condition. The broad absorption band in the 450–800 nm region was also observed at 1 μs after laser excitation (Fig. 3b). The broad band is assignable to the T1–Tn absorption of the PH moiety. The result indicates that the triplet excited state of PH (3PH*) was generated by laser excitation. The broad band was similar to that at 1 μs in Fig. 3a. Therefore, the broad absorption band around 450 nm at 1 μs in Fig. 3a is mainly ascribed to the T1–Tn absorption of the PH moiety in MePH.
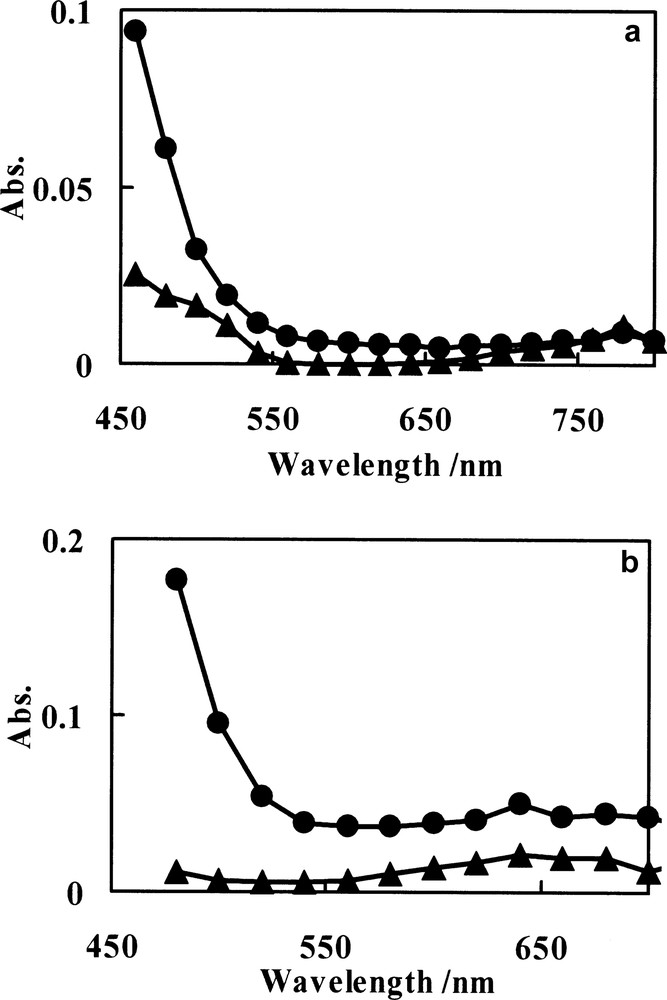
Transient absorption spectra of (a) C60N+ (0.1 mM)–MePH (1 mM) at 1 (●) and 8 (▲) μs and (b) MePH (1 mM) at 1 (●) and 10 (▲) μs in THF/H2O (2:1) mixed solvent at 298 K.
At 8 μs after laser excitation, the broad absorption decayed and then the shoulder band around 520 nm due to phenothiazine cation radical (PH•+) and the absorption bands (λmax, 780 nm) above 650 nm due to C60 anion radical (C60•–) appeared in Fig. 3a. As another reference system, the transient absorption spectrum of only C60N+ cluster in the THF/H2O (2:1) mixed solvent was measured at the same experimental condition. However, no significant transient absorption was observed in nanosecond time-region. On the basis of these observations, the photoinduced intermolecular electron-transfer process (kCS(T)) from 3PH* to C60N+ cluster, (C60N+)n occurred as shown in Scheme 2.
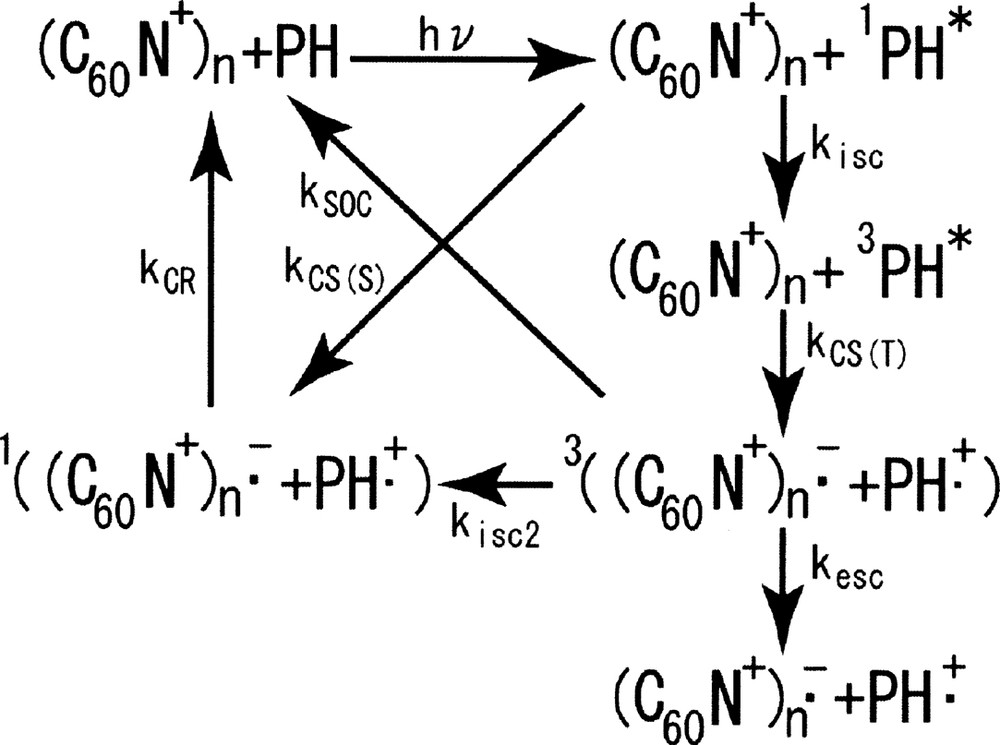
3.3 Magnetic field effects on the dynamics of radical pair in C60N+ clusters–MePH system
MFEs on the dynamics of radical pair in C60N+ clusters–MePH system were examined in THF/H2O (2:1) mixed solvent. MFEs on the decay profiles of the transient absorption at 520 nm due to PH•+ were shown in Fig. 4. The decay was retarded in the presence of magnetic field. In addition, the absorbance at 10 μs after laser excitation increased with the increase of magnetic field. The result indicated that the yield of the escaped PH·+ increased with the increase of magnetic field. Therefore, the magnetic field effects on the decay profile were clearly observed.
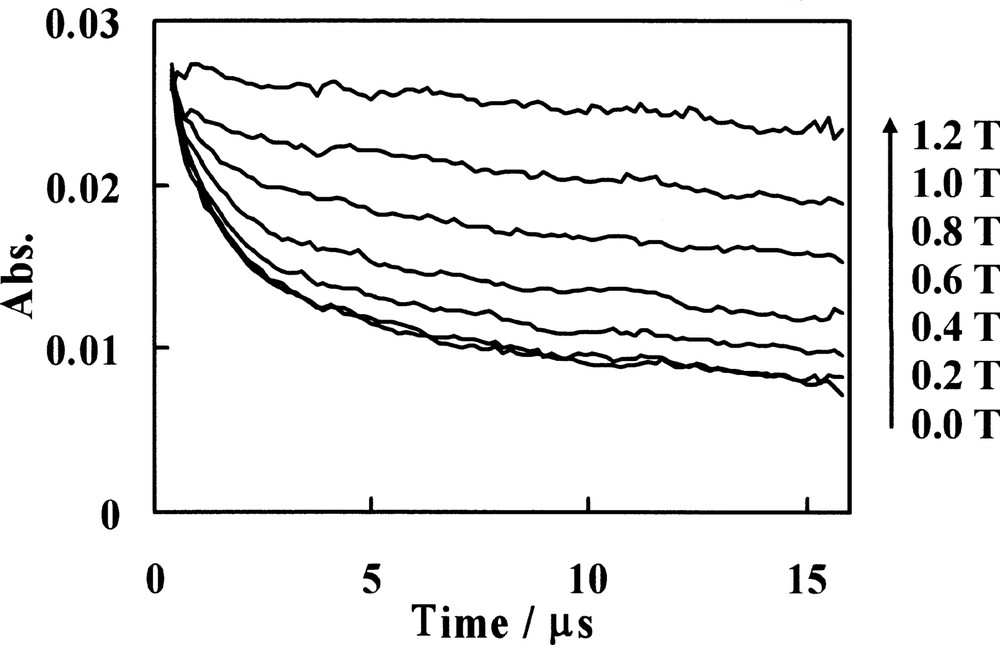
MFEs on the decay profiles of the transient absorption at 520 nm upon laser excitation of C60N+ (0.1 mM)–MePH (1 mM) in THF/H2O (2:1) mixed solvent at 298 K.
The magnitudes of the MFEs were evaluated by the following equation; Δ = Abs(H T)/Abs(0 T), where Abs(H T) and Abs(0 T) represent the absorbance at 10 μs at 520 nm in the presence (H T) and the absence of magnetic field. The Δ-values increased with the increase of magnetic field. The Δ-value became 2.7 at 1.2 T as shown in Fig. 5.
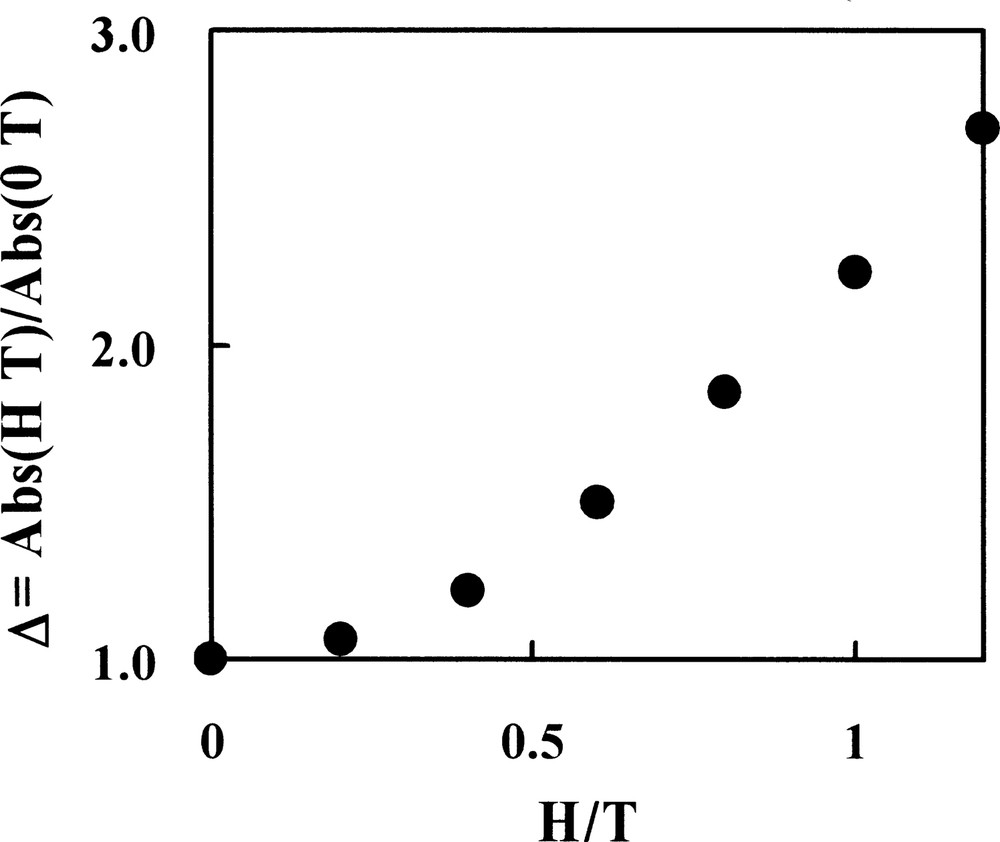
MFEs on Δ = Abs(H T)/Abs(0 T) in C60N+ (0.1 mM)–MePH (1 mM) in THF/H2O (2:1) mixed solvent.
As a reference system of MFEs, we have examined the MFEs on the decay profiles of the transient absorption at 520 nm in the case of the nonlinked system; C60ref and MePH in benzonitrile [33]. In the homogeneous solution, the absorption band due to 3C60* in C60ref was observed around 680 nm and the absorption due to PH•+ at 520 nm initially rose and then decayed in the transient absorption spectra following laser excitation at 532 nm. The decay at 680 nm corresponded well with the rise at 520 nm. The results indicated that the triplet radical pairs were generated by photoinduced intermolecular electron-transfer from PH to 3C60*. However, no MFEs on the decay profile at 520 nm due to PH•+ were observed. The result is ascribed to fast diffusion of the radicals in homogeneous solution. Therefore, the observation of MFEs in the C60N+ clusters–MePH system are most likely ascribed to the cage effect due to the incorporation of MePH into the C60N+ cluster.
The MFEs in C60N+ clusters–MePH system are explained by radical-pair mechanism between C60N+ cluster, (C60N+)n, and MePH, as shown in Scheme 2. MePH is mainly photoexcited by the 355-nm laser light, because of the excess amount of MePH as comparison with C60N+. The singlet excited state of PH (1PH*) is generated by the laser excitation. The intersystem-crossing process (kisc) occurs and 3PH* is generated. The intermolecular electron-transfer process (kCS(T)) from 3PH* to C60N+ cluster, (C60N+)n, occurs and generates triplet radical pair, 3((C60N+)n•– + PH•+). The triplet radical pair disappears partly via spin-orbit coupling (SOC)-induced intersystem-crossing process (kSOC) to the ground state. The process (kSOC) is independent of magnetic field. The triplet radical pair decayed to ground state via singlet radical pair, 1((C60N•+)n•– + PH•+). The intersystem-crossing process (kisc2) for the radical pair, but not reverse electron-transfer process (kCR) from the singlet radical pair to the ground state, becomes a rate-determining step for the radical pair to decay via reverse electron-transfer reaction. The intersystem-crossing process (kisc2) is influenced by magnetic field. In the absence of magnetic field, the three sublevels of triplet radical pair are degenerate. In the presence of magnetic field, Zeeman splitting of triplet sublevels occurs. As a consequence of Zeeman splitting, the intersystem-crossing process (kisc2) is retarded in the presence of magnetic field. As a result, the escape process (kesc) from the triplet radical pair increased with the increase of magnetic field and the yield of the escaped PH·+ increased with increase of magnetic field. In the presence of magnetic field, the intersystem-crossing process (kisc2) is controlled by the spin-lattice relaxation from triplet sublevels. Thus the MFEs are explained in terms of spin-lattice relaxation mechanism [10–13].
In the donor-C60 linked compounds such as Ph–C60 [16–18] and ZnP–C60 [19] linked compounds, the decay rate constant of biradical decreases steeply in lower magnetic fields (< 0.1–0.2 T), and then gradually increases and finally becomes almost constant in higher magnetic fields (0.1–0.2 ≤ H ≤ 1 T). The MFEs on the decay of the radical pair between a C60 cluster anion and a pyrene cation have been also reported in a micellar system [29]. In that case, decay rates of the radicals increased with increasing the magnetic field, and MFEs above 2 T were ascribed to the SLR mechanism due to anisotropic Zeeman interaction. The present MFEs are different from those reported previously in the donor-C60 linked compounds or the micellar C60 cluster–pyrene system. Therefore, the MFEs in the present study are most likely ascribed to the nanoclusters of C60N+.
4 Conclusion
A Fullerene derivative containing a positive charge (a monopyrrolidinium salt) (C60N+) formed optically transparent clusters (diameter of ca. 100 nm) in THF/H2O mixed solvent from the results of the absorption spectra and AFM images, and dynamic light scattering. Transient absorption spectra of (C60N+)n–MePH system following laser excitation at 355 nm indicated that the photoinduced intermolecular electron-transfer from triplet excited state of PH to C60N+ cluster (C60N+)n occurred and the yield of the escaped PH•+ increased with increase of the magnetic field. The MFEs are explained by radical pair mechanism and are most likely ascribed to the nanoclusters of (C60N+)n. The present study provides useful information for designing the nanostructures using donor-C60 systems for the development of novel molecular spin systems. Further investigations on the C60N+ cluster-other donor (such as porphyrin) systems and quantitative analysis of the MFEs are now in progress.
Acknowledgments
The authors are grateful to Mr. H. Horiuchi for the preparation of quartz cells for transient absorption spectral experiments. The authors also thank The Center of Advanced Instrumental Analysis, Kyushu University, for 1H-NMR measurements. The present study was financially supported by the grants of the Inamori Foundation and the society for the support of Kyushu University, and by the Grant-in-Aids for Scientific Research: Priority Area of ‘Innovative Utilization of Strong Magnetic Fields’ (Area 767, No. 15085203) and ‘Molecular Nano Dynamics’ (Area 432, No. 17034051), Scientific Research (C) (No. 17550131), and 21 st Century COE Program ‘Function Innovation of Molecular Informatics’ from MEXT of the Japan.