1 Introduction
Over the last years, optical information recording media possessing higher recording density have become very attractive for industry [1]. To meet this goal, an optical disc referred to as a write-once digital versatile disc (DVD-R) has been proposed and successfully introduced on the market. The recording principle is the same for recordable compact discs CD-R and DVD-R, the difference remaining the spot size and the wavelength of the laser light used. CD-R are writable at a wavelength comprised between 770 and 830 nm. More recently, the use of compact high-performance red diode lasers with wavelengths ranging from 600 to 700 nm allowed for DVD-R a six- to eightfold improvement in data packing density in comparison with conventional CDs. However, considering factors such as the recent spread of networks (e.g. Internet) and the emergence of high definition television (HDTV) broadcasting, cheap and convenient recording media, capable of recording image information at even larger capacity, are now required. While DVD-R discs currently serve as high-capacity recording media, demand for larger capacity and higher density has increased. Blu-ray® discs (Blu-ray® disc is a standard developed by Hitachi Ltd., LG Electronics Inc., Matsushita Electric Industrial Co. Ltd., Pioneer Corporation, Royal Philips Electronics, Samsung Electronics Co. Ltd., Sharp Corporation, Sony Corporation, Thomson Multimedia) or HD-DVD discs (a standard developed by Toshiba and NEC) are going to be the next milestone in optical recording technology [2]. By adopting a blue diode laser with a wavelength of 405 nm, the data storage may be increased up to 45 Gigabytes for a 12 cm diameter disc and a 0.6 mm thickness [2]. This high-capacity disc family is going to be the next solution in optical recording technology, turning personal computers into real entertainment devices. Organic dyes have attracted considerable attention in the field of diode laser optical storage for industrial applications. Commercial CD-R and DVD-R contain, as recording layers, numerous dyes based on phthalocyanines [3], hemicyanines [4], cyanines [5] and metallised azo structures [6]. Already present on CD-R and DVD-R as very efficient recording layers, metal complex dyes are adaptable to the upcoming blue laser technology. Basic requirements for such dye media are: (i) strong absorption in the range 350–450 nm (the highest the epsilon, the more efficient is the laser light absorption and the sharper is the pit on the disc), (ii) thermal stability (sharp decomposition above 200 °C), (iii) no melting and flowing of the unrecorded/recorded dye up to the thermal decomposition temperature, (iv) solubility in organic solvents and filterability of their solution allowing the use of a spin coating process to prepare thin films.
Quinonoid molecules possess remarkable chemical and physical properties [7]. The synthesis of unprecedented 6π + 6π electron, potentially antiaromatic quinonoid zwitterions (molecules of type 1) has recently been reported [8–10].
In addition to their fundamental interest [11–16], such molecules appear very promising in different research areas including organic [9,12,17] and supramolecular chemistry [10,17], biochemistry [17], hair colouring [18–20] and coordination chemistry [9,10,21,22]. Here we extend the family of transition metal complexes based on 1 and show that copper compounds can be used as inorganic dyes for potential optical recording application.
2 Experimental section
All solvents were dried and distilled using common techniques unless otherwise stated. 1H NMR (300 MHz) and 13C NMR (75 MHz) spectra were recorded on a Bruker AC-300 instrument. MALDI-TOF mass spectra were recorded on a Biflex III Bruker mass spectrometer. Elemental analyses were performed by the ‘Service de microanalyse du CNRS, université Louis-Pasteur’ (Strasbourg, France). The detailed synthesis and characterising data of compounds 2–6, 8–10, 12 and 13 have been described elsewhere [8–10,21,22].
2.1 General procedure for the synthesis of ligands 7 and 11
Typically, diaminoresorcinol dihydrochloride (0.500 g, 2.35 mmol) was dissolved in water (ca. 10 ml) and then excess of amine (ca. 7 equiv.) was added to the solution. After the reaction mixture was stirred for 2 h, it was extracted with CH2Cl2. The organic layer was collected and dried with MgSO4. After filtration and concentration to 10 ml, Et2O was added to precipitate the product. The product was isolated by filtration.
7: Yield: 70%. Ms (MALDI-TOF+): m/z: 309.2 [M + 1]+; 1H NMR (300 MHz, CDCl3): δ = 1.83 (pent, 3J = 6.3 Hz, 4H, CH2CH2NH), 2.22 (s, 12H, CH3), 2.40 (t, 3J = 6.3 Hz, 4H, CH2N), 3.43 (t, 3J = 6.3 Hz, 4H, CH2NH), 5.18 (s, 1H, NCCH), 5.39 (s, 1H, OCCH), 9.18 (br s, 2H, NH); 13C{1H} NMR (75 MHz, CDCl3) δ = 25.43 (s, CH2CH2NH), 42.45 (s, CH3), 45.31 (s, CH2N), 57.29 (s, CH2NH), 80.59 (s, NCC), 99.11 (s, OCC), 156.77 (s, NC), 172.88 (s, OC); Anal. Calcd. for C16H28N4O2·1.25H2O: C, 58.07; H, 9.29; N, 16.93. Found: C, 58.06; H, 8.97; N, 16.32.
11: Yield: 76%. Ms (MALDI-TOF+): m/z: 393.3 [M + 1]+; 1H NMR (300 MHz, CDCl3): δ = 1.86 (pent, 3J = 6.0 Hz, 4H, CH2CH2CH2), 2.45 (br t, 3J = 4.3 Hz, 8H, NCH2CH2O), 2.51 (t, 3J = 6.0 Hz, 4H, CH2CH2CH2N), 3.44 (t, 3J = 6.1 Hz, 4H, CH2NH), 3.79 (t, 3J = 4.3 Hz, 8H, NCH2CH2O), 5.07 (s, 1H, NCCH), 5.39 (s, 1H, OCCH), 9.50 (br s, 2H, NH); 13C{1H} NMR (75 MHz, CDCl3) δ = 23.58 (s, CH2CH2CH2), 43.30 (s, NCH2CH2O), 53.86 (s, CH2CH2CH2N), 57.41 (s, NHCH2), 66.68 (s, OCH2), 80.41 (s, NCC), 99.12 (s, OCC), 156.86 (s, NC), 172.80 (s, OC); Anal. Calcd. for C20H32N4O4·1/3H2O: C, 60.28; H, 8.26; N, 14.06. Found: C, 60.79; H, 8.36; N, 13.65.
2.2 Synthesis of Cu(II) complexes
Typically, the zwitterionic ligand (ca. 2 mmol) was dissolved in anhydrous dichloromethane (80 ml) and 0.5 equiv. Cu(acac)2 was added to the solution. After the solution was stirred at room temperature for 3 h, the solvent was evaporated and the red, crystalline complex was isolated by filtration after precipitation from a mixture of dichloromethane and pentane.
14: Yield: 86%. (MALDI-TOF+): m/z: 622.3 [M + 1]+; Anal. Calcd. for C28H46CuN8O4·CH2Cl2: C, 49.25; H, 6.84; N, 15.84. Found: C, 49.13; H, 7.05; N, 15.87.
15: Yield: 89%. (MALDI-TOF+): m/z: 678.4 [M + 1]+; Anal. Calcd. for C32H54CuN8O4: C, 56.66; H, 8.02; N, 16.52. Found: C, 56.24; H, 7.98; N, 16.20.
16: Yield: 87%. (MALDI-TOF+): 622.3 [M + 1]+; Anal. Calcd. for C28H46CuN8O4·1/3CH2Cl2: C, 52.31; H, 7.23; N, 17.22. Found: C, 52.34; H, 7.39; N, 17.38.
17: Yield: 85%. (MALDI-TOF+): m/z: 570.2 [M + 1]+; Anal. Calcd. for C24H34CuN4O8: C, 50.56; H, 6.01; N, 9.83. Found: C, 50.16; H, 6.00; N, 9.65.
18: Yield: 90%. (MALDI-TOF+): m/z: 790.3 [M + 1]+; Anal. Calcd. for C36H54CuN8O8: C, 54.70; H, 6.89; N, 14.18. Found: C, 54.38; H, 7.00; N, 14.26.
19: Yield: 85%. (MALDI-TOF+): m/z: 846.5 [M + 1]+; Anal. Calcd. for C40H62CuN8O8: C, 56.75; H, 7.38; N, 13.24. Found: C, 56.90; H, 7.60; N, 12.59.
2.3 Physical measurements
λmax and ε in l/mol cm values of the compounds were determined in CH2Cl2 using an UV spectrophotometer. These values were obtained from measurements performed at three different concentrations.
Decomposition point (DP) and heat release (HR) were determined using a TA Instruments DSC Q100 apparatus, the compound being incorporated into a sealed aluminium pan. The analysis conditions were the following: temperature range from 25 to 400 °C, heating rate 10 °C/min, nitrogen flow of 50 ml/min. Values were determined from a single measurement. The dye melts and decomposes immediately in an irreversible process.
2.4 Test procedure
The following method was used to check the solubility and filterability of dyes for opto-electronic applications. All operations were carried out under a hood and the safety precautions contained in the safety data sheets of the products have to be followed. The temperature has to be kept between 20 and 22 °C during the manipulation, and the solvent was stored in the room at least 24 h before test run begins. The solvent 2,2,3,3-tetrafluoropropanol (TFP) should not contain more than 200 ppm of water; if this is not the case, it must be redistilled until this value is reached.
A sample was prepared from 0.40 g of dye and 20 ml of TFP. It was stirred for 12–15 h on a magnetic plate without heating. The resulting solution was filtered using a 10 ml syringe equipped with a 200 nm pore size PTFE-filter (diameter 13 mm). Filtration Ranking (three categories by filterability): (i) a compound is class A when 10 ml of the solution are filtered with one filter with virtually no stop of smooth filtration (max time allowed for complete filtration is 30 s); (ii) a compound is class B when around 5–6 ml of the solution are filtered with one filter with virtually no stop of smooth filtration and the remaining solution can still be filtered, by applying increased pressure; (iii) a sample is class C when filtration occurs dropwise as the solution passes through the filter.
3 Results and discussion
3.1 Ligand synthesis
The first member of the zwitterionic benzoquinonemonoimine family (molecule 2) was prepared in 2002 by following a multi-step procedure [8]. More recently, we have extended this class of molecules by using a transamination reaction which allows the introduction of additional functionalities, as in 6 and 8–10 [9,10]. Accordingly, the zwitterions [C6H2(NHR)2(O)2] 7 (R = –(CH2)3NMe2) and 11 (R = –(CH2)3N) can now be prepared in high yield by the smooth reaction at room temperature in water and under aerobic conditions between diaminoresorcinol and a large excess of the corresponding primary amines RNH2 (see Section 2).
3.2 Synthesis of the metal complexes
It has been shown that the metallation reaction of ligands with no coordinating arm, such as zwitterion 2, leads to the formation of square planar complexes 3–5 which are almost insoluble (Scheme 1) [21].

In contrast, the metallation of ligand 6, which bears a coordinating amine moiety, affords high yields of the octahedral and soluble complexes 12 [22] and 13 [9] (Scheme 2). Similarly, reactions of ligands 6–11 at room temperature with Cu(acac)2 in a 2:1 ligand/metal ratio leads to the complexes
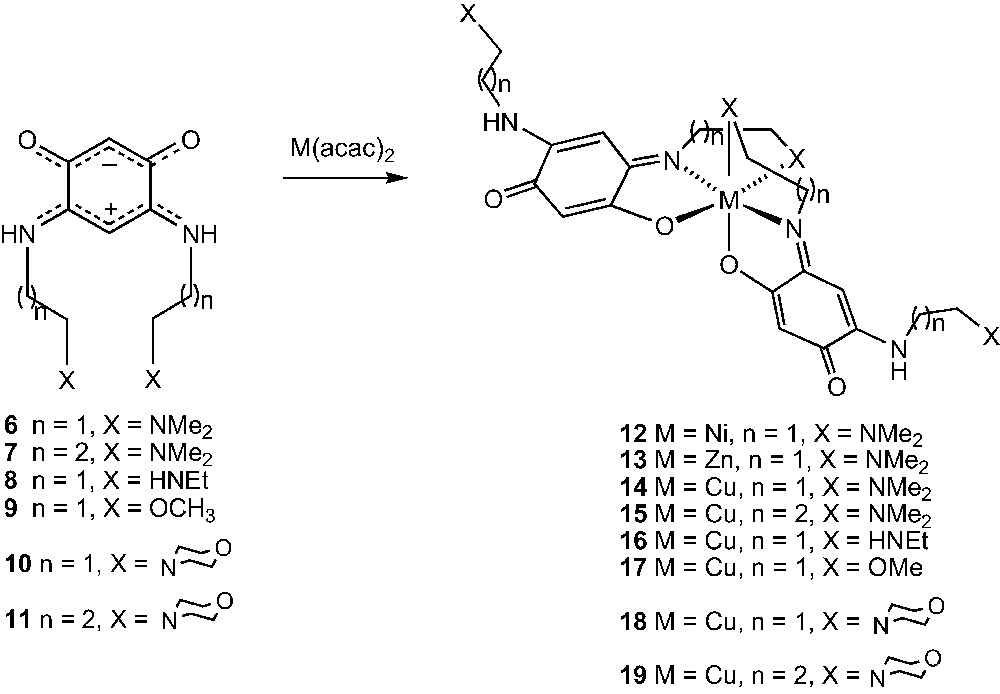
3.3 Physical properties
All the data are listed in Table 1.
Physical properties
Compound | λmax (nm) | ε (l/mol cm) | Decomp. temp. (°C) | HR (w/g) | Filterability |
2 | 340 | 31500 | 213(m) | m* | C |
3 | – | – | – | – | Insoluble |
4 | – | – | – | – | Insoluble |
5 | – | – | – | – | Insoluble |
6 | 348 | 13500 | 191(md) | 0.5 | B |
8 | 350 | 59000 | 167(m) | m | A |
9 | 348 | 29000 | 145(m) | m | A |
12 | 363 | 67000 | 249(m) | m | C |
13 | 335 | 33000 | 210(m) | m | A |
14 | 347 | 55000 | 231(d) | 2 | A |
15 | 351 | 55000 | 207(d) | 1 | A |
16 | 349 | 55000 | 222(d) | 2 | A |
17 | 365 | 54000 | 221(d) | 0.5 | C |
18 | 372 | 60000 | 231(d) | 2.8 | A |
19 | 371 | 69000 | 215(d) | 1.7 | A |
3.3.1 Thermal decomposition
Although the thermal behaviour of a molecule is closely related to its molecular structure (ligand vs. complex, nature of the metal centre and/or the N-substituent), its decomposition is difficult to predict (endothermic vs. exothermic process and/or sharpness of the peaks).
Measurements on the organic dyes 2, 6, 8 and 9 showed a thermal decomposition at lower temperatures than required for optical recording application (> 200 °C). In contrast, the presence of a metal centre such as Zn in complex 13 led to a much better thermal stability than that of its corresponding organic ligand 6 (melting at 210 vs. 191 °C) (Table 1). However, although the temperature was above 200 °C for 13, its decomposition is not exothermic, as required, and occurs with melting (Fig. 1). We also examined the thermal properties of the analogous nickel and copper complexes (12 and 14) to study the influence of the metal centre on thermal stability. Although 12 presents the highest melting temperature (249 °C, see Table 1), only 14 revealed upon decomposition under nitrogen atmosphere an exothermic reaction above 200 °C, which is a key point for this type of application (Fig. 1). Therefore, we decided to focus on the synthesis of copper complexes.

Thermal decomposition of ligand 6 and complexes 12–15.
Consistent with the data for 14, compounds 15–19 revealed also an exothermic thermal decomposition at temperatures above 200 °C, which make them good candidates for optical recording (Table 1). Interestingly, the presence of a longer coordination side arm, as in 15 compared to 14 (one more CH2 group), led to a sharper decomposition which makes it a better candidate, although this decomposition occurs at a lower temperature (207 vs. 231 °C for 15 and 14, respectively) (Fig. 1).
3.3.2 Solubility
Dyes used for recordable compact discs or DVDs are generally applied as solutions by spin-coating on an injected polycarbonate substrate. The preferred solvent for a smooth application (homogeneous groove filling) is 2,2,3,3-tetrafluoropropanol (TFP). When this solvent is evaporated at high rotation speed, only a thin layer of dye remains on the polymer. Non-soluble crystals or solid impurities are usually eliminated by a pre-filtering system on industrial manufacturing lines, causing then the interruption of the production flow. For this reason, a control of solubility as well as of filterability of all the dyes synthesised must be effected. The square planar compounds 3–5 were insoluble in TFP. In contrast, all octahedral complexes (owing to the presence of coordinating arms) were soluble. Inorganic dyes 13–16, 18 and 19, and ligand 8 and 9 revealed the highest solubility and the best filterability (class A, Table 1).
3.3.3 Absorption
Except for 2 and 13, all the complexes and organic dyes showed an absorption maximum very close to the required range (350–450 nm) for potential blue ray optical application (Table 1). Interestingly, the presence of a metal centre does not strongly modify the absorption by comparison with the free ligand.
4 Conclusion
We have prepared novel copper complexes for potential application in optical recording. The following key parameters have been identified: (i) the presence of additional coordinating arms on the quinonoid ligand leads to octahedral complexes with increased solubility, (ii) the presence of Cu(II) as a metal centre leads to a favourable exothermic thermal behaviour, (iii) the presence of quinonoid moieties results in the optical absorption to be in the desired range. A comparison of the data for such dyes revealed that complexes 14–16, 18 and 19 are the best candidates. They are currently under investigations for the preparation of thin films in the blue laser technology.
Acknowledgements
This work was supported by the ‘Centre national de la recherche scientifique’ and the ‘Ministère de la Recherche et des Nouvelles Technologies’ (France). We also thank Clariant for a post-doctoral grant to Dr. Q.-Z. Yang.