1 Introduction
The oligomerization of ethylene represents one of major industrial processes [1]. Linear α-olefin oligomers with relatively short chain lengths are commonly used as comonomers for the preparation of linear low-density polyethylene (LLDPE). The selective dimerization reaction was first achieved in 1954 by Zieger and Martin [2] and later the selectivity was improved by modification of the catalysts and optimization of the reaction conditions [3]. Until now, many efforts are still devoted to the development of highly active and selective ethylene oligomerization catalysts. The independent discovery by Small et al. [4] and Britovsek et al. [5] of highly active bis(imino)pyridyl iron- and cobalt-based catalysts for ethylene polymerization and oligomerization in 1998 has led to high interests in the catalytic chemistry of late-transition metal complexes bearing tridentate ligands [6]. Since that, many studies have been reported about the effects of ligand environment on activity, selectivity and the property of the products [7]. For the tridentate bis(imino)pyridyl ligands, it has generally been found that cobalt-based catalysts are at least an order of magnitude less active than their iron analogues. Furthermore, complexes bearing other tridentate ligands commonly lead to less catalytic activity for ethylene activation [8]. On the other hand, there were also cobalt complexes reported bearing bidentate ligands for ethylene oligomerization, such as dimine [9], 2-(2-pyridyl)quinoxaline [10], β-diketiminate [11], 2-(carbethoxy)-6-iminopyridine [12], 6-(organyl)-2-(imino)pyrine [13]. Again, those catalytic systems showed considerable to good catalytic activity for ethylene reactivity.
We previously reported that cobalt complexes ligated by 2,9-bis(imino)-1,10-phenanthrolines showed much higher activity than their iron analogues [14]. This unusual behavior was different from the bis(imino)pyridine systems. In order to eliminate the coordination effect of the additional imino group on the active center and improve the catalytic properties, 2-imino-1,10-phenanthroline ligands were synthesized and their iron(II) complexes were shown to be highly active catalysts with high selectivity for ethylene oligomerization [15]. Extensive investigations on other late-transition metal complexes and their catalytic properties have also been carried out and nickel analogues showed high activity for ethylene oligomerization [16].
During the course of the preparation of this manuscript, the Solan group reported a series of 2-imino-1,10-phenanthroline cobalt(II) chloride complexes in which the steric bulk of the ortho-aryl substitution pattern has been kept constant and the electronic properties of the para-substituents were systematically varied for aldimine- and ketimine-based ligands [17]. Treatment of these complexes with excess methylaluminoxane (MAO) yields modestly active catalysts for the oligomerization of ethylene (1 atm) which afford mainly linear α-olefins along with some degree of internal olefins. The nature of the para-position substitution pattern has little effect on the activity of the catalyst but does influence internal olefin ratio.
Herein we report our recent studies on a series of cobalt(II) complexes bearing 2-imino-1,10-phenanthroline ligands as active catalytic precursors for ethylene oligomerization. At variance with the work of the Solan group, the steric and electronic properties of the ortho-substituents have been changed systematically. Our work demonstrates the differences on catalytic performance between iron and cobalt complexes as well as the effects of the ligand backbone. Reaction parameters, such as different co-catalysts, Al/Co molar ratio, reaction temperature and ethylene pressure have also been studied and their effects on the catalytic activity will be discussed.
2 Results and discussion
2.1 Synthesis and characterization
All used 2-imino-1,10-phenanthroline ligands were synthesized according to our previous report [15]. The cobalt complexes 1–15 were easily prepared by mixing an ethanol solution of the corresponding ligand and one equivalent of CoCl2 at room temperature (Scheme 1). The resulting precipitate was separated from the reaction solution by filtration, washed with diethylether and dried in vacuum as air-stable powders. All the obtained cobalt complexes were characterized by FT-IR spectra and elemental analysis. In the IR spectra of these complexes, the stretching vibration bands of C=N was shifted to lower wave numbers and the peak intensity was greatly reduced when compared with the free ligands [15], thus demonstrating coordination of the imino nitrogen atom to the cobalt atom. The molecular structures of 2, 3, 8, and 14 were determined by single-crystal X-ray diffraction analysis.
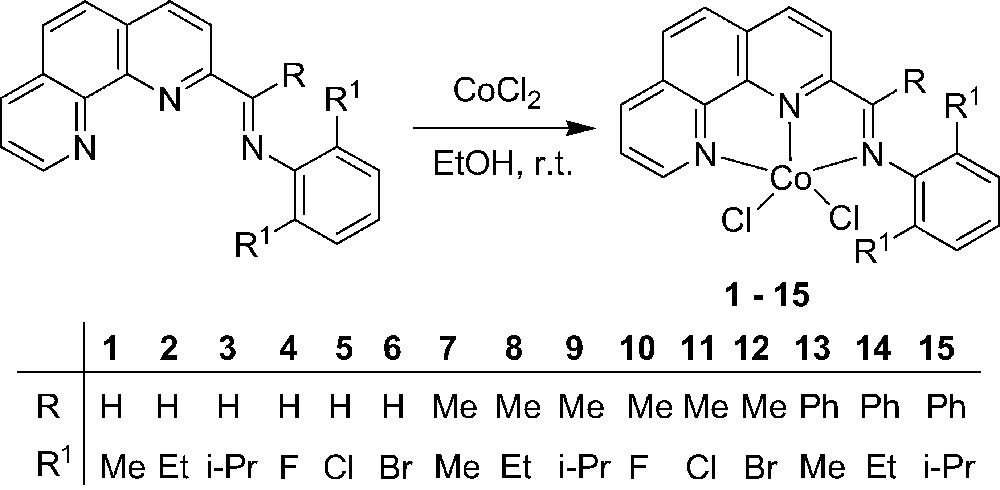
The synthesis of cobalt complexes 1–15.
Single crystals of complexes 2, 3, 8 and 14 suitable for X-ray diffraction analysis were obtained by slow diffusion of diethylether into their methanol solutions. In these four cobalt complexes, the coordination geometry around the cobalt center can be regarded as a distorted trigonal bipyramidal in which the equatorial plane includes one nitrogen atom (next to the imino-C) of phenanthroline and two chlorine atoms. The asymmetric unit of complex 2, however, contains the halves of two independent, nearly identical molecules (Fig. 1) whereas one molecular structure can be found in the asymmetric unit of complex 3, 8, 14 (Figs. 2–5, respectively). Their selected bond lengths and bond angles are listed in Tables 1 and 2.
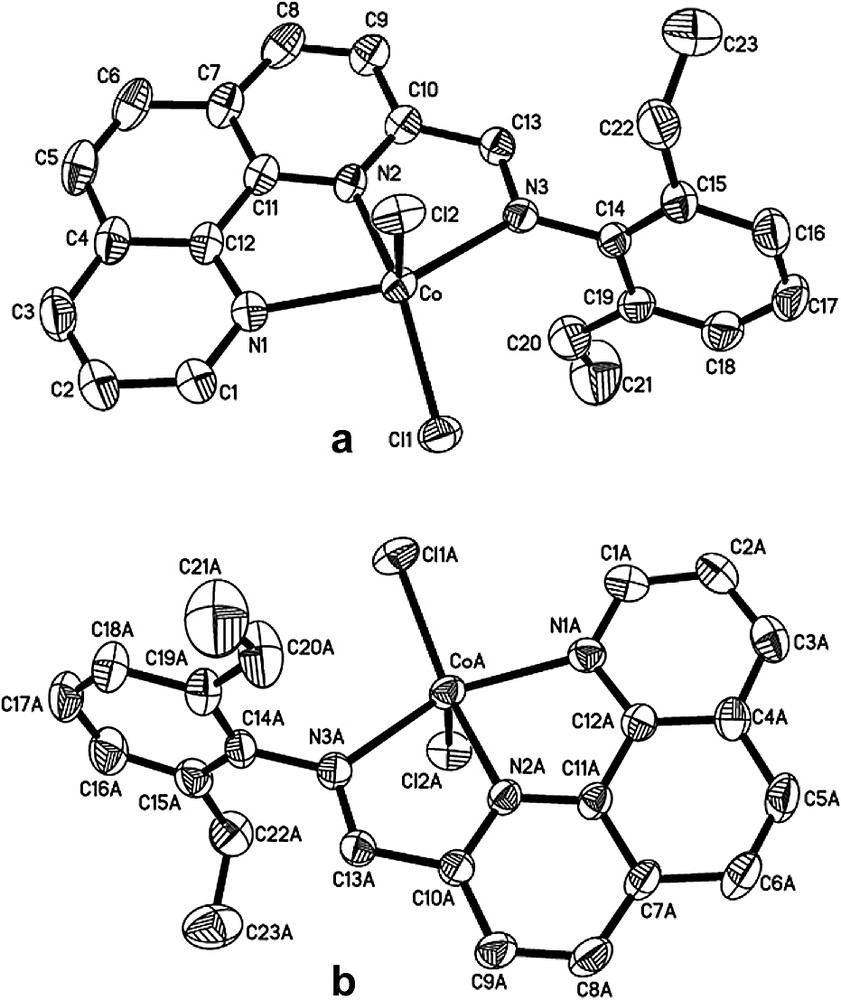
Crystal structure of complex 2 showing the two independent molecules of the asymmetric unit. Displacement ellipsoids are drawn at 30%.
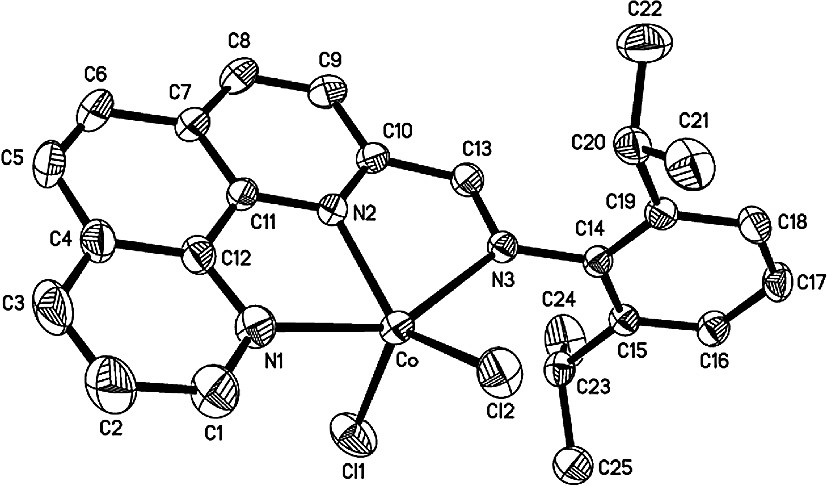
Crystal structure of complex 3 with thermal ellipsoids at 30% probability level and all hydrogen atoms omitted for clarity.

The 1-D chain formed by intermolecular hydrogen bonding interactions (H2A···Cl1) in complex 3.
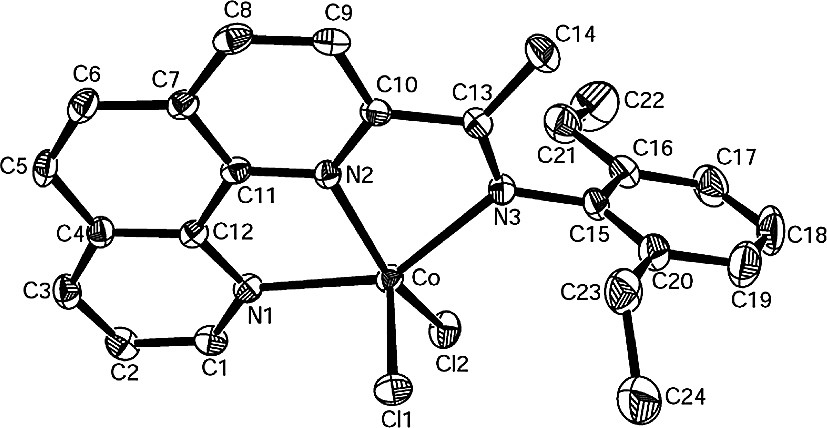
Crystal structure of complex 8 with thermal ellipsoids at 30% probability level and all hydrogen atoms omitted for clarity.
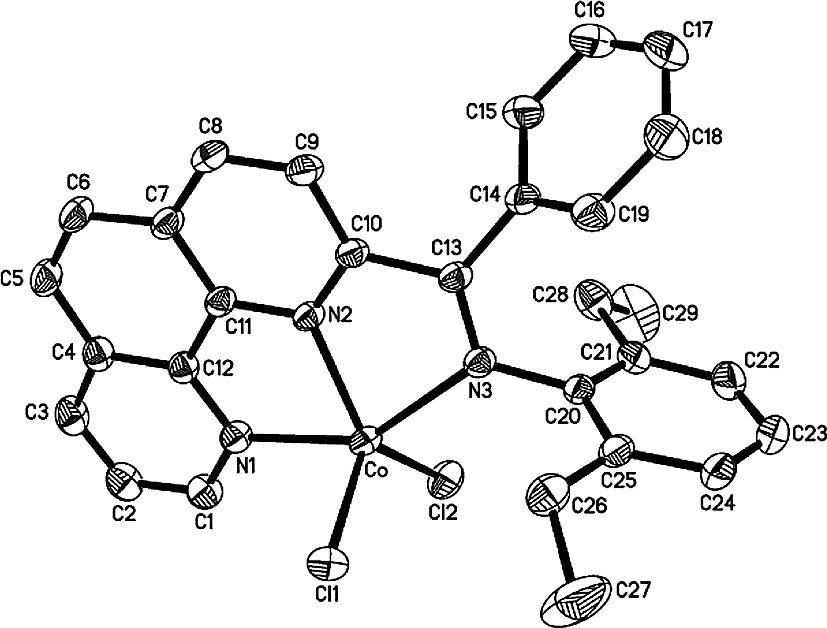
Crystal structure of complex 14 with thermal ellipsoids at 30% probability level and all hydrogen atoms omitted for clarity.
Selected bond lengths (Å) and angles (°) for complex 2
Bond lengths | |||
Co–N(1) | 2.199(2) | CoA–N(1A) | 2.217(2) |
Co–N(2) | 2.035(2) | CoA–N(2A) | 2.039(2) |
Co–N(3) | 2.293(2) | CoA–N(3A) | 2.256(2) |
Co–Cl(1) | 2.251(1) | CoA–Cl(1A) | 2.245(1) |
Co–Cl(2) | 2.270(1) | CoA–Cl(2A) | 2.292(1) |
N(3)–C(13) | 1.277(3) | N(3A)–C(13A) | 1.273(3) |
Bond angles | |||
N(2)–Co–N(1) | 76.58(9) | N(2A)–CoA–N(1A) | 75.47(8) |
N(2)–Co–N(3) | 74.03(8) | N(2A)–CoA–N(3A) | 73.80(8) |
N(1)–Co–N(3) | 148.87(9) | N(1A)–CoA–N(3A) | 145.26(8) |
N(1)–Co–Cl(1) | 95.17(6) | N(1A)–CoA–Cl(1A) | 95.67(6) |
N(2)–Co–Cl(1) | 143.28(6) | N(2A)–CoA–Cl(1A) | 149.17(6) |
N(3)–Co–Cl(1) | 102.22(5) | N(3A)–CoA–Cl(1A) | 102.44(6) |
N(1)–Co–Cl(2) | 94.96(7) | N(1A)–CoA–Cl(2A) | 100.49(6) |
N(2)–Co–Cl(2) | 100.58(6) | N(2A)–CoA–Cl(2A) | 96.19(6) |
N(3)–Co–Cl(2) | 100.24(5) | N(3A)–CoA–Cl(2A) | 98.56(6) |
Cl(1)–Co–Cl(2) | 115.88(3) | Cl(1A)–CoA–Cl(2A) | 114.55(3) |
Selected bond lengths (Å) and angles (°) for complexes 3, 8 and 14
3 | 8 | 14 | |
Bond lengths | |||
Co–N(1) | 2.225(2) | 2.244(2) | 2.223(1) |
Co–N(2) | 2.047(2) | 2.055(2) | 2.053(1) |
Co–N(3) | 2.310(2) | 2.254(2) | 2.316(1) |
Co–Cl(1) | 2.255(1) | 2.264(1) | 2.259(1) |
Co–Cl(2) | 2.233(1) | 2.282(1) | 2.255(1) |
N(3)–C(13) | 1.267(3) | 1.284(3) | 1.281(3) |
Bond angles | |||
N(2)–Co–N(1) | 75.91(7) | 75.42(8) | 76.27(7) |
N(2)–Co–N(3) | 73.47(6) | 73.61(8) | 72.88(7) |
N(1)–Co–N(3) | 147.79(7) | 146.64(7) | 148.62(7) |
N(1)–Co–Cl(1) | 94.77(5) | 98.96(6) | 96.37(6) |
N(2)–Co–Cl(1) | 104.54(5) | 99.64(7) | 112.88(6) |
N(3)–Co–Cl(1) | 102.29(5) | 98.12(6) | 100.69(6) |
N(1)–Co–Cl(2) | 99.06(6) | 97.73(6) | 96.55(6) |
N(2)–Co–Cl(2) | 137.24(5) | 149.72(6) | 132.63(6) |
N(3)–Co–Cl(2) | 96.63(5) | 102.73(6) | 100.02(5) |
Cl(1)–Co–Cl(2) | 118.22(3) | 110.60(3) | 114.44(3) |
In the structure of complex 2, the two independent molecules give slightly different bond lengths and bond angles (Table 1). In molecule (a), the cobalt center slightly deviates by 0.062 Å from the triangular plane formed by N2, Cl1 and Cl2, while this deviation is 0.035 Å in molecule (b). Furthermore, differences are observed for the dihedral angles between the equatorial plane and the phenanthroline plane, the phenyl ring attached to the imino-N and the phenanthroline plane (69.9° and 83.7° in (a), however, 94.9° and 74.4° in (b), respectively). In both molecules, the bond angles subtended by the axial Co–N bonds are 148.87(9)° (N(1)–Co–N(3)) and 145.26(8)° (N(1A)–CoA–N(3A)), respectively. The Co–N distance in the equatorial plane and the imino C=N distance are nearly identical. In each molecule, the Co–N bond in the equatorial plane is shorter than that of the two axial Co–N bonds and the two Co–Cl bond distances show a slight difference, which is very similar to the situation in the iron(II) analogues [15].
Unlike complex 2, one complex molecule is found in the asymmetric unit of complex 3 (Fig. 2). The cobalt atom is almost coplanar with the equatorial plane composed by N2, Cl1 and Cl2 with the deviation of 0.005 Å. The equatorial angles are 104.54(5)°, 137.24(5)° and 118.22(3)°, respectively, and the axial Co–N bonds form a N(1)–Co–N(3) angle of 147.79(7)°. The dihedral angle between the equatorial plane and the phenanthroline plane is 73.0°, largely deviating from 90°. The phenyl ring on the imino-C is nearly perpendicular to the phenanthroline plane with a dihedral angle of 91.5°. The Co–N(2) bond (2.047(2) Å) on the equatorial plane is obviously shorter than the axial Co–N(1) (2.225(2) Å) and Co–N(3) (2.310(2) Å) bonds. The two Co–Cl bond lengths show a slight difference: Co–Cl(1) = 2.2546(7) Å and Co–Cl(2) = 2.233(1) Å. The imino N(3)–C(13) bond length of 1.267(3) Å, which indicates a typical C=N double-bond character, is relatively shorter than that in other cobalt analogues, which is probably ascribed to the less bulky hydrogen atom on the imino-C and the bulkier isopropyl groups at the ortho-position of the phenyl ring on the imino-N.
In the solid state, complex 3 displays intermolecular hydrogen bonding interactions between one chlorine atom (Cl1) and one hydrogen atom (H(2A)) of the phenanthroline from another molecule. Hydrogen bonding between two neighboring molecules results in a 1-D infinite zigzag chain, as shown in Fig. 3. The hydrogen bond angle of C2—H2A···Cl1 is 144.87° and the distances of H2A···Cl1 and C(2)···Cl1 are 2.757 and 3.558 Å, respectively.
In complex 8 (Fig. 4), one nitrogen atom (N2) of the phenanthroline and two chlorine atoms form the equatorial plane, and the cobalt atom deviates slightly by 0.023 Å from this plane. The three equatorial angles N(2)–Co–Cl(1), N(2)–Co–Cl(2) and Cl(1)–Co–Cl(2) are, respectively, 99.64(7)°, 149.72(6)° and 110.60(3)° whereas the axial Co–N bonds form an angle of 146.64(7)°. The equatorial plane is almost perpendicular to the phenanthroline plane with a dihedral angle of 91.8° and the phenyl ring on the imino-N and the phenanthroline plane form a dihedral angle of 77.0°. The two axial Co–N bond lengths, 2.244(2) and 2.254(2) Å, are longer by about 0.19 Å than that in the equatorial plane (Co–N(2) = 2.055(2) Å). The imino N(3)–C(13) bond length of 1.284(3) Å indicates a typical C=N double bond character, but is slightly longer than that in the corresponding aldimine complex 2, which is probably due to the relatively bulky methyl on the imino-C.
In the structure of 14 (Fig. 5), the cobalt atom slightly deviates by 0.0257 Å from the triangular plane of N2, Cl1 and Cl2 with equatorial angle ranging from 112.88(6)° and 132.63(6)°. This equatorial plane is nearly perpendicular to the phenanthroline plane with a dihedral angle of 87.6°. The dihedral angles between the two phenyl rings, the phenyl ring on the imino-N and the phenanthroline plane are 82.9° and 93.6°, respectively. The Co–N(2) bond (2.053(2) Å) is much shorter than the axial bonds Co–N(1) (2.223(2) Å) and Co–N(3)(imino) (2.316(2) Å). The imino N(3)–C(13) bond length of 1.281(3) Å is similar to that in the corresponding methyl-ketimine complex 8, but slightly longer than in the aldimine complex 2.
2.2 Ethylene oligomerization
Various aluminum-based co-catalysts were used for exploring the catalytic activity of the cobalt complexes. When diethylaluminum chloride or ethylaluminum dichloride was used as a co-catalyst, only low catalytic activity (less than 104 g mol–1 h–1) was observed. Considerable to good activities were obtained with MAO, modified methylaluminoxane (MMAO) and triisobutylaluminum (Al(i-Bu)3) as co-catalysts. Complex 15 was typically investigated under a range of reaction conditions, such as different co-catalysts, molar ratio of co-catalyst to cobalt and reaction temperature at 1 atm of ethylene. The best catalytic activity (more than 105 g mol–1 h–1) was observed upon activation with MMAO which was therefore used extensively. Butenes predominate among the oligomers produced. The results for ethylene oligomerization are summarized in Table 3.
The results of ethylene oligomerization by complexes 1–15 at 1 atm of ethylenea
Entry | Complex | Co-cat | Al/Co | Tb (°C) | Activityc | Oligomers distributiond (%) | |
C4 | C6 | ||||||
1 | 15 | Al(i-Bu)3 | 500 | 20 | 0.652 | 100 | – |
2 | 15 | MAO | 250 | 20 | 0.822 | 95.4 | 4.6 |
3 | 15 | MAO | 500 | 20 | 1.19 | 95.3 | 4.7 |
4 | 15 | MAO | 1000 | 20 | 0.962 | 93.7 | 6.3 |
5 | 15 | MMAO | 100 | 20 | 1.17 | 95.5 | 4.5 |
6 | 15 | MMAO | 250 | 20 | 2.28 | 95.3 | 4.7 |
7 | 15 | MMAO | 500 | 20 | 3.53 | 94.5 | 5.5 |
8 | 15 | MMAO | 1000 | 20 | 7.00 | 94.2 | 5.8 |
9 | 15 | MMAO | 1500 | 20 | 4.15 | 95.2 | 4.8 |
10 | 15 | MMAO | 2000 | 20 | 4.05 | 92.9 | 5.1 |
11 | 15 | MMAO | 2500 | 20 | 3.98 | 93.7 | 6.3 |
12 | 15 | MMAO | 1000 | 0 | 3.14 | 95.9 | 4.1 |
13 | 15 | MMAO | 1000 | 40 | 4.03 | 97.2 | 2.8 |
14 | 15 | MMAO | 1000 | 60 | 1.21 | 100 | – |
15 | 1 | MMAO | 1000 | 20 | 1.58 | 100 | – |
16 | 2 | MMAO | 1000 | 20 | 1.93 | 99.5 | 0.5 |
17 | 3 | MMAO | 1000 | 20 | 2.65 | 100 | – |
18 | 4 | MMAO | 1000 | 20 | 1.46 | 100 | – |
19 | 5 | MMAO | 1000 | 20 | 2.95 | 100 | – |
20 | 6 | MMAO | 1000 | 20 | 3.23 | 99.4 | 0.6 |
21 | 7 | MMAO | 1000 | 20 | 1.93 | 100 | – |
22 | 8 | MMAO | 1000 | 20 | 2.34 | 97.5 | 2.5 |
23 | 9 | MMAO | 1000 | 20 | 2.57 | 99.3 | 0.7 |
24 | 10 | MMAO | 1000 | 20 | 1.66 | 100 | – |
25 | 11 | MMAO | 1000 | 20 | 2.16 | 99.2 | 0.8 |
26 | 12 | MMAO | 1000 | 20 | 4.18 | 97.0 | 3.0 |
27 | 13 | MMAO | 1000 | 20 | 3.08 | 97.3 | 2.7 |
28 | 14 | MMAO | 1000 | 20 | 3.60 | 98.0 | 2.0 |
a General conditions: complex: 5 μmol; solvent: toluene (30 ml); reaction time: 30 min.
b Reaction temperature.
c The unit of activity: 105 g mol–1 h–1.
d Determined by GC.
2.2.1 Effects of the molar ratio of Al/Co and reaction temperature
Upon activation with MAO, complex 15 showed the highest catalytic activity at the Al/Co molar ratio of 500. However, when MMAO was employed as a co-catalyst and the Al/Co molar ratio was changed from 100 to 2500, the catalytic activity of 15 firstly increased and then decreased and displayed the maximum at the Al/Co molar ratio of 1000. Between 1500 and 2500 almost no change in activity could be observed. The variation of the Al/Co molar ratio had no large influence on the distribution of oligomers.
With a fixed Al/Co molar ratio of 1000 using MMAO, the reaction temperature largely affected the catalytic activity. The highest activity was obtained at 20 °C (entry 8, Table 3). Higher reaction temperatures led to a large decrease in the catalytic activity (entry 14, Table 3), most likely due to the deactivation of some active centers and the lower solubility of ethylene in toluene.
2.2.2 Effect of the ligands environment
To compare the effect of the ligand environment on the catalytic activity, all cobalt complexes 1–15 were investigated under the same conditions (Al/Co molar ratio of 1000 and 20 °C). The 2,6-diisopropyl-substituted phenyl-ketimine complex 15 showed the highest catalytic activity (entry 8, Table 3).
Variation of the R substituent on the imino-C resulted in a change of the catalytic activity. For the complexes bearing the same alkyl groups at the ortho-positions of the imino-N aryl ring, the catalytic activities increased in turn according to aldimine (R = H), methyl-ketimine (R = Me) and phenyl-ketimine (R = Ph) complexes (Ph > Me > H). This trend was found to be different from the related iron systems [15]. Furthermore aldimine as well as ketimine complexes bearing bulky substituents on the ortho-position of the imino-N aryl ring displayed the highest catalytic activities (isopropyl > ethyl > methyl groups). For the cobalt complexes bearing 2,6-dihalogen-substituted ligands, the bulkiness and electronegativity of the halogen atoms had large influence on the catalytic activity. For both aldimine and methyl-ketimine complexes with the bulky and moderately electronegative bromine atoms at the ortho-position of the imino-N aryl ring, the catalytic activities were found to be highest (bromo- > chloro- > fluoro-, entry 18–20 and 24–26 in Table 3). Except for the 2,6-dichloro-substituted complexes, methyl-ketimine complexes showed higher catalytic activity than the corresponding aldimine complexes. Cobalt complexes bearing electron-withdrawing halogen groups displayed comparable catalytic activities and produced similar oligomeric products as the complexes bearing electron-donating alkyl groups. The substituents' effect was not so clearly observed in the recent paper by Pelletier et al. [17], possibly because of the fixed bulky ortho-isopropyl group and a weaker influence of the para-substituents of the imino-N aryl ring.
2.2.3 Effect of ethylene pressure
Firstly the catalytic performances of complex 15 were investigated using MMAO or MAO as co-catalyst at 10 atm of ethylene. The catalytic activity increased more than three times under similar conditions when MMAO was used as co-catalyst, but a higher pressure seems to have almost no influence on the oligomeric products formed. However, when the catalyst precursor was activated with MAO, the consumption of ethylene was observed to last for a longer time, consistent with a longer lifetime of the active species. The catalytic activity increased about 10 times, up to 1.46 × 106 g mol–1 h–1 in 60 min, compared with the result at 1 atm after 30 min (entry 2 in Table 4 and entry 3 in Table 3). More importantly, the amount of C6 products among the oligomers increased up to 35 wt.%. Therefore complexes 1–6 and 13–14 were also investigated in the presence of MAO and the results are summarized in Table 4. Except for the 2,6-difluoro-substituted aldimine complex 4, these complexes showed higher catalytic activities under 10 atm of ethylene, but they are about one order of magnitude less active than their corresponding iron analogues under similar conditions [15]. Furthermore at 10 atm for the 2,6-dialkyl-substituted aldimine complexes 1–3 and phenyl-ketimine complexes 13–15, the same activity pattern was observed at 1 atm of ethylene pressure. The complexes with bulky isopropyl groups showed higher activities than those with less bulky methyl groups. Except for complex 4 with less bulky and high electronegative fluorine atoms, complexes 5 and 6 containing halogen groups also displayed higher catalytic activity (more than 106 g mol–1 h–1) at 10 atm of ethylene pressure.
The results of ethylene oligomerization by complexes 1–6 and 13–15 at 10 atm of ethylene a
Entry | Complex | Activityb | Oligomers distributionc (%) | ||
C4 | C6 | C8 | |||
1d | 15 | 23.4 | 93.4 | 6.6 | – |
2 | 15 | 14.6 | 64.2 | 35.0 | 0.8 |
3 | 1 | 6.70 | 97.7 | 2.0 | 0.3 |
4 | 2 | 12.0 | 97.6 | 1.6 | 0.8 |
5 | 3 | 12.6 | 94.1 | 5.2 | 0.7 |
6 | 4 | 1.26 | 97.6 | 2.4 | – |
7 | 5 | 11.8 | 98.6 | 1.4 | – |
8 | 6 | 10.2 | 96.8 | 3.2 | – |
9 | 13 | 9.95 | 98.1 | 1.9 | – |
10 | 14 | 10.4 | 97.0 | 3.0 | – |
a General conditions: complex: 5 μmol; solvent: toluene (100 ml); co-cat: MAO; molar ratio of Al/Co: 500; reaction temperature: 40 °C; reaction time: 60 min.
b The unit of activity: 105 g mol–1 h–1.
c Determined by GC.
d co-cat: MMAO; molar ratio of Al/Co: 1000; reaction temperature: 20 °C; reaction time: 30 min.
3 Conclusion
A series of tridentate cobalt(II) complexes bearing 2-imino-1,10-phenanthrolinyl ligands have been synthesized and fully characterized. Upon treatment with MAO or MMAO, these cobalt(II) complexes showed moderate to high catalytic activities of up to 2.34 × 106 g mol–1 (Co) h–1 for ethylene oligomerization at 10 atm ethylene. Butenes were found to be predominant among the oligomers produced. MMAO was found to be a more effective co-catalyst when the reactions were carried out at 1 atm ethylene. By comparison with the data obtained with MAO as co-catalyst, the lifetime of the catalysts was prolonged at elevated ethylene pressure along with an improved catalytic activity. For the complexes bearing the same alkyl groups at the ortho-positions of the imino-N aryl ring, the catalytic activities increased in the sequence: aldimine (R = H), methyl-ketimine (R = Me) and phenyl-ketimine (R = Ph). For both aldimine and methyl-ketimine complexes, bulky and moderately electronegative atoms at the ortho-positions of the imino-N aryl ring resulted in the sequence of catalytic activities: bromo- > chloro- > fluoro.
4 Experimental section
4.1 General considerations
All manipulations for air or moisture-sensitive compounds were carried out under an atmosphere of nitrogen using standard Schlenk techniques. IR spectra were recorded on a Perkin Elmer FT-IR 2000 spectrometer using KBr disc in the range of 4000–400 cm–1. Elemental analyses were performed on a Flash EA1112 microanalyzer. GC analyses were performed with a Carlo Erba gas chromatograph equipped with a flame ionization detector and a 30 m (0.25 mm i.d., 0.25 μm film thickness) DM-1 silica capillary column. The yield of oligomers was calculated by referencing with the mass of the solvent on the basis of the prerequisite that the mass of each fraction is approximately proportional to its integrated areas in the GC traces.
Toluene was refluxed over sodium/benzophenone and distilled under nitrogen prior to use. Diethylaluminum chloride and ethylaluminum dichloride were purchased from Acros Chemicals. Triisobutylaluminum (Al(i-Bu)3), MAO (1.46 M in toluene) and MMAO-3A (7% aluminum in heptane solution) were purchased from Akzo Nobel Chemical Inc. All other chemicals were obtained commercially and used without further purification unless otherwise stated.
4.2 Synthesis of the complexes 1–15
4.2.1 General procedure
A solution of anhydrous CoCl2 in absolute ethanol was added dropwise to a solution of the ligand in absolute ethanol. Then the reaction mixture was stirred at room temperature for 9 h. The resulting precipitate was filtered, washed with diethyl ether and dried in vacuum. All complexes were prepared in high yield in this manner.
4.2.2 2-Formyl-1,10-phenanthroline(2,6-dimethylanil)CoCl2 (1)
Isolated as a brownish yellow powder in 82% yield. FT-IR (KBr disc, cm–1): 3437, 3063, 2953, 1612, 1513, 1495, 1472, 1437, 1407, 1297, 1183, 1142, 1117, 1094, 963, 861, 780, 738, 645. Anal. Calc. for C21H17Cl2CoN3·0.5EtOH (464.26): C, 56.92; H, 4.34; N, 9.05. Found: C, 56.53; H, 4.05; N, 8.84.
4.2.3 2-Formyl-1,10-phenanthroline(2,6-diethylanil)CoCl2 (2)
Isolated as a brown powder in 52% yield. FT-IR (KBr disc, cm–1): 3444, 3060, 2967, 2934, 2875, 1610, 1585, 1514, 1495, 1451, 1407, 1297, 1176, 1143, 1117, 963, 865, 762, 739, 645. Anal. Calc. for C23H21Cl2CoN3 (469.27): C, 58.87; H, 4.51; N, 8.95. Found: C, 58.64; H, 4.47; N, 8.78.
4.2.4 2-Formyl-1,10-phenanthroline(2,6-diisopropylanil)CoCl2 (3)
Isolated as a brownish yellow powder in 93% yield. FT-IR (KBr disc, cm–1): 3437, 3059, 2965, 2929, 2869, 1609, 1515, 1495, 1463, 1409, 1297, 1176, 1144, 1117, 961, 851, 806, 765, 737, 645. Anal. Calc. for C25H25Cl2CoN3 (497.33): C, 60.38; H, 5.07; N, 8.45. Found: C, 60.12; H, 5.24; N, 8.34.
4.2.5 2-Formyl-1,10-phenanthroline(2,6-difluoroanil)CoCl2 (4)
Isolated as a brownish yellow powder in 49% yield. FT-IR (KBr disc, cm–1): 3418, 3059, 3021, 1613, 1587, 1514, 1470, 1435, 1407, 1284, 1236, 1198, 1143, 1117, 1016, 965, 863, 787, 737. Anal. Calc. for C19H11Cl2CoF2N3 (449.15): C, 50.81; H, 2.47; N, 9.36. Found: C, 50.60; H, 2.48; N, 9.18.
4.2.6 2-Formyl-1,10-phenanthroline(2,6-dichloroanil)CoCl2 (5)
Isolated as a yellowish green powder in 65% yield. FT-IR (KBr disc, cm–1): 3398, 3062, 3012, 1613, 1581, 1564, 1512, 1494, 1440, 1407, 1359, 1341, 1296, 1234, 1199, 1117, 1143, 963, 865, 788, 739, 644. Anal. Calc. for C19H11Cl4CoN3 (482.06): C, 47.34; H, 2.30; N, 8.72. Found: C, 47.35; H, 2.60; N, 8.64.
4.2.7 2-Formyl-1,10-phenanthroline(2,6-dibromoanil)CoCl2 (6)
Isolated as a bluish green powder in 62% yield. FT-IR (KBr disc, cm–1): 3420, 3064, 1642, 1585, 1572, 1549, 1512, 1496, 1459, 1429, 1397, 1212, 1198, 1143, 867, 777, 742, 728, 641. Anal. Calc. for C19H11Br2Cl2CoN3 (570.96): C, 39.97; H, 1.94; N, 7.36. Found: C, 40.12; H, 2.15; N, 7.51.
4.2.8 2-Acetyl-1,10-phenanthroline(2,6-dimethylanil)CoCl2 (7)
Isolated as a brownish yellow powder in 86% yield. FT-IR (KBr disc, cm–1): 3435, 3049, 2978, 1612, 1583, 1511, 1492, 1467, 1406, 1373, 1287, 1211, 1152, 1093, 864, 790, 772, 743. Anal. Calc. for C22H19Cl2CoN3 (455.25): C, 58.04; H, 4.21; N, 9.23. Found: C, 58.02; H, 4.31; N, 8.95.
4.2.9 2-Acetyl-1,10-phenanthroline(2,6-diethylanil)CoCl2 (8)
Isolated as a brownish yellow powder in 58% yield. FT-IR (KBr disc, cm–1): 3448, 2964, 2876, 1611, 1584, 1512, 1493, 1449, 1407, 1373, 1286, 1197, 1154, 873, 836, 785, 741, 657. Anal. Calc. for C24H23Cl2CoN3 (483.30): C, 59.64; H, 4.80; N, 8.69. Found: C, 59.69; H, 4.86; N, 8.62.
4.2.10 2-Acetyl-1,10-phenanthroline(2,6-diisopropylanil)CoCl2 (9)
Isolated as a brownish yellow powder in 76% yield. FT-IR (KBr disc, cm–1): 3435, 2967, 2869, 1609, 1511, 1464, 1410, 1373, 1287, 1196, 1146, 850, 789, 654. Anal. Calc. for C26H27Cl2CoN3 (511.35): C, 61.07; H, 5.32; N, 8.22. Found: C, 60.86; H, 5.49; N, 7.83.
4.2.11 2-Acetyl-1,10-phenanthroline(2,6-difluoroanil)CoCl2 (10)
Isolated as a brownish yellow powder in 85% yield. FT-IR (KBr disc, cm–1): 3420, 3056, 1614, 1587, 1515, 1472, 1407, 1373, 1282, 1227, 1151, 1027, 1005, 862, 775, 744. Anal. Calc. for C20H13Cl2CoF2N3 (463.19): C, 51.86; H, 2.83; N, 9.07. Found: C, 51.55; H, 3.01; N, 8.78.
4.2.12 2-Acetyl-1,10-phenanthroline(2,6-dichloroanil)CoCl2 (11)
Isolated as a greenish yellow powder in 81% yield. FT-IR (KBr disc, cm–1): 3446, 3060, 2973, 2867, 1616, 1560, 1515, 1493, 1438, 1406, 1375, 1284, 1233, 1064, 862, 788, 744, 658. Anal. Calc. for C20H13Cl4CoN3 (496.08): C, 48.42; H, 2.64; N, 8.47. Found: C, 48.29; H, 2.78; N, 8.26.
4.2.13 2-Acetyl-1,10-phenanthroline(2,6-dibromoanil)CoCl2 (12)
Isolated as a greenish yellow powder in 86% yield. FT-IR (KBr disc, cm–1): 3446, 3055, 2968, 2866, 1616, 1579, 1549, 1515, 1492, 1432, 1406, 1374, 1285, 1230, 1149, 1063, 862, 832, 787, 743, 727, 658. Anal. Calc. for C20H13Br2Cl2CoN3 (584.98): C, 41.06; H, 2.24; N, 7.18. Found: C, 40.77; H, 2.25; N, 7.10.
4.2.14 2-Benzoyl-1,10-phenanthroline(2,6-dimethylanil)CoCl2 (13)
Isolated as a brown powder in 71% yield. FT-IR (KBr disc, cm–1): 3442, 3060, 2908, 1603, 1565, 1510, 1492, 1440, 1403, 1295, 1217, 1094, 998, 866, 789, 701. Anal. Calc. for C27H21Cl2CoN3 (517.31): C, 62.69; H, 4.09; N, 8.12. Found: C, 62.43; H, 4.08; N, 8.06.
4.2.15 2-Benzoyl-1,10-phenanthroline(2,6-diethylanil)CoCl2 (14)
Isolated as a greenish yellow powder in 69% yield. FT-IR (KBr disc, cm–1): 3443, 3063, 2971, 2933, 1608, 1586, 1557, 1507, 1491, 1444, 1404, 1326, 1282, 1211, 1147, 1109, 999, 882, 836, 782, 703. Anal. Calc. for C29H25Cl2CoN3 (545.37): C, 63.87; H, 4.62; N, 7.70. Found: C, 63.71; H, 4.42; N, 7.51.
4.2.16 2-Benzoyl-1,10-phenanthroline(2,6-diisopropylanil)CoCl2 (15)
Isolated as a greenish yellow powder in 83% yield. FT-IR (KBr disc, cm–1): 3060, 2964, 2867, 1606, 1583, 1510, 1491, 1438, 1404, 1291, 1270, 1209, 997, 864, 782, 701. Anal. Calc. for C31H29Cl2CoN3 (573.42): C, 64.93; H, 5.10; N, 7.33. Found: C, 64.78; H, 5.29; N, 7.22.
4.3 General procedure for ethylene oligomerization
4.3.1 Ethylene oligomerization at 1 atm of ethylene pressure
A flame dried three-neck round flask was loaded with the complex and vacuated-filled three times with nitrogen. Then ethylene was charged together with freshly distilled toluene and stirring was maintained for 10 min. A certain amount of co-catalyst was injected via a syringe. The reaction mixture was stirred for 30 min and then terminated with 5% aqueous hydrogen chloride. The content and distribution of oligomers were determined by GC.
4.3.2 Ethylene oligomerization at 10 atm of ethylene pressure
A 250-ml autoclave stainless steel reactor equipped with a mechanical stirrer and a temperature controller was heated in vacuum for at least 2 h over 80 °C, allowed to cool to the required reaction temperature under ethylene atmosphere, and then charged with toluene, the desired amount of co-catalyst and a toluene solution of the catalytic precursor. The total volume was 100 ml. Reaching the reaction temperature, the reactor was sealed and pressurized to 10 atm of ethylene pressure. The ethylene pressure was kept constant during the reaction time by feeding the reactor with ethylene. To determine the composition and mass distribution of the oligomers obtained, using gas chromatography, a small amount of the reaction solution was collected after the reaction was carried out for the required time and quenched by addition of 5% aqueous hydrogen chloride. Then the residual reaction solution was quenched with 5% hydrochloric acid ethanol.
4.4 X-ray crystallography measurements
Single-crystal X-ray diffraction studies for 2 and 3 were carried out on a Bruker P4 diffractometer with graphite monochromated Mo–K radiation (λ = 0.71073 Å) at 293(2) K. Intensity data for crystals of 8 and 14 were collected with a Rigaku RAXIS Rapid IP diffractometer with graphite monochromated Mo-K radiation (λ = 0.71073 Å) at 293(2) K. Cell parameters were obtained by global refinement of the positions of all collected reflections. Intensities were corrected for Lorentz and polarization effects and empirical absorption. The structures were solved by direct methods and refined by full-matrix least-squares on F2. All non-hydrogen atoms were refined anisotropically. All hydrogen atoms were placed in calculated positions. Structure solution and refinement were performed by using the SHELXL-97 Package [18]. Crystallographic data and processing parameters for complexes 2, 3, 8 and 14 are summarized in Table 5. The data of complexes 2, 3, 8 and 14 have been deposited with the Cambridge Crystallographic Data Center under CCDC 621104, 621105, 621106 and 621107, respectively.
Crystal data and structure refinement for complexes 2, 3, 8 and 14
Data | 2 | 3 | 8 | 14 |
Formula | 2 C23H21Cl2CoN3 | C25H25Cl2CoN3 | C24H23Cl2CoN3 | C29H25Cl2CoN3 |
Formula weight | 938.52 | 497.31 | 483.28 | 545.35 |
Temperature (K) | 293(2) | 293(2) | 293(2) | 293(2) |
Wavelength (Å) | 0.71073 | 0.71073 | 0.71073 | 0.71073 |
Crystal system | Monoclinic | Orthorhombic | Triclinic | Triclinic |
Space group | P2(1)/n | Pbca | ||
a (Å) | 9.4674(3) | 14.9445(7) | 8.1365(16) | 8.7410(17) |
b (Å) | 18.6424(6) | 16.0133(8) | 9.0670(18) | 8.7867(18) |
c (Å) | 24.9135(8) | 19.4261(10) | 16.194(3) | 17.165(3) |
α (°) | 90 | 90 | 104.31(3) | 87.87(3) |
β (°) | 94.0570(10) | 90 | 90.04(3) | 78.88(3) |
γ (°) | 90 | 90 | 110.21(3) | 75.65(3) |
Volume (Å3) | 4386.1(2) | 4648.9(4) | 1081.4(4) | 1253.2(4) |
Z | 4 | 4 | 2 | 2 |
Dcalc (Mg m–3) | 1.421 | 0.711 | 1.484 | 1.445 |
μ (mm–1) | 1.040 | 0.493 | 1.057 | 0.921 |
F(000) | 1928 | 1028 | 498 | 562 |
Crystal size (mm) | 0.26 × 0.2 × 0.10 | 0.30 × 0.21 × 0.15 | 0.21 × 0.16 × 0.03 | 0.30 × 0.2 × 0.20 |
θ range (°) | 1.97–28.31 | 2.10–28.31 | 2.48–27.48 | 1.21–27.38 |
Limiting indices | –12 ≤ h ≤ 12 | –19 ≤ h ≤ 19 | 0 ≤ h ≤ 10 | 0 ≤ h ≤ 11 |
–24 ≤ k ≤ 23 | –12 ≤ k ≤ 21 | –11 ≤ k ≤ 11 | –10 ≤ k ≤ 11 | |
–28 ≤ l ≤ 33 | –16 ≤ l ≤ 25 | –21 ≤ l ≤ 20 | –21 ≤ l ≤ 22 | |
Reflections collected | 37,063 | 24,328 | 4833 | 5583 |
Unique reflections | 10 877 | 5767 | 3278 | 4583 |
Completeness to θ (%) | 99.5 (θ = 28.31°) | 99.8 (θ = 28.31°) | 97.4 (θ = 27.48°) | 98.1 (θ = 27.38°) |
Absorption correction | Empirical | Empirical | Empirical | Empirical |
Number of parameters | 523 | 280 | 274 | 316 |
Goodness-of-fit on F2 | 1.128 | 1.124 | 0.913 | 1.072 |
Final R indices [I > 2σ(I)] | R1 = 0.0420, wR2 = 0.1141 | R1 = 0.0390, wR2 = 0.0972 | R1 = 0.0410, wR2 = 0.0704 | R1 = 0.0391, wR2 = 0.1058 |
R indices (all data) | R1 = 0.0927, wR2 = 0.1318 | R1 = 0.0819, wR2 = 0.1039 | R1 = 0.0785, wR2 = 0.0770 | R1 = 0.0517, wR2 = 0.1120 |
Largest diff. peak, hole (e Å–3) | 0.735, –0.285 | 0.321, –0.320 | 0.342, –0.441 | 0.622, –0.418 |
Acknowledgements
This project was supported by NSFC No. 20473099. K.W. was supported by DAAD postdoctoral fellowship.
Vous devez vous connecter pour continuer.
S'authentifier