1 Introduction
Polyaniline (PAn), one of the most thoroughly studied conducting polymers, has received great attention due to its thermal and environmental stabilities, as well as its energy storage, sensible and electrochemical properties [1–3]. Nanostructure of polyaniline attracts attention along with the development of nanoscience. Nanostructural polyaniline has been achieved by two main methods: (1) “template” and (2) “template free”. In method (1), “soft template” and “hard template” are widely used. “Template free” method includes self-assemble [4], physics [5] and electrochemical method [6].
“Soft template” method includes emulsion [7,8], microemulsion [9–11], diffusion polymerization [12,13], and interfacial polymerization, which can be regarded as an efficient method for preparing polyaniline nanofibers [14,15]. And the phase transferring method to synthesize conducting nanostructure is an interesting synthesis route to nanostructure building. Carswell et al. [16] controlled the nanostructure of PAn and polypryrole by absorbed surfactant which can transfer the micelles' phase from spherical to cylindrical and to planar, through the coadsorbing molecules. Jang et al. [17,18] added FeCl3 into surfactant AOT/isooctane mixture and AOT reversed micelles became cylindrical phase because Fe3+ ion decreases the second critical concentration of AOT, and hollow nanotubes can be achieved through this kind of “soft template” method.
“Hard template” methods always use membrane [19], porous materials [20,21] and etched template [22]. Numata et al. [23] demonstrated a method to synthesize polyaniline nanofibers by a polymer wrapping method using β-1,3-glucan (schizophyllan), which can form a tunnel used to direct the growth of polyaniline nanofibers, namely a controllable template.
In this paper, we introduced a series of nanostructures of polyaniline synthesis methods in facile conditions, and found that the nanostructure can be controlled by changing the condition such as monomer/oxidant adding procedure and rate, monomer concentration, and monomer/stabilizer ratio. The different shapes of the product, including nanoscale star, vertical net, fiber, and coral surface sphere are synthesized. The products are characterized by SEM, XRD and FTIR.
2 Experiment and materials
2.1 Preparation of PAn with different nanostructure
Aniline (An) monomer was distilled twice in nitrogen atmosphere. Ammonium persulfate (APS, AR), PEG-600 (polyethylene glycol, MW = 600, C.P.) and Triton X-100 (t-oct-C6H4–(OCH2CH2)xOH, x = 9–10, C.P.) were used as received without further treatment.
Experiments were processed in two different routes: (1) An and 95 mL water were first added into the flask. APS aqueous solution of 5.0 mL was added dropwise at different rates under stirring. Stabilizer of different ratios was added into the flask, and compared with the products without stabilizer. (2) An and APS aqueous solution (5 mL) were added dropwise simultaneously into a flask containing 90 mL of stabilizer aqueous solution, under stirring during 3 h. All experiments took place at ambient temperature and with equal molecular ratio between An and APS. After 24 h, methanol was added to cease the reaction and precipitate the product, which was washed with de-ionized water and ethanol to remove the impurities and low molecular weight product. Detailed experimental conditions are given in Table 1.
Detailed experimental conditions utilizing Triton X-100 as stabilizer
An | m(An)/m(Triton X-100) | pH | Oxidant adding time | Remark |
10 mmol/L | 4 | 3 h | Route 1 | |
10 mmol/L | 4 | 20 min | Route 1 | |
10 mmol/L | 10:1 | 4 | 20 min | Route 1 |
5 mmol/L | 10:1 | 4 | 20 min | Route 1 |
1 mmol/L | 10:1 | 4 | 20 min | Route 1 |
10 mmol/L | 10:5 | 4 | 20 min | Route 1 |
10 mmol/L | 4 | 3 h | Route 2 | |
10 mmol/L | 10:1 | 4,3,2, respectively | 3 h | Route 2 |
10 mmol/L | 10:3 | 3 h | Route 2 | |
10 mmol/L | 10:5 | 3 h | Route 2 | |
10 mmol/L | 10:7 | 3 h | Route 2 | |
10 mmol/L | 10:10 | 3 h | Route 2 |
The experiments with PEG-600 replacing Triton X-100 took place at m(An)/m(PEG-600) = 10:3, 10:5, 10:7, 10:10, and pH = 4. And the procedure of the polymerization and product treatment were the same as mentioned above.
2.2 PAn structure characterization
The FTIR spectra of the samples were recorded by Nicolet Avatar 360 Fourier infrared spectrophotometer (FTIR) with the KBr pellet technique. Scanning electron measurement (SEM) was performed with a LEO 1530 (Oxford Instrument, England). Measurement of X-ray diffraction spectra was performed on Panalytical X′Pert diffractometer (Philips, Holland).
3 Results and discussion
3.1 SEM study on the nanostructure of PAn
Fig. 1A shows SEM photos of PAn with oxidant, APS addition in 3 h. Compared with Fig. 1B, SEM photo of polymer with oxidant addition in 20 min, the fiber diameter of Fig. 1A (∼20 nm) is smaller than the one of Fig. 1B (∼120 nm), whereas the sample 2 has a porous framework. Because porous materials have a larger specific surface area, this may act as a promising catalyst carrier. The difference of the products between two adding rates of oxidant demonstrates that slower adding rate results in relatively smaller diameter nanofiber. It is believed that aniline monomer with hydrophilic group (–NH2) and hydrophobic group (–C6H5) can be used as emulsifier, tending to self-assemble into fiber [24]. And through the experiments, oxidant adding rate is proven as an important factor deciding the feature of the product.
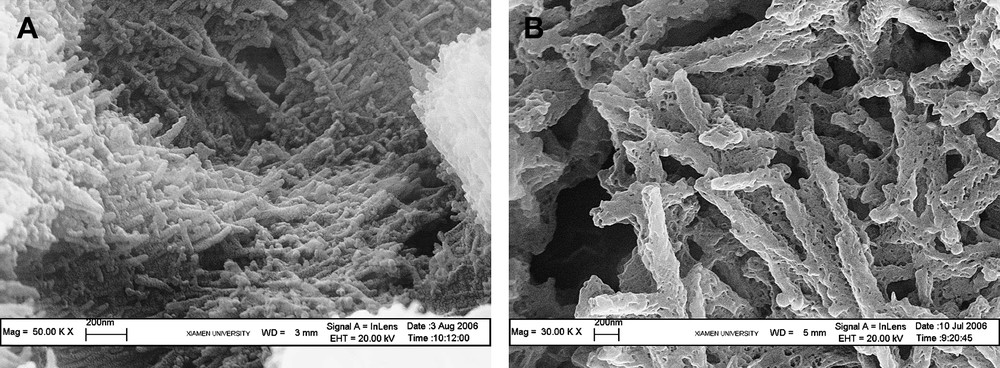
SEM micrographs of PAn with different oxidant adding rates. [An] = 10 mol/L, [APS] = [An], pH = 4, A: addition in 3 h, B: addition in 20 min.
Compared with Fig. 1, nanofibers of polyaniline (Fig. 2A) have smaller diameter and longer length, when stabilizer Triton X-100 was introduced into the reaction system, although not so evident. To understand the influence of monomer concentration, reactions with different concentrations of monomer take place. The cross particle was found in higher monomer concentration (Fig. 2B and 2C), which was not provided in the literature.
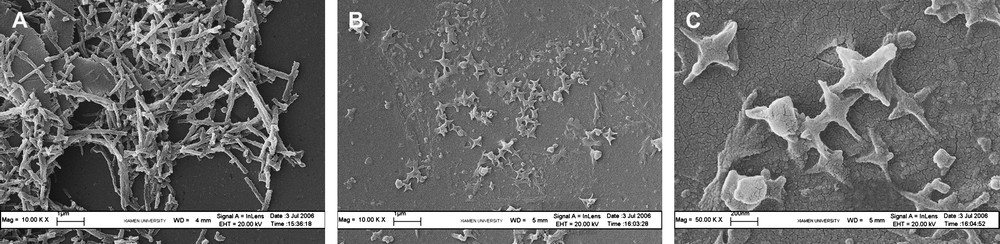
SEM micrographs of PAn with oxidant addition in 20 min. [APS] = [An], pH = 4, m(An)/m(Triton X-100) = 10:1, A: [An] = 10 mmol/L, B: [An] = 5 mmol/L, C: [An] = 1 mmol/L.
Route 2, in which oxidant and An monomer were added simultaneous is designed to understand the influence of monomer-to-stabilizer ratio on the final structure. A series of net-like polymer nanofibers are produced via the typical photo (Fig. 3). They are almost perpendicular with each other, especially in Fig. 3C, and the fibers in Fig. 3C, whose sample has the highest monomer/stabilizer ratio have the least size. Intercrossed nanofiber was reported before [1,25], whereas the reported structure was random and irregular, and the tendency of congregation was not so easy to resolve. A series of PAn nanofibers in the article illuminates the monomer/stabilizer ratio and pH of aqueous solution affects the regularity of polymer nanofibers. When the aqueous solution is at high pH value and stabilizer/monomer ratio, the product has a regular size and a net-like structure.
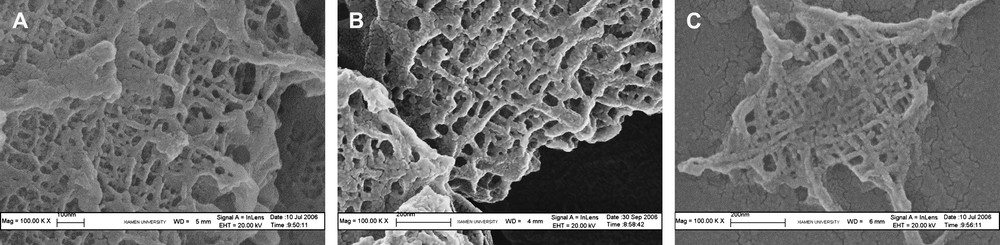
SEM micrographs of PAn with oxidant and monomer addition, respectively, and simultaneously. [An] = 10 mmol/L, [APS] = [An], pH = 4. A: m(An)/m(Triton X-100) = 10:3, B: m(An)/m(Triton X-100) = 10:7, C: m(An)/m(Triton X-100) = 10:10.
Experiments in which Triton X-100 was replaced by PEG-600 are taken at the same ratio of monomer/stabilizer by route 2. Triton X-100 and PEG-600 are partially similar, because of the same repeat unit (CH2CH2O) and proximate molecular weight. The difference between them is the hydrophobic group existing in Triton X-100, which makes the molecules assemble in aqueous solution spontaneously. When adding PEG-600, it can be observed from the typical photos that the microspheres were assembled by coral-like nanometer fibers (Fig. 4).
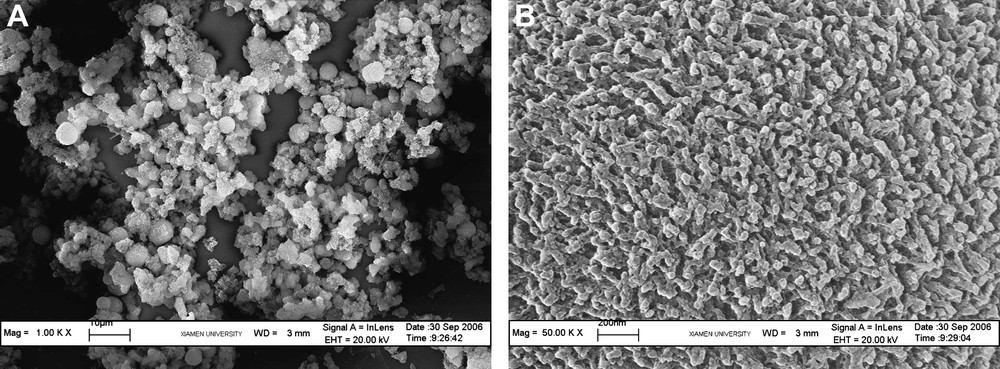
SEM micrographs of PAn with PEG as stabilizer. [An] = 10 mmol/L, [APS] = [An], pH = 4, m(An)/m(PEG-600) = 10:10, B is the surface magnified photo of spherical particles in A.
It is believed that at the first step PAn nuclei emerge [26], then nanofibers can grow on the nuclei. The diameter of the nanofibers is almost the same as nuclei. The polymerization rate and polymer particle formation rate reduce versus the stabilizer concentration [27]. The soft nonionic surfactant tail hinders the persulfate ion close to the active nuclei and reduces the rate of particle growth rate [28]. So higher concentration of stabilizer benefits to engender a unique and integral product, which is the reason for regular product synthesized at a high monomer/stabilizer ratio.
It is believed that An oligomer emerges at all pH values, especially at high pH values (pH > 4), whereas An macromolecule forms rapidly at low value (pH < 2). When pH > 4, An exists as a neutral molecule and is easily attacked by an oxidant. But if pH < 4, An is protonated to anilinium cation [29,30] and oxygen atom in the stabilizer backbone can absorb on anilinium cation more easily. In other words, the lower the pH value, the higher the polymerization rate. In our experiments, at a high pH value, the stabilizer has enough time to absorb on the nanofibers' surface and makes the product regular before its growth in size. Several shape of the final product can be observed. The reason for different structures is: the monomer aggregates in the surface of surfactant micelles so that the concentration of monomer on the micelles is higher than that in bulk solution, namely so-called “micelle-aided polymerization”. And with the growing concentration, the shape of micelles will change from spheroid to bar-like, branch-like, etc. [31]. That is the reason why the net-like structure is regular at low acidity, and relatively high concentration of stabilizer. And we suppose PEG does not have enough ability to arrange on the surface of the active particle, so the size of the particle is relatively large. But the balls of polyaniline maintain a coral-like nanostructure.
3.2 FTIR and XRD study on the nanostructural PAn chain structure
Typical FTIR spectra are shown in Fig. 5. The peaks at 3430 cm−1 and 3280 cm−1 are the free and hydrogen-bond N–H, respectively [32]. Absorption peaks at 1573 cm−1 and 1500 cm−1 are the stretching of quinonoid and benzenoid rings, respectively. C–N and CN stretching vibrations are at about 1290 cm−1 and 1174 cm−1, the same as literature reported [33]. Peak at 1174 cm−1, corresponding to the acid doping level, is smaller than typical PAn [32]; therefore our acid concentration is relatively low, not adequate for doping. A shoulder peak appears at 1640 cm−1, which is assigned to the carbonyl group (CO), different from typical PAn, because of the quinone formation during polymerization process [34]. In addition, 1443 cm−1 and 1041 cm−1 are assigned to the N–N single bond, which is the defect of the polymer [34]. IR spectra of different monomer/oxidant adding method and monomer/stabilizer ratio are almost identical, even in different stabilizer. The peak at 1174 cm−1 heightens with the decrease of pH value of solution, namely doping level heightening. It indicates that the pH value is the key influence to their chain structure.
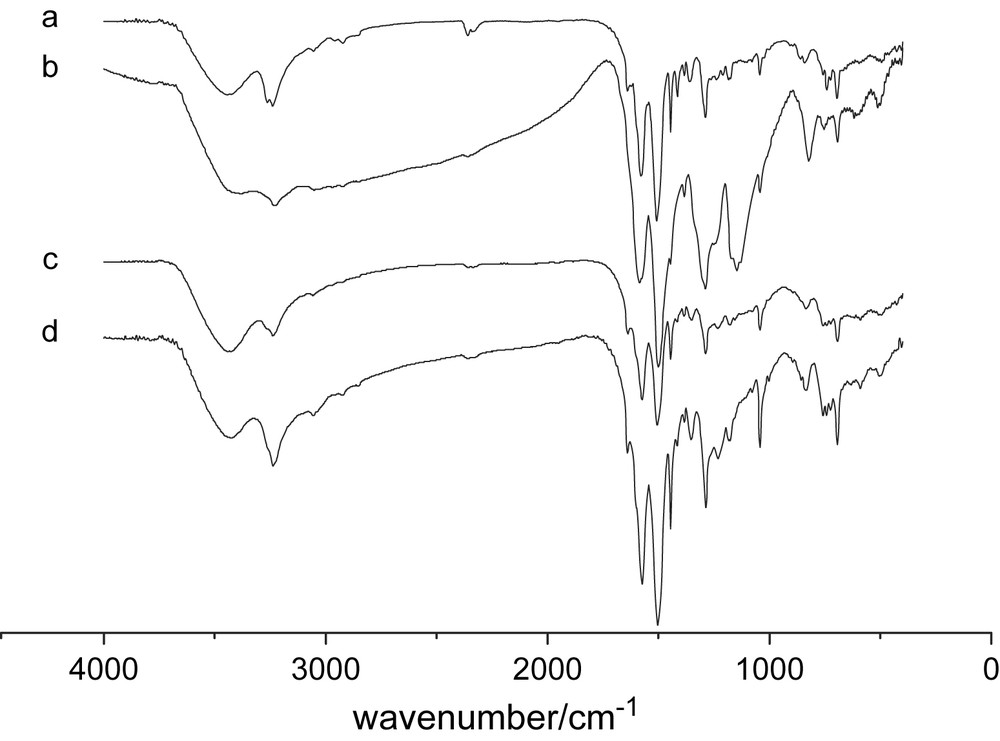
FTIR spectra of products in different conditions [An] = 10 mmol, oxidant and monomer addition, respectively, and simultaneously (a) pH = 4, m(An)/m(Triton X-100) = 10:3, (b) pH = 2, m(An)/m(Triton X-100) = 10:3, (c) pH = 4, m(An)/m(PEG-600) = 10:3, (d) pure PAn synthesized without stabilizer.
XRD spectra of PAn at different monomer/stabilizer ratios are almost the same (Fig. 6). Typical spectra are chosen to demonstrate the influence of acidity. Several peaks centered at 19° and 25° are ascribed to the periodicity parallel and perpendicular to the polymer chain [15]. A special peak center at 6.9° may arise from interplanar distance between polyaniline molecules [6] or reflection to periodic distance between dopant and N atom on adjacent main chain [4]. And the peaks at 6.9°and 25° decrease with lower pH, demonstrating that solid-state order degree reduces, consistent with the SEM and FTIR results. And XRD spectra of samples synthesized in PEG (Fig. 6e) and pure polyaniline without stabilizer (Fig. 6a) are the same as the ones of the products synthesized in Triton X-100, although the crystallization decreases with Triton X-100, PEG, and pure polyaniline. Maybe it is because that the emulsifier, Triton X-100, has better stabilizing ability.
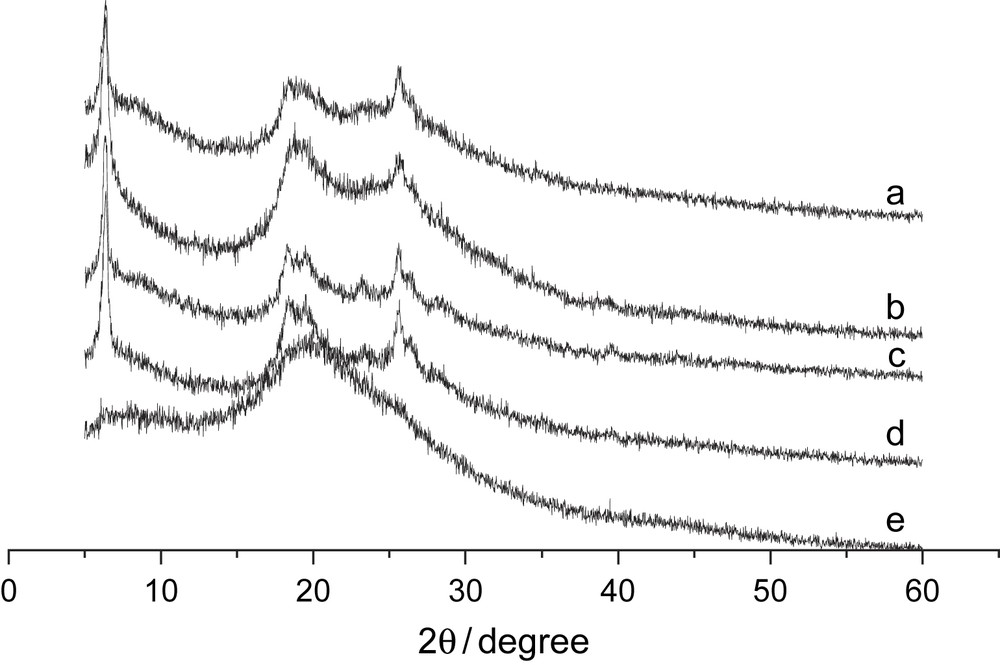
XRD pattern of PAn at different stabilizer and pH value condition. (a) Pure PAn without disperser; (b) [An]/[Triton] = 10:7, pH = 2; (c) [An]/[Triton] = 10:7, pH = 3; (d) [An]/[Triton] = 10:7, pH = 4; (e) [An]/[PEG] = 10:7, pH = 4.
Acknowledgements
The authors greatly acknowledge supports from Scientific and Technical Major Project of of Fujian Province (2006I0026), Natural Science Fundation of Fujian Province of China (2006J0174, E0310003), Natural Science Foundation of Fujian Province of China (2006J0174), and Scientific and Technical Project of Xiamen City (3502Z20055020).