1 Introduction
Investigations on late-transition metal complexes as catalysts for olefin oligomerization and polymerization have rapidly increased in a dozen of years, which were initiated with nickel and palladium catalysts by the Brookhart group [1], as well as iron and cobalt catalysts developed simultaneously by Brookhart [2] and Gibson groups [3]. Relative research works have been focused on modifying those catalysts' models through synthesizing numerous derivatives of their complexes and also devising alternative models of complex catalysts. The progress of fast-expending researches on nickel catalysts has been nicely considered in several recent review articles [4–9]. However, there are few models of complexes' catalysts of iron and cobalt complexes performing good catalytic activities toward ethylene oligomerization and polymerization in comparison with various models of nickel complexes. There are some significant advantages for iron and cobalt catalysts, such as high catalytic activity, high α-olefin selectivity in ethylene oligomerization, and lower toxicity than other metals. Therefore intensive screening researches on new molecular models of iron and cobalt complexes are still highly attractive, and the comprehensive review papers are reported [10,11].
Most alternative models of iron and cobalt complexes showed lower catalytic activities than that of bis(imino)pyridyl metal catalogues [11]. Fortunately, the derivatives of bis(imino)pyridyl metal (Fe or Co) dihalides showed some unique properties in our results, extensively, the 6-carboxalate-2-iminopyridyl metal halides showed catalytic activities toward ethylene oligomerization. Furthermore, inspired by the coordination electronic configuration of bis(imino)pyridyl metal (Fe or Co) dihalides, the models of metal complexes bearing 2-imino-1,10-phenanthrolines and 6-organyl-2-(imino)pyridines were devised and proved to be successful in ethylene oligomerization and polymerization. Herein, our experiences were outlined with parts as derivatives of bis(imino)pyridyl iron dihalides and hypothesis of active species, endeavoring works toward metal complexes bearing 2-imino-1,10-phenanthrolines, and novel metal complexes ligated by a series of 6-organyl-2-(imino)pyridines.
2 The typical iron catalyst and the hypothesis of active species
2.1 Symmetrical and unsymmetrical 2,6-bis(imino)pyridyl iron(II) complexes
The highly active 2,6-bis(imino)pyridyl iron(II) catalysts [2,3] moved more chemists into the investigation of late-transition metal complexes as catalysts for olefin oligomerization and polymerization. In the beginning, numerous 2,6-bis(imino)pyridyl iron analogues were prepared for detail comparative investigation, and three complexes performed significantly different catalytic activities in ethylene reactivity. (Fig. 1) [12]. The complex 1 with substituted phenyl groups showed high activities toward ethylene polymerization as reported under treatment with co-catalyst of MAO. However, complexes 2 and 3 exhibited different properties: no activity for 2, and meanwhile good ethylene oligomerization for 3. Within the same coordination frame of complexes, their different catalytic performances reflected the significant influences of substituents linked on ligands, in another word, the electronic and steric effects of ligands.
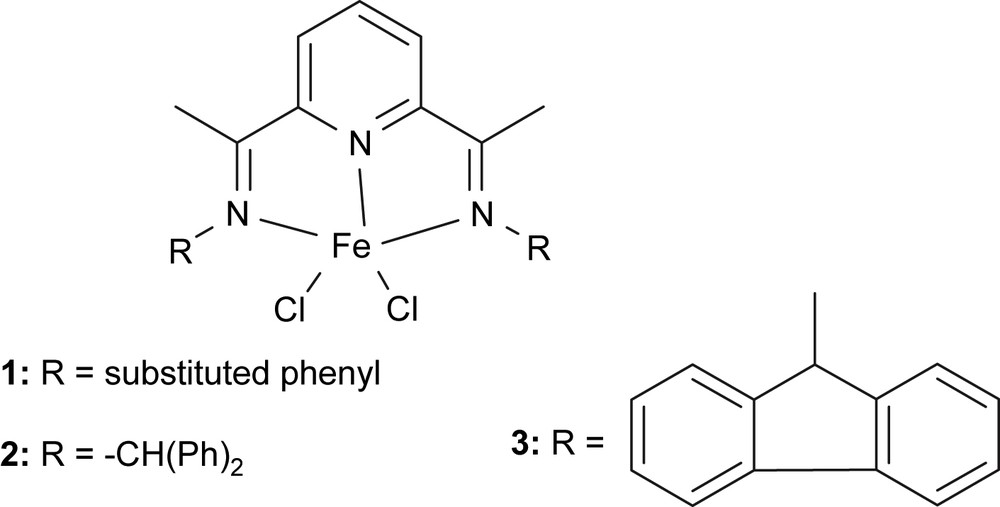
Symmetrical and unsymmetrical 2,6-bis(imino)pyridine iron(II) complexes.
Considering commercial catalysts in heterogeneous forms, the suitable carriers were selected in order to make supported iron catalysts for ethylene polymerization. The SiO2-supported catalysts showed a little lower activity with acceptable morphology of resultant polyethylene particles [13]. However, the better carrier was generally found to be spherical MgCl2 for late-transition metal catalysts [14,15]. Beyond that, simulation modeling comprising experimental works was carried out. The metal atom net charge correlation (MANCC) was employed to study the relative catalytic activities of bis(imino)pyridyl iron(II) and bis(imino)pyrimidyl iron(II) complexes for olefin oligomerization/polymerization [16]. It was concluded that the catalytic activity was more relied on the electronic configuration of the catalyst, especially the net charge on the central metal atom. Therefore, the magnitude of the net charge characterizes the activity of connecting bonds during polymerization.
2.2 2-(Ethylcarboxylato)-6-iminopyridyl iron and cobalt complexes
2,6-Diacetylpyridine is a basic substance in forming bis(imino)pyridines for catalyst preparation; however, it was too expensive for us to buy 2,6-diacetylpyridine. Instead, 2,6-diacetylpyridine was prepared by the reaction of 2,6-dicarbethoxypyridine with excessive amount of sodium ethanolate. Surprisingly, a byproduct of 6-acetylpyridine-2-carboxylate was often observed in small fraction or trace amount. By controlling the amount of NaOEt, 2,6-diacetylpyridine and 6-acetylpyridine-2-carboxylate could be individually obtained [17]. With our synthetic procedure, these two compounds are commercially available at reasonable price in China. In order to use 6-acetylpyridine-2-carboxylate, 2-(ethylcarboxylato)-6-iminopyridines and their metal complexes were generally prepared and investigated [17–19]. Though 2-(ethylcarboxylato)-6-iminopyridyl iron and cobalt complexes (Fig. 2) showed moderate catalytic activity (up to 105 g (mol metal)−1 h−1) toward ethylene oligomerization and polymerization in the presence of excessive amount of MAO, the prospect of iron and cobalt catalysts was the high selectivity for α-olefins. In addition, 6-acetylpyridine-2-carboxylate could be a substance for synthesizing new heterocyclic compounds as suitable ligands for complex catalysts (see Sections 5.2 and 6).
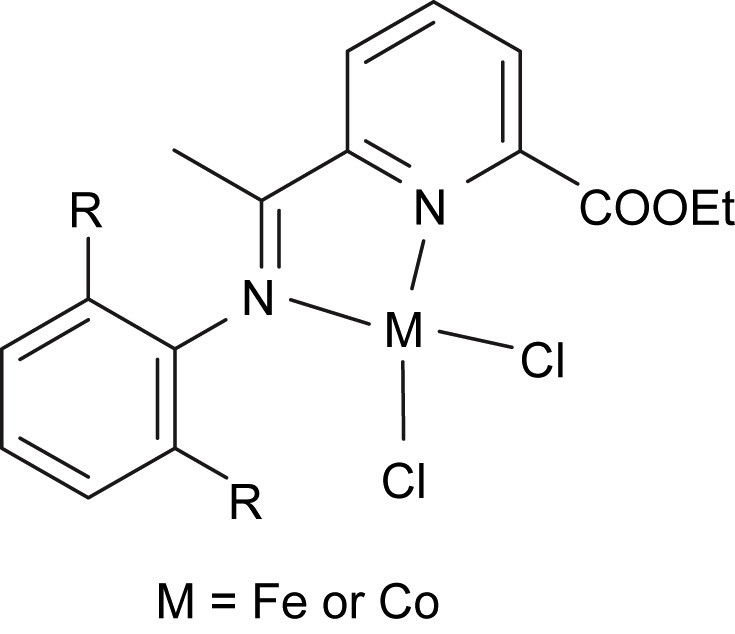
2-(Ethylcarboxylato)-6-iminopyrid iron and cobalt catalysts.
3 “Active species” and hypothesis of molecular models
The late-transition metal catalysts are also called post-metallocene catalysts; interestingly, most complex catalysts perform better catalytic activity with methylaluminoxane (MAO), though not always. As the case specialized, let us have a careful look at active species of common (iron, cobalt and nickel) complexes' catalysts compared to zirconocene catalyst. Their catalytic precursors are shown in Fig. 3. It is commonly accepted that those precursors will be alkylated into “active species”, which are dialkylated intermediates, such as metallocene [20], nickel catalyst [21], iron catalyst [22,23], with the exception of monoalkylated cobalt active species [23,24]. It is not difficult to find the 14e intermediate model for those “active species”, and such coordinative features perform the good to high catalytic activity in ethylene oligomerization and polymerization.
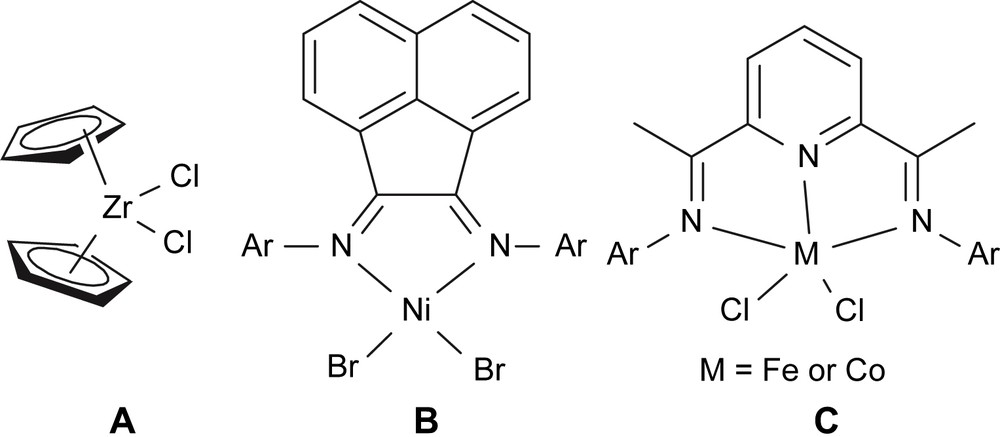
Typical complex pre-catalyst.
This hypothesis was supported by the iron dichloride- bearing 2-imino-6-pyridyl pyridines, in which the catalytic system with MAO showed good activity for ethylene oligomerization [25]. The extension to cobalt complexes bearing 6-organyl-2-iminopyridines also provided catalytic system for ethylene oligomerization [26]. However, newly designed iron and cobalt complexes generally showed lower catalytic activity than the complexes containing bis(imino)pyridines. Moreover, steric influences of ligand along with the constrained geometry will be also of importance. Based upon tridentate ligands favorable to iron catalytic models, our further explorations have been focused on devising alternative ligands of 6-organyl-2-iminopyridines and 2-imino-1,10-phenanthrolines.
4 Iron(II) and cobalt(II) complexes bearing 2-imino-1,10-phenanthrolines
Inspired by Brookhart's and Gibson's original work of the 2,6-bis(imino)pyridyl iron and cobalt complexes [2,3], we were interested in exploring new molecular models of complexes as catalysts for ethylene oligomerization and polymerization. In order to construct tridentate N^N^N ligands, the phenanthroline derivatives and their transition metal complexes were investigated through works of synthetic methodology and multi-step synthetic works. Although tremendous effort cost in multi-step syntheses, the highly active catalysts were successfully obtained for ethylene oligomerization and polymerization.
4.1 2,9-Bis(imino)-1,10-phenanthrolinyl iron(II) and cobalt(II) complexes
There are two parent chemicals commercially available, 1,10-phenanthroline and 2,9-dimethyl-1,10-phenanthroline. To construct tridentate nestle compounds as ligands for complexes, the transformation of the methyl group was potentially easy. At that stage, however, it was hard to selectively mono-functionalize 2,9-dimethyl-1,10-phenanthroline. The controlling oxidation provided 2,9-diformyl-1,10-phenanthroline, and therefore, 2,9-bis(imino)-1,10-phenanthrolines were prepared through the condensation reaction with various anilines, and then coordinated with metal halides (Fig. 4) [27]. The ligands could provide tridentate coordination feature which was confirmed by the single-crystal X-ray diffraction of their metal complexes.
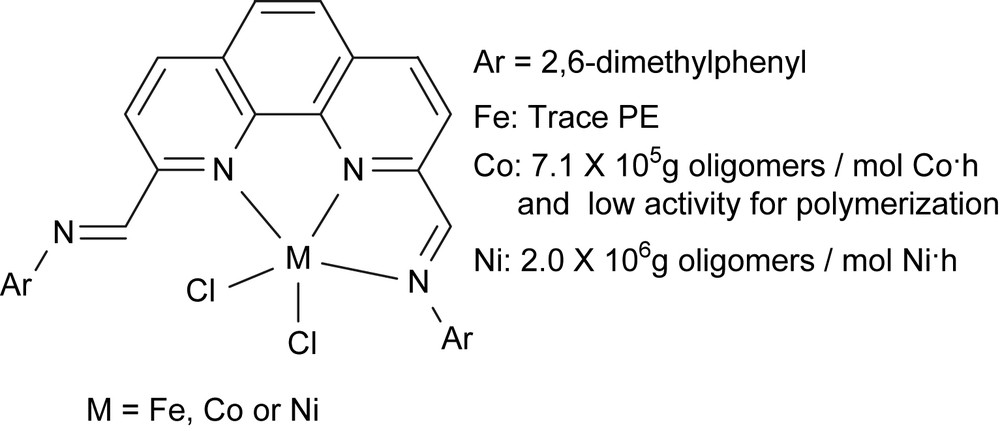
2,9-Bis(imino)-1,10-phenanthrolinyl metal complexes.
The original idea of designing these N^N^N tridentate compounds was to obtain the iron complexes as highly active catalysts. However, the iron complexes showed only negligible activities for ethylene polymerization. Fortunately, upon treatment with MAO, the cobalt complexes showed good activity for ethylene oligomerization and polymerization (up to 9.3 × 105 g mol−1 h−1); meanwhile the nickel analogues showed high activity for ethylene oligomerization. The nitrogen atom of the additional imino group potentially caused this problem because the additional coordination onto the active center (iron) would occupy the sites which were necessary for the coordination of ethylene in catalytic reaction. To verify the hypothesis that the additional imino group deactivated the iron species, the additional imino group should be removed. Therefore, our further effort was the synthesis of 2-imino-1,10-phenanthrolines and their metal complexes for ethylene reactivity.
4.2 2-Imino-1,10-phenanthrolinyl iron(II) and cobalt(II) complexes
All catalysts would not be recognized without approving their high performance (activity and selectivity) in special subjects. Regarding the background of polyolefins rooted in industrial application, the synthetic procedure of catalysts is necessary to have reliable substances and manipulation process. Therefore, we spent years on their preparative procedures of 2-formal-, 2-acetyl-, and 2-benzoyl-1,10-phenanthrolines from 1,10-phenanthroline. However, some expected products could not be obtained in traditional synthetic routines. For example, the hydrolysis of 2-cyano-1,10-phenanthroline gave 1,10-phenanthroline-2-carboxylic acid, which was expected to react with methanol for its ester. The esterification reaction could not happen, however, it was necessary to form its acetyl chloride, which then reacted with methanol. The total yield of 2-acetyl-1,10-phenanthroline was about 30% on the basis of best yields of intermediate substances observed in six-step syntheses (Fig. 5). Though there are many reports on the transformation of nitrile into ketones, 2-cyano-1,10-phenanthroline did not give acetylated product on reacting with methyllithium. The trimethylaluminum is one common reagent as co-catalyst in our group, and fortunately, the trial reaction of 2-cyano-1,10-phenanthroline with trimethylaluminum could produce 2-acetyl-1,10-phenanthroline in reasonable yield (Fig. 5). In a word, 2-acetyl-1,10-phenanthrolines could be prepared from 1,10-phenanthrolines with three-step reactions instead of six-step reactions [28].
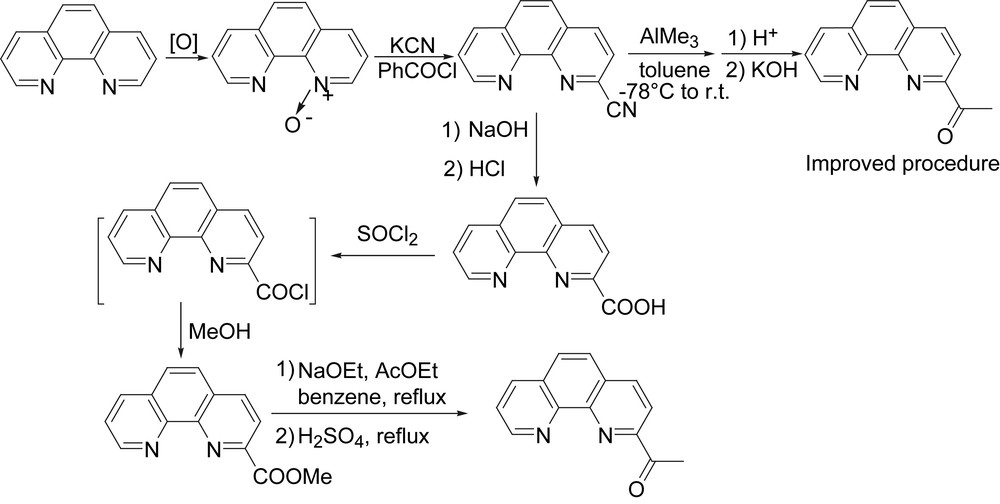
The synthetic procedure of the desired substance for ligands.
With the prepared 2-formal-, 2-acetyl-, and 2-benzoyl-1,10-phenanthrolines, the various 2-imino-1,10-phenanthroline derivatives were easily formed by the condensation reaction with anilines; further coordination with iron and cobalt dihalides gave the designed 2-imino-1,10-phenanthrolinyl metal complexes (Fig. 6) [28,29].
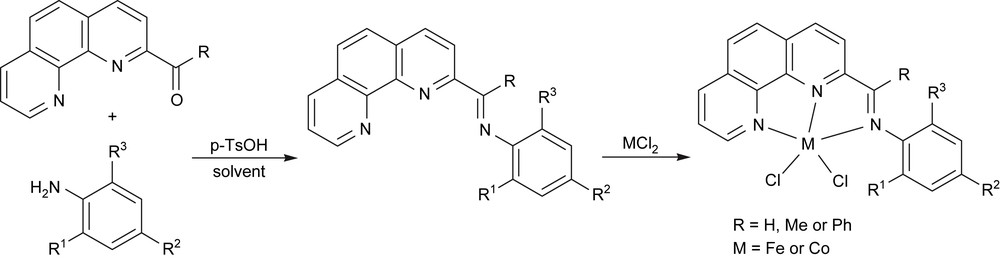
Synthesis of 2-imino-1,10-phenanthrolinyl metal complexes.
Upon treatment with MAO or MMAO, these iron complexes showed high catalytic activities for ethylene oligomerization with high α-olefin selectivity. MAO was found to be a more effective co-catalyst for iron catalytic system with activity up to 4.91 × 107 g mol−1(Fe) h−1 than MMAO [28]. The substituents' effects of ligands on the catalytic activities and the distributions of oligomer products were also investigated with various iron analogues containing different R, R1–3 substituents. Indeed, both the R on the imino-C and the substituents on the N-aryl rings had an obvious influence on the catalytic activity, distribution of oligomers, and selectivity for α-olefins due to their steric and electronic properties.
Aldimine (R = H) and phenyl-ketimine (R = Ph) complexes showed relatively lower activities than the corresponding methyl-ketimine (R = Me) complexes. Electron-donating groups placed on the N-aryl rings increased the selectivity for α-olefins. The placement of bulky o-alkyl groups on the N-aryl rings led to reduced activity because of steric interaction. Compared with bis(imino)pyridyl iron catalyst, 2-imino-1,10-phenanthrolinyl iron catalysts performed comparable catalytic activity toward ethylene reactivity, but more preferred to form oligomers and high order polyethylene waxes. Though there are more interesting catalysts for polyethylenes, such alternative catalytic process provides new substance with unique properties, especially the vinyl-type linear polyethylene waxes. More iron analogues were reported by our group [29].
Parallel research works for their cobalt analogues [30] were carried out, and the cobalt catalysts also showed good catalytic activities, which were lower than those of their iron analogues. The cobalt catalysts produced short-chain oligomers with mainly butenes and hexenes. MMAO was found to be a more effective co-catalyst at 1 atm ethylene pressure. Increasing the ethylene pressure to 10 atm, prolonged lifetime and improved catalytic activity were observed in the presence of MAO. The ligand environment greatly affected the catalytic performance. For the complexes bearing the same alkyl groups at the ortho-positions of the imino-N-aryl ring, the catalytic activities increased in the sequence: aldimine (R = H), methyl-ketimine (R = Me) and phenyl-ketimine (R = Ph), which was different from the case of their iron analogues. Beyond our research, there were more derivative analogues of cobalt catalysts reported by the Solan group [31].
In order to directly reflect the significant differences of catalytic activities of 2-imino-1,10-phenanthrolinyl metal complexes with or without the additional imino group, typical examples in ethylene reactivity are listed in Table 1. The 2,9-bis(imino)-1,10-phenanthrolinyl iron complexes showed no oligomerization activity, though one case had a little polyethylene produced. However, all the 2-imino-1,10-phenanthrolinyl iron complexes showed very high activity for both oligomerization and polymerization. Therefore, we can get a conclusion that the additional imino group coordinated onto the active catalytic center and prevented the coordination of ethylene. Regarding their cobalt analogues (Table 1), 2-imino-1,10-phenanthrolinyl cobalt complexes showed higher oligomerization activity and no polymerization activity. Though 2,9-bis(imino)-1,10-phenanthrolinyl cobalt complexes showed lower catalytic activity, polyethylenes were obtained. The characteristic difference of cobalt and iron complexes could also be observed.
Comparison of the catalytic performances of 2-imino-1,10-phenanthrolinyl metal complexes with or without additional imino group
Complex | R1 | R2 | Ao (g mol−1 h−1) | Ap (g mol−1 h−1) | Products |
i-Pr | H | No | No | ||
Me | H | No | 1.0 × 104 | PE | |
Me | Me | No | No | ||
i-Pr | H | 9.42 × 106 | 2.1 × 104 | C4–C28 and wax | |
Me | H | 3.89 × 107 | 1.02 × 107 | C4–C28 and wax | |
Me | Me | 1.24 × 107 | 1.38 × 107 | C4–C28 and wax | |
i-Pr | H | 7.0 × 105 | 6.0 × 103 | C4 and PE | |
Me | H | 7.1 × 105 | 9.6 × 104 | C4, C6 and PE | |
i-Pr | H | 1.26 × 106 | No | C4–C8 | |
Me | H | 6.7 × 105 | No | C4–C8 |
4.3 Additional remarks on complex catalysts bearing 1,10-phenanthrolinyl group
With the framework of 1,10-phenanthroline, reasonable efforts have been spent for various derivatives and their metal complexes in our group. With a view to developing complex catalysts for olefin oligomerization and polymerization, more research works including the synthesis of their derivative complexes and exploring alternative co-catalysts and optimum catalytic conditions is still worthwhile. In general, nickel complexes could be easily synthesized with various coordinative ligands. Therefore, more nickel complexes have been prepared with 1,10-phenanthroline derivatives, such as 2,9-disubstituted-1,10-phenanthrolines [32], 2-imino-1,10-phenanthrolines [33], 9-phenyl-2-imino-1,10-phenanthrolines [34], and 2-(benzimidazol-2-yl)-1,10-phenanthrolines [35] (Fig. 7). Though their nickel pre-catalysts showed good to high catalytic activities toward ethylene oligomerization, α-olefin selectivity was pretty low due to the catalytic mechanism of very fast alkyl migration on nickel active species.
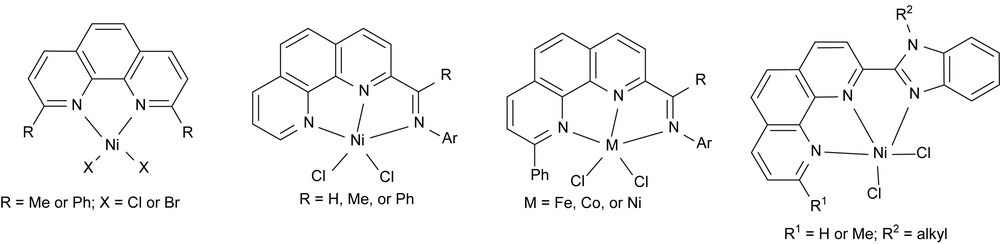
Nickel complexes bearing 1,10-phenanthroline derivatives.
In our experiences, the metal complexes ligated by those alternative derivatives of 1,10-phenanthrolines showed high catalytic activity toward ethylene reactivity. Moreover, some 1,10-phenanthroline derivatives could be synthesized in tens of grams of product in the laboratory. Those iron catalysts provided high activity for ethylene oligomerization, with good selectivity for α-olefins. Moreover, those catalytic systems produced less polyolefins; especially the polyolefins were polyolefin waxes without high molecular fractions. This is an advantage with regard to ethylene oligomerization process because any polyolefin with high molecular weight could potentially block the pipelines of the chemical process.
5 6-Organyl-2-(imino)ethylpyridyl iron(II) and cobalt(II) complexes
Though there are some reports regarding 6-organyl-2-(imino)pyridyl metal complexes mentioned above [17–19,25,26], the full scope of 6-organyl-2-(imino)pyridines has not been investigated yet. Driven by devising alternative tridentate metal complexes for catalysts, 6-(2-benzoimidazolyl)-2-iminopyridine and 6-(quinoxalinyl)-2-iminopyridines, as an alternative model to 6-pyridyl-2-imino-pyridines, appeared in our mind. As a matter of fact, our other challenge for iron complexes bearing 2,6-bis(2-benzoimidazolyl)pyridines was not successful within iron complex, but resulted in 2,6-bis(2-benzoimidazolyl)pyridyl chromium complexes and their catalysis for ethylene reactivity [36]. It was worthy to mention that the investigation of o-phenylenediamine was carried out in detail to form benzimidazoles, quinoxalines and 1,5-benzodiazepines by our group [37], meanwhile formation of 6-acetylpyridine-2-carboxylate as a starting substance [17] is making sense in our following research works.
5.1 6-Organyl-2-(benzoimidazolyl)pyridyl metal complexes
There are alternative approaching synthetic methods in preparing various 6-organyl-2-(benzoimidazolyl)pyridines targeting to 6-(2-benzoimidazolyl)-2-iminopyridines: the simplified ways were presented in our recent paper in detail [38]. To have a whole view of various metal complexes, both bidentate and tridentate iron and cobalt complexes were prepared and well characterized (Fig. 8) [38].
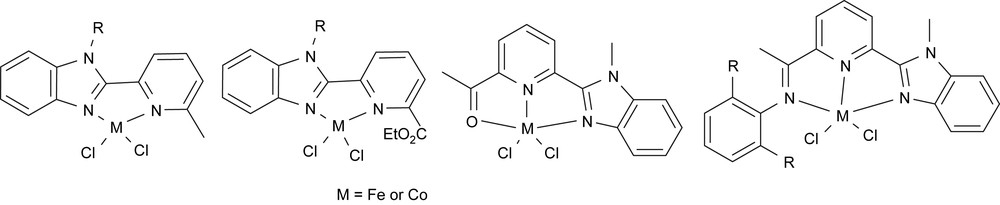
6-Organyl-2-(benzoimidazolyl)pyridyl iron(II) and cobalt(II) complexes.
Those iron and cobalt complexes were generally investigated for ethylene reactivity with the assistance of various organoaluminium co-catalysts. In general, the tridentate complexes showed relatively higher activity than the bidentate complexes. For the bidentate complexes, considerable catalytic activities of iron complexes were obtained with co-catalysts such as MAO, MMAO and Et2AlCl; but the catalytic system with MAO showed relatively lower activities. However, their bidentate cobalt analogues showed very low activities toward ethylene reactivity with co-catalysts of MAO and MMAO, but good activities with Et2AlCl.
In the presence of MAO, the tridentate iron complexes performed good to high catalytic activities, up to 1.43 × 107 g mol−1 h−1 in producing α-olefins (C4–C28) with very high selectivity (>99%). In addition to high selectivity for α-olefins, some waxes were also obtained in some iron catalytic systems. The steric effect of ligands on the activities was easily observed when they were activated by MAO. Changing the R group on the ortho-positions of the N-aryl group, their oligomerization activity varied in the orders of Me < Et < i-Pr and Cl < Br, and the bulky group protected the metal center and consequently resulted in higher activity. The catalysts with electron-withdrawing group showed lower activity than that of catalysts with electron-donating group. The distribution of oligomers produced resembled Schulz–Flory rules. In addition, different ethylene pressure exhibited strong influence on ethylene reactivity. At ambient ethylene pressure, only C4 and C6 were produced. The higher pressure increased the chain propagation, leading to longer chain oligomers up to the range of C4–C28 along with the improved activity. For their tridentate cobalt analogues, however, moderate catalytic activity was observed for ethylene oligomerization, with good selectivity for α-olefins.
The reported paper contained parts of our results [38]; there are more works under progress, including further investigation for optimum catalytic conditions and derivatives along with other metal analogues [39].
5.2 2-Quinoxalinyl-6-iminopyridyl iron and cobalt pre-catalysts
This work was carried out in order to find more applications of new the organic synthon, 6-acetylpyridine-2-carboxylate [17], which is commercially available in China. The suitable methodology was developed for the preparation of 6-(2-quinoxalinyl)-2-acetylpyridine, and numerous 2-quinoxalinyl-6-iminopyridines were easily synthesized by the condensation reaction with anilines and further coordinated with iron and cobalt dichlorides to provide pre-catalysts for ethylene oligomerization (Fig. 9) [40].
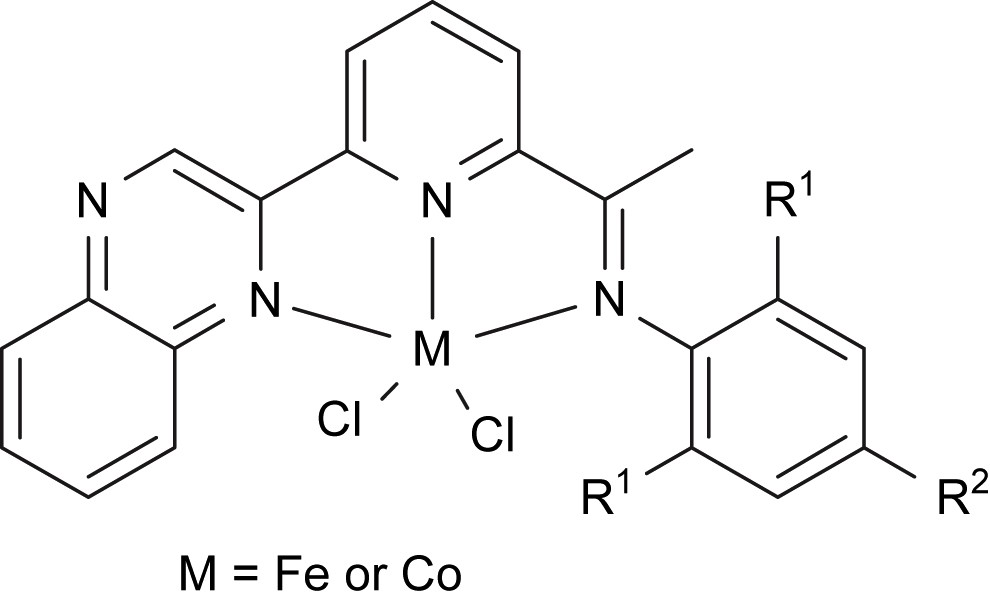
Iron and cobalt complexes ligated by 2-quinoxalinyl-6-iminopyridines.
Initial scanning of Et2AlCl, MMAO and MAO as co-catalysts of the iron complexes for ethylene reactivity showed that the iron catalytic system containing MAO gave the highest activities for ethylene oligomerization, and the butenes were observed as the major products. At 10 atm of ethylene pressure, both activity and contents of longer-chain oligomers increased. In some cases, the polyethylene waxes were collected to prove good polymerization activity; one example showed 2.24 × 106 g (mol Fe)−1 h−1 for oligomerization and 8.56 × 105 g (mol Fe)−1 h−1 for polymerization. Different substituents on the imino-N-aryl ring exhibited some influences on their catalytic performance. The bulky substituents at the ortho-positions of the imino-N-aryl ring resulted in lower catalytic activities and the complexes with electron-withdrawing groups showed similar catalytic reactivity as their analogues with electron-donating groups. In the presence of MMAO, all cobalt(II) analogues displayed considerable catalytic activities for ethylene oligomerization, with relatively lower activity than the iron(II) complexes. In addition, their nickel analogues showed good activity for ethylene oligomerization [41]. There are more detailed investigations under progress.
In a general consideration of 6-organyl-2-(imino)pyridyl metal catalysts for olefin oligomerization and polymerization, the literature works are still limited and extensive studies will be expected for valuable results.
5.3 Comparison of 2-benzoimidazolyl- and 2-quinoxalinyl-6-iminopyridyl metal complexes
These two kinds of iron and cobalt analogues showed different catalytic behaviors toward ethylene oligomerization and polymerization. Comparing their molecular structure typically shown in Fig. 10, the coordination geometry around the metal center was a distorted trigonal-bipyramidal with the pyridyl nitrogen atom and the two chlorides forming an equatorial plane. The nitrogen atom of the imino group and the other nitrogen atom of the benzimidazolyl or quinoxalinyl group form the axial plane. However, an obvious difference about bond length was determined; in benzimidazole complexes, the bond length of Fe–Nbenzimidazole is similar to that of Fe–Npyridine, but the bond length of Fe–Nquinoxaline is much longer than that of Fe–Npyridine in quinoxaline complexes, which can be explained by the high electron density on the coordinated Nbenzimidazole atom, and Fe–Nbenzimidazole is more stable than Fe–Nquinoxaline. The same phenomenon was also found in their cobalt analogues.
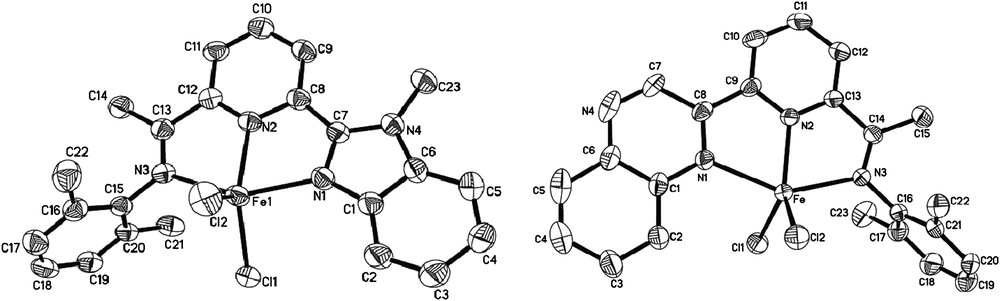
Molecular structures of 2-benzoimidazolyl- and 2-quinoxalinyl-6-iminopyridyl iron complexes with thermal ellipsoids at the 30% probability level. Hydrogen atoms have been omitted for clarity.
6 Bimetallic complex catalyst
The synergic effect of multinuclear complexes or cluster was often observed in catalysis of hydroformylation. Previously we obtained dinickel complexes for ethylene oligomerization and polymerization [42]. Therefore, we have kept an eye on developing bimetallic complexes as catalyst models for ethylene reactivity. The practical way is to bridge two catalyst models in one molecule. The most popular model is the bis(imino)pyridyl metal (iron or cobalt) catalysts [2,3], meanwhile the close model could be monoiminopyridyl metal complexes [17]. The fused molecule, 3,3-dihydro-2-methyl-2,4-bis(6-acetylpyridin-2-yl)-1H-1,5-benzodiazepine, which could link the two models together, was obtained by the reaction of o-phenylenediamine with diacetylpyridine. A further step, reaction with anilines, provided 2-methyl-2,4-bis(6-iminopyridin-2-yl)-1H-1,5-benzodiazepines for preparing bimetallic complexes [43] (Fig. 11).
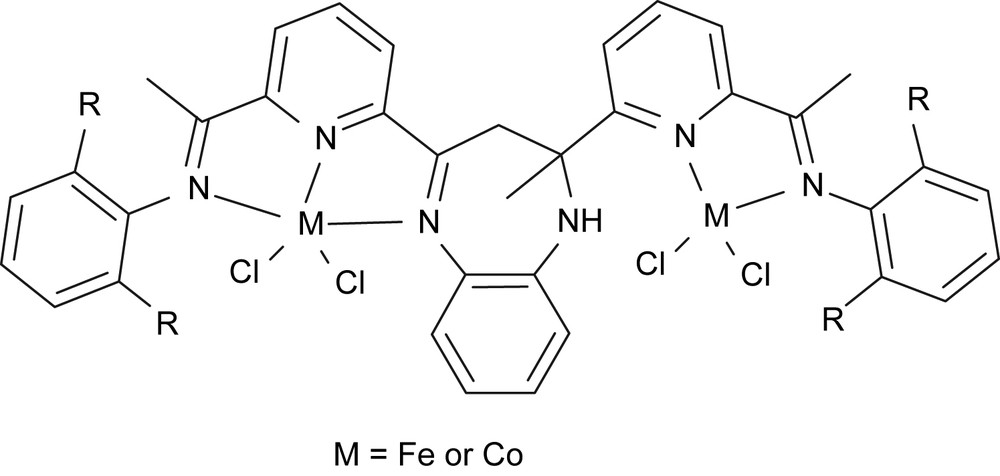
Bimetallic complexes.
The iron and cobalt complexes were studied for their catalytic activities with various alkylaluminums as co-catalysts. The catalytic system with modified methylaluminoxane (MMAO) showed high catalytic activities for ethylene oligomerization and polymerization with very high selectivity for α-olefin. The low-molecular-weight polyethylene wax was also produced with a large percentage (up to 75.0%). The ligands greatly affected the catalytic behavior of their complexes. Under the same reaction conditions, noticeably reduced catalytic activities were observed for the sterically bulkier catalyst systems. The catalytic activity, distribution of oligomers and percentage of polyethylene wax were also greatly affected by the reaction parameters such as Al/M molar ratio and reaction temperature. In general, the iron(II) complexes showed relatively higher activity than the cobalt(II) analogues. Moreover, their nickel analogues also performed good catalytic activities toward ethylene oligomerization [44]. Therefore, the bimetallic complexes could be new targeting catalysts, and the positive results encourage us for further exploration.
7 Conclusions and prospects
The N^N^N tridentate iron(II) and cobalt(II) complexes are promising catalysts for ethylene oligomerization due to their high catalytic activity and high α-olefin selectivity, meanwhile their polymerization provided unique materials with long-chain vinyl-type linear polyethylenes. In addition to bis(imino)pyridyl metal (iron or cobalt) catalysts, there are more alternative catalysts models performing high activity and selectivity for α-olefins. Our current results will be in small portion of catalysts' models in long-term consideration. Those works are closely related to industrial application, and further investigations will result in new conceptual conclusion of structural and homogeneous theory. Surely the development of new catalytic models will rely on the master works of organic synthesis, coordination, structural and physical chemistry.
The ethylene reactivity, oligomerization and polymerization, is core with fast and multi-steps of carbon–carbon coupling reactions. The high selectivity of linear α-olefins makes way for such catalysts in industrial practice with ethylene oligomerization. The unique properties of long-chain linear polyethylenes (waxes) will be argued for new conceptual polymers, as, for example, further oligomerization provides long branched polymers or copolymerization with other olefins for high molecular-weight polymers. Those polymers with high molecular weights will be difficult to crystallize, and will be potentially rubber materials. Beyond the current consideration, iron is one of the less toxic atoms, the extension of researches using those iron complexes could be considered for organic synthesis, likely as metallocene chemistry, with great influence on organic methodology and synthesis. The cobalt catalysts are found to be oxidation catalysts; the arguable question will be whether to use cobalt catalysts with multifunctional applications in catalysis.
The less investigated iron and cobalt complexes as catalysts will give more chances to scientists. There are more flexible complexes and structures to be found, more investigations will make those complexes useful and reliable for industrial processes.
Acknowledgements
This work was supported by NSFC Nos. 20473099 and 20674089, and MOST 2006AA03Z553. We are indebted to our group members for their contributions in developing this chemistry.