1 Introduction
In 1925, a German physical chemist, named Hans Zocher (Fig. 1a), reported the observation, by polarized light microscopy, of small birefringent domains in colloidal suspensions of vanadium pentoxide [1] (Fig. 1b). He called these domains “tactoids” from the Greek “to assemble” and correctly inferred that they must be made of small rod-like particles aligned in the same direction (note that the word “tactoid” is often used nowadays, with a different meaning, by clay scientists to describe small stacks of clay sheets). He also discussed the spontaneous formation of these aligned domains in comparison with the liquid-crystalline phases, newly discovered at this time, of small organic molecules. However, because liquid crystals were not yet well understood, such a comparison was indeed quite difficult. Moreover, the deep analogy between the so-called “thermotropic liquid crystals”, used in display technology, and the “lyotropic liquid crystals”, which are dispersions of surfactants or biomolecules, had not yet been understood [2]. Nevertheless, this was probably the first reported example of what we now call a mineral liquid crystal, i.e. a liquid-crystalline suspension of anisotropic inorganic nanoparticles in a solvent [3]. Unfortunately, these early observations were mostly forgotten by the end of the 20th century. Meanwhile, V2O5 gels raised much attention from the materials science community due to perspectives of applications in many fields: electrochromic layers, humidity sensors, reversible cathodes for lithium batteries, antistatic coatings… [4]. Besides, V2O5 suspensions have become famous as one of the best model systems used to study inorganic polycondensation by the sol/gel process, which led to the invention of “chimie douce” (an expression coined by Jacques Livage in an article in the French daily press [5]) that is a kind of inorganic chemistry performed at low temperature, in a solvent. Besides, one of the side effects of the “nano” trend, which still has to this date very strong momentum, is a renewed interest in colloidal suspensions of anisotropic nanoparticles and their possible liquid-crystalline properties. This is due to the fact that such suspensions form very good model systems to test statistical physics theories of liquid-crystalline ordering [6]. Moreover, these materials can combine the anisotropy and fluidity of liquid crystals with the typical electronic properties of inorganic materials such as magnetism or electronic conductivity. Meanwhile, the understanding and applications of usual organic liquid crystals had made huge progress, which nowadays provides a comprehensive basis of comparison for the suspensions of vanadium pentoxide ribbons.

a: photograph of Hans Zocher (reproduced with permission from the Brazilian Academy of Sciences); b: micrographs of “tactoids”, reproduced from reference [1] (Copyright Wiley-VCH Verlag GmbH & Co. KGaA. Reproduced with permission).
V2O5 suspensions display a liquid-crystalline nematic phase at concentrations larger than about 0.1 mol L−1 (or volume fraction ϕsol/gel ≈ 0.7%); they also show a sol/gel transition [7] at a concentration of 0.2 mol L−1 (or volume fraction ϕsol/gel ≈ 1.5%). At larger concentrations, they clearly show a finite yield stress whereas they are free-flowing liquids at lower concentrations. Nevertheless, the gels can be fluidized again by shaking, which proves that the gelation is reversible and does not involve covalent bonds; in other words V2O5 gels are “physical” gels. The mechanism of this sol/gel transition is not precisely known so far and the gel character sometimes complicates the interpretation of the other physical properties of the suspensions.
From a structural point of view, two main techniques demonstrate that the nanoparticles found in colloidal V2O5 gels are indeed ribbons. Transmission electron microscopy (TEM) images of xerogels (i.e. dried gels) display a fibrous network of ribbons, several microns long and 20–30 nm wide [8,9]. Moreover, small-angle X-ray scattering (SAXS) studies show a dependence of the scattered intensity, I(q), with scattering vector modulus that can be described with a ribbon form factor, using a thickness t = 1 nm, a width w = 25 nm and a length L = 300 nm. This latter value should rather be considered as the persistence length Lp of the ribbons (i.e. the length below which they can be considered as rigid and straight). The SAXS signature of the ribbon shape is a crossover, at q ≈ 2π/w from a q−1 dependence at small angles to a q−2 dependence at wider angles [10].
The microscopic structure of vanadium pentoxide ribbons has been investigated several times by various diffraction experiments on the xerogels [8,11]. The structure was refined very recently using the newly-developed “pair distribution function” technique that is particularly efficient in the case of nanometric particles that give diffraction patterns displaying wide peaks [12]. The results obtained are close to those of previous models and show that the ribbon structure is closely related to that of bulk crystalline (orthorhombic) V2O5. The ribbons are well-defined bilayers of single V2O5 layers made of pyramidal VO5 units running in parallel double chains that are connected via their corners to form the slabs. The bilayers are separated by water molecules the number of which depends on the water content. Actually, the detailed atomic structure of the ribbons will not be very relevant in the following.
In this review article, we summarize the most salient structural features and physical properties of these liquid-crystalline suspensions of vanadium pentoxide ribbons. In Section 2, the processes taking place during the synthesis of V2O5 gels will first be discussed from a physicist's perspective. Then, the liquid-crystalline nematic phase of V2O5 suspensions will be described in section 3. The alignment of the nematic phase by magnetic and electric fields will be discussed in Section 4. Section 5 gives a quick overview of the thermodynamics of vanadium pentoxide suspensions and of their phase diagram, including the report of a biaxial nematic gel state. Section 6 is devoted to an NMR study of these colloids and finally Section 7 reviews various applications of V2O5 gels in quite diverse areas of soft condensed matter.
2 Synthesis of V2O5 gels
To the best of our knowledge, the first synthesis of vanadium pentoxide gels was reported in 1885 by Ditte [13] who simply added a mineral acid to vanadate solutions. However, a more convenient way to synthesize these materials, by polycondensation of charge-neutral decavanadate species appearing in a very limited domain around pH2, consists in pouring a solution of sodium metavanadate NaVO3 through an ion-exchange resin column. The solution is recovered in successive vials as it flows out of the column. In some of these vials, the acidification of the solution leads to the polymerization of the precursors via olation and oxolation reactions [14]. This technique is particularly interesting because no foreign ions are introduced and the only ions in solution are protons and the VV condensed species. More recently, the phenomena occurring in these vials have been reexamined from a more physical perspective, using in situ SAXS, light scattering, optical microscopy, NMR, and rheology [15].
Interestingly, it should be stressed here that, in contrast with most colloids, the colloidal state of V2O5 ribbons seems actually to be a thermodynamically stable state. Indeed, if a crystalline V2O5 powder is brought in contact with water, on a time scale of about a year, this powder will swell and form a gel that has the same properties as those of V2O5 gels synthesized by acidification of a sodium decavanadate solution.
We now describe the evolution and aging of the V2O5 suspension from its starting point right after flowing out the column to the point where it can be considered as a mature liquid-crystalline gel. The most striking observation is the flocculation that takes place in the vial within a few hours. A precipitate of solid particles appears but, instead of sedimenting, it swells gradually to fill the whole vial and eventually redisperses to form a homogeneous phase. The flocculation can also be observed by optical microscopy and image treatment has shown that the structure of the flocculate, at the 1–1000 μm length scale, is actually fractal, with a fractal dimension df = 1.85. This value, which is confirmed by SAXS on a very different length scale (10–100 nm), is consistent with the cluster–cluster aggregation model [16] that predicts a fractal dimension ranging from 1.78 to 1.91 and often applies to colloids formed by nonequilibrium aggregation processes. This suggests that the material formed by the transient flocculation results from an isotropic and random aggregation of vanadium species.
SAXS experiments show that large macromolecular species appear during the first 4–6 h of aging, probably due to fast olation reactions that give rise to corner sharing chains. The number of macromolecules increases exponentially during this stage that also corresponds to the flocculation but reaches a plateau at longer times, which corresponds to the redispersion stage. The analysis of the shape of the SAXS curves, and in particular the location of the crossover from the q–1 to q–2 regimes, shows that the width of the ribbons increases during the redispersion stage. This stage may therefore correspond to the slower oxolation reactions that assemble the macromolecules into ribbons by edge sharing. The ribbon width reaches its final value after some 10–15 h, after the redispersion of the flocculate is completed. The fact that the ribbon width always lies in the 10–30 nm range, whatever the synthesis conditions and even the synthesis process, still remains unexplained. Besides, at this stage, the scattering curves can still be fitted with the ribbon form factor and there is still no need to introduce a structure factor. In other words, there is not yet any spatial organization of the ribbons. Even though the suspensions look very much like mature gels, they do not exhibit yet any liquid-like positional order. Indeed, such order requires a few days of aging to appear.
The synthesis of vanadium pentoxide gels was also followed by rheological experiments. A sudden increase of the elastic constant by two orders of magnitude takes place after about 10–12 h. The suspensions then show an elastic behaviour arising from entanglements typical of a gel. The elastic modulus and the yield stress then reach values comparable to those of mature gels (a few hundred Pa and 20 Pa, respectively). However, more subtle rheological nonlinear properties (like aging and rejuvenation) are still different from those of mature gels [17].
The appearance of liquid-crystalline features is the last stage of evolution of the suspensions. Indeed, birefringence, the typical liquid-crystalline property, only appears after about a week. At such large concentrations, the birefringence does not come from the formation of small nematic droplets (tactoids) but arises uniformly over the whole sample. It results from the slow and constrained motion of the ribbons that gradually align parallel to each other in these nematic gels. From a physicist's point of view, this slow evolution, in a gelled system, from an isotropic random highly entangled state to a nematic phase with liquid-like short-range order, is rather uncommon and puzzling. It should also be emphasized here that V2O5 suspensions were found to be stable over years (and even decades), from the points of view of colloidal stability and of liquid-crystalline and gel properties.
The most intuitive explanation of the unexpected flocculation/redispersion phenomenon consists in assuming that the flocculation is due to a decrease in surface charge and that the redispersion is due to the appearance of electrical charges on the ribbon surfaces. Indeed, once colloidal vanadium oxide macromolecules have been formed, water adsorption and dissociation occurs at their surface, giving rise to hydroxyl groups. However, because of the large positive charge of VV ions, an electron transfer occurs and surface V–OH groups exhibit acidic properties. V2O5 gels should therefore be described as polyvanadic acids. The ribbons are negatively charged and bear a linear charge density of about 5 e–/nm that is compensated by H+ ions. The complex flocculation/redispersion evolution could actually be followed by electrical conductivity measurements that showed a steep initial decrease of the conductivity during the flocculation stage, followed by a slow increase during the redispersion. Such experiments demonstrate that the electrostatic interactions play a major role in this phenomenon.
Finally, one should bear in mind that partial reduction of VV always takes place in V2O5 suspensions so that the ribbons can actually be considered as mixed-valence macromolecules, which gives them interesting conductivity properties [18]. A small amount (1–5 %) of VIV species is usually detected, for example by ESR, depending on synthesis conditions and sample handling (metallic tools should be avoided). This can be appreciated visually from the colour of the suspension because VV species are purple whereas VIV species are green. Partial reduction to about 5% of a suspension is therefore recognized by a brownish colour. Further reduction (> 10%) of a suspension brings about its flocculation.
3 Liquid-crystalline properties
When diluted with pure water, V2O5 gels, prepared by the ion-exchange technique, spontaneously display in vials a phase separation between a top isotropic phase and a bottom birefringent nematic phase (Fig. 2a). This striking phenomenon is very easily observed between crossed polarizers with the naked eye and is the nonambiguous signature of a thermodynamic first-order phase transition. The same transition is also readily observed by polarized-light microscopy using optical flat glass capillaries: small domains of the nematic phase form; they are Zocher's tactoids. If the capillaries are stored vertically, the tactoids sediment and merge to form the nematic phase. The tactoids have interesting physical properties. They are anisotropic and usually have a prolate (spindle-like) shape; the detailed analysis of their shape gives information about the elastic constants and the surface properties of the nematic phase at coexistence with the isotropic one [19].
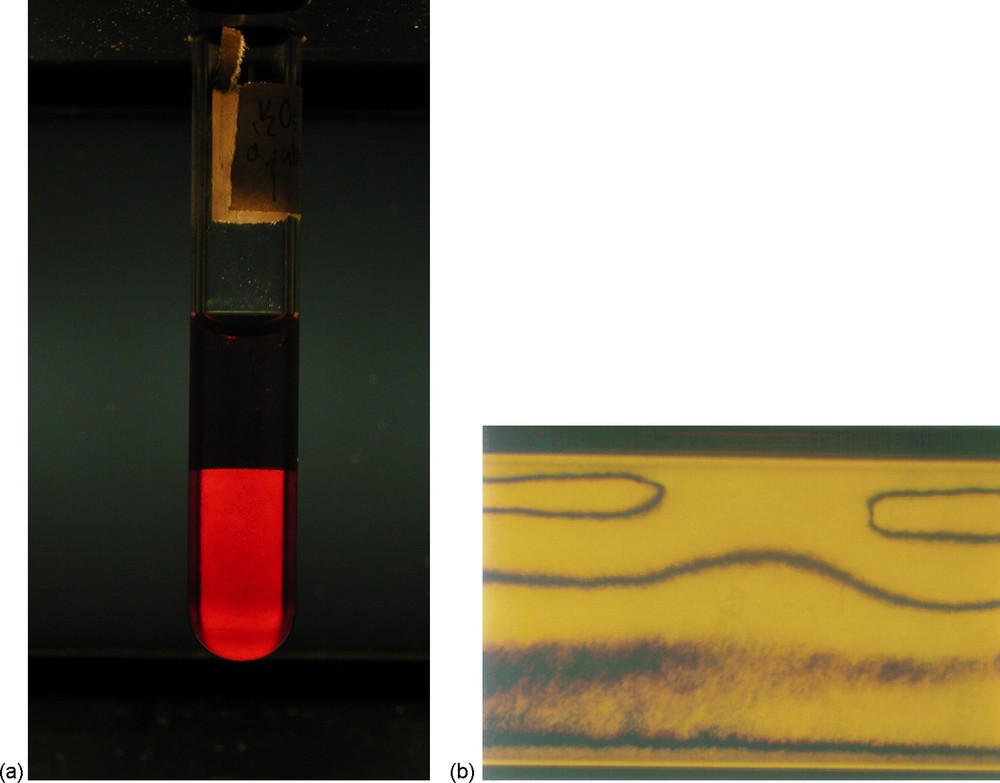
a: photograph of a biphasic V2O5 suspension in a test-tube, viewed between crossed polarizers; the isotropic phase at the top appears dark whereas the nematic phase at the bottom is birefringent; b: V2O5 nematic suspension, in an optical capillary tube observed by polarized light microscopy, showing a typical nematic texture.
At volume fractions larger then ϕN = 0.7%, a pure nematic phase forms (i.e. with no isotropic phase left) and shows typical nematic textures in polarized light microscopy (Fig. 2b). In contrast, sols prepared at volume fractions lower than ϕI = 0.5% do not display any birefringence at rest, which shows that they are in the isotropic phase. However, when submitted to shear flow, they display an appreciable birefringence. This anisotropy, which is confirmed by in situ SAXS experiments under shear, proves that the ribbons are still intact in dilute sols. Within experimental accuracy, the phase borders (ϕN and ϕI) are not sensitive to temperature and, in a first good approximation, the system can be considered as athermal (in a range 10–80 C).
4 Alignment of V2O5 nematic suspensions in magnetic and electric fields
The nematic sols of volume fractions ranging from 0.7 to 1.5%, are easily aligned by small magnetic fields [20]. This is easily observed by polarized light microscopy observations of flat glass capillaries filled with nematic sol samples and submitted to a transverse magnetic field (Fig. 3). The nematic texture due to topological defects called disclination lines [2] becomes completely uniform as the defects vanish. Once the alignment is completed, the sample is completely dark when the field is parallel to either the polarizer or analyzer directions and shows maximum brightness when the field makes an angle of 45° with these directions. Both planar (nematic axis parallel to the capillary plane) and homeotropic (nematic axis perpendicular to the capillary plane) orientations could be produced by varying the field direction. The alignment kinetics depends on both the magnetic field strength B and the suspensions concentration. For the nematic phase at coexistence with the isotropic phase (i.e. the most dilute nematic phase that can be obtained), of volume fraction 0.7 %, the alignment kinetics can be described by a simple exponential law, with a time constant τ = 360 s for B = 1 T and τ proportional to 1/B2, as classically expected. The birefringence of this suspension could also be measured, Δn = 10–2, a fairly large value for such a dilute system, which illustrates the interest of considering inorganic particles in the field of liquid crystals. Altogether, applying a magnetic field represents a very simple way of controlling the orientation of the V2O5 ribbons in the suspension, even though they are not ferromagnetic, in a completely noninvasive way. Note that the tactoids are also affected by an applied magnetic (or electric) field; they orient their main axis along the field direction. Another way of aligning V2O5 suspensions is by applying them a shear flow, in which case, the ribbons orient their main axis along the flow direction.
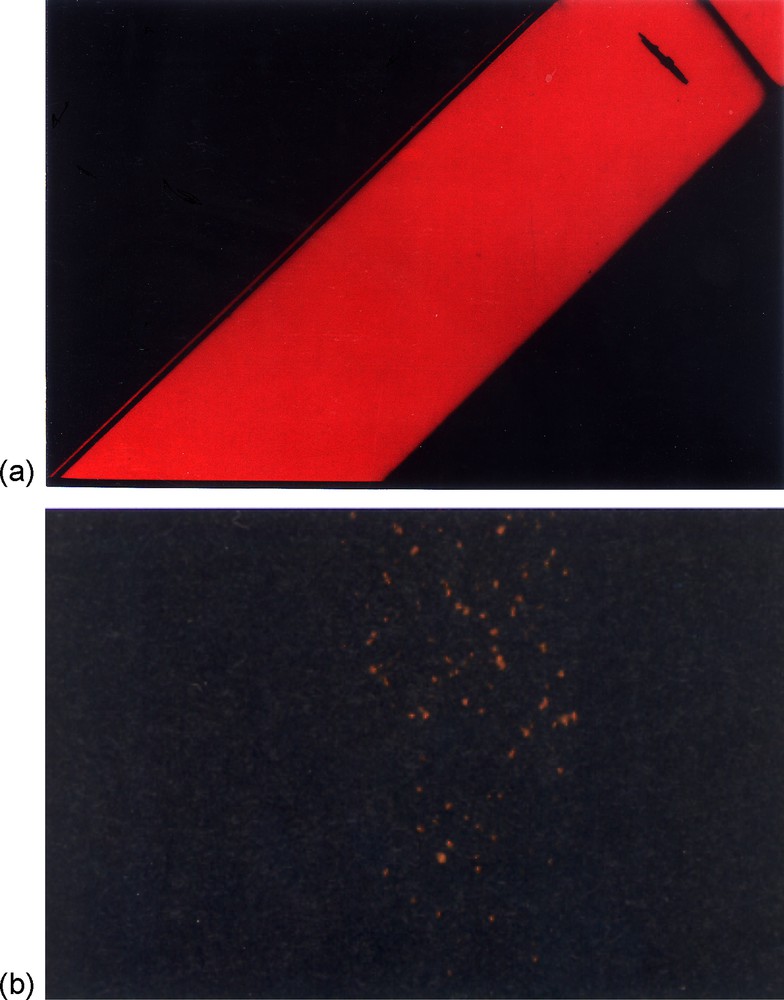
V2O5 nematic suspension aligned in a magnetic field: a: in position of maximum transmission (i.e. nematic director at 45̊ with the polarizer); b: in extinction position (i.e. nematic director parallel to the polarizer).
Single domains of the nematic phase are very well suited for SAXS analysis and give rise to a scattering pattern displayed on Fig. 4. This pattern is obviously anisotropic and since no sharp scattering features, such as Bragg peaks or diffraction lines, are observed, it is typical of a nematic liquid-crystalline phase [7,10]. Moreover, the diffuse scattering peaks visible on the SAXS pattern represent the intersection with the Ewald sphere of a diffuse torus perpendicular to the nematic director that is aligned along the field direction. This diffuse torus arises from the lateral interferences between ribbons in the plane perpendicular to the director and therefore its position in reciprocal space provides the average distance between ribbons.

SAXS pattern of a single domain of V2O5 nematic suspension aligned in a horizontal magnetic field (the white rectangle at the centre is the beamstop).
The most important structural feature of the nematic phase is the nematic order parameter S = <1/2 (3 cos2θ − 1)> where θ is the angle between a ribbon and the director n and where the brackets represent a statistical average. S takes values between 0 for an isotropic phase and 1 for an ideally aligned nematic phase, with no fluctuations. Using well-documented methods [21], S can be extracted from the SAXS pattern and was estimated at S = 0.75 ± 0.05. This fairly high value is quite comparable to the nematic order parameters of conventional organic liquid crystals used in display technology. It is also in very good agreement with the value predicted by the Onsager model of the isotropic/nematic phase transition (see below).
Nematic single domains show a quite complex transient behaviour upon a sudden reorientation of the magnetic field direction. For example, Fig. 5 illustrates the instabilities that occur when a nematic fluid V2O5 suspension, previously aligned in the horizontal direction, is submitted to a magnetic field oriented either along the vertical direction or perpendicular to the figure. Transient instabilities appear, resulting in striped and patterned textures made of domains with different director orientations. Such instabilities are actually typical of liquid-crystalline phases of rod-like particles and have been studied in great detail in the case of suspensions of rigid viruses [22]. The wavelength of the instability can be adjusted between 10 and 100 microns and depends on the sample thickness and magnetic field intensity. This transient phenomenon therefore allows one to micropattern the direction of V2O5 ribbons in a very simple and cheap way.
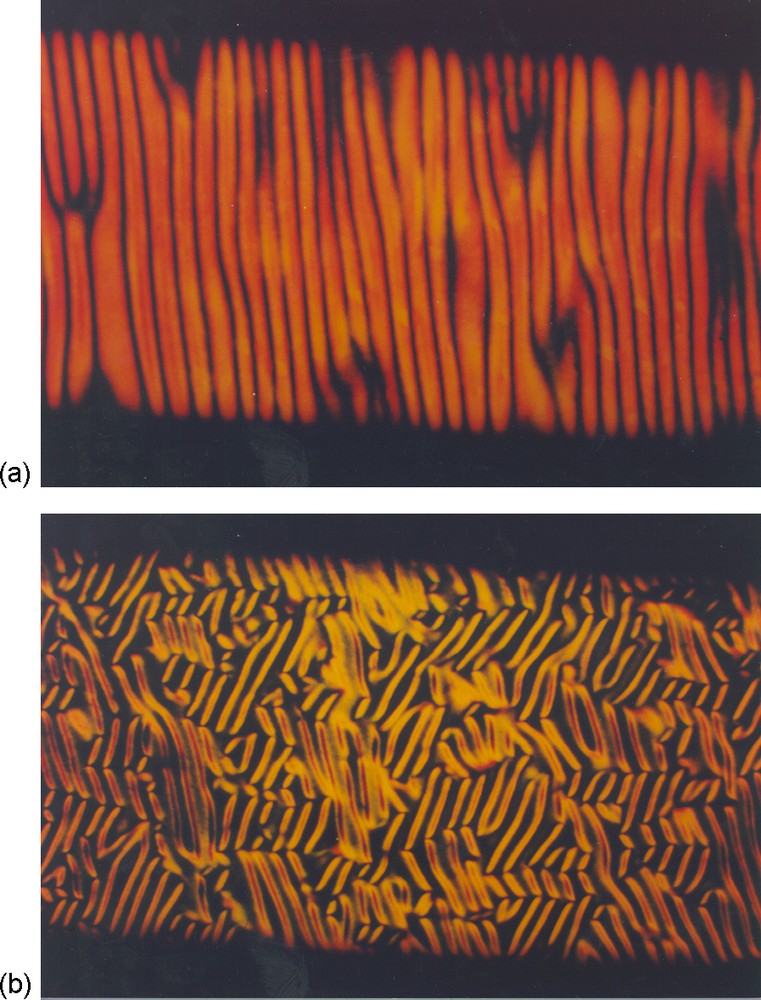
Transient hydrodynamic instabilities observed with a single domain of V2O5 nematic suspension when the magnetic field is suddenly reoriented from the horizontal direction to: a: the vertical direction; b: perpendicular to the figure (polarized light optical microscopy; the capillary is 1 mm wide).
Nowadays, electric fields, rather than magnetic fields, are always used to address liquid-crystal cells for display technology, which immediately suggests examining the influence of an applied voltage to a nematic V2O5 sample. However, the very nature of this liquid crystal makes this experiment difficult. Indeed, the presence of water implies a very large electrical conductivity and electrochemical phenomena can occur at the electrodes. Moreover, H+ ions can easily migrate and create a depolarizing field that can cancel the applied field. In order to avoid such difficulties, high frequency (a few 100 kHz) alternative fields were used and the electrodes were coated with passivating polymer thin films [23]. Furthermore, 10 μm thick cells were filled with the suspensions and the nematic director was aligned parallel to the cell walls by the flow induced upon filling. In the absence of applied field, a nonuniform planar texture was observed that is due to flow-alignment occurring during cell filling. The ribbons lay in the plane of the cell but without any preferential orientation. Then, applying moderate electric fields of 0.5 V/μm on the cells resulted in the complete alignment of the nematic phase in about 10 s, with the nematic director aligned along the field direction, perpendicular to the cell. At this volume fraction and for this 10 μm thickness, a minimum voltage of 2 V (the “Frederiks threshold” [2]) must be applied to the cell in order to achieve alignment. This orientation relaxed to the previous planar one when the field was switched off and a switching behaviour was demonstrated when the field was alternatively switched on and off, with typical time constants of a few tens of seconds. Moreover, the same kind of transient instabilities as those induced by the sudden rotation of a magnetic field were observed with the electric field. The analysis of these phenomena also led to the estimation of combinations of macroscopic physical constants such as the rotational viscosity and the anisotropy of dielectric susceptibility. Moreover, a relatively large field-induced birefringence was also observed in the isotropic phase, with a response time of a fraction of a second. This example demonstrates that mineral liquid crystals could in principle be considered for display applications. However, the response times of these large colloidal objects are much longer than those of usual liquid-crystalline organic small molecules, preventing their use for fast displays.
5 Thermodynamics and phase diagram of vanadium pentoxide suspensions
The onset of the nematic order in vanadium pentoxide suspensions can be discussed in the frame of the Onsager model of the isotropic/nematic phase transition [6,24]. Without going into details, this approach considers the particles as rigid rods interacting only through hardcore repulsions (i.e. excluded volume interactions). Since there is no energy scale in the interaction potential used here, temperature cannot have any influence on the transition. The model is a trade-off between the orientational entropy loss due to the alignment of the rods and a packing entropy gain when the rods become parallel. The model predicts a first-order phase transition (i.e. with phase coexistence) with a large jump of the nematic order parameter from 0 in the isotropic phase to 0.75 in the nematic phase. The volume fractions of the coexisting isotropic and nematic phases are, respectively, ϕI = 3.2 L/D and ϕN = 4.4 L/D where L is the rod length and D the rod diameter. The width of the biphasic gap is therefore predicted to be 4.4/3.2 = 1.38.
The main predictions (first-order transition, biphasic gap of 1.4, athermal character, large-order parameter jump, S = 0.75, at the transition) of the Onsager model are indeed verified with the V2O5 suspensions. In principle, electrostatic interactions should also be considered but they should be partially screened in this case of suspensions at pH around 2–3 where the ionic strength is fairly high. Particle flexibility could also be incorporated in the theory. Altogether, the Onsager model provides a fairly good semiquantitative description of the transition although other important V2O5 ribbon features, such as particle biaxiality and polydispersity, should also be considered in more detail. However, suspensions of rod-like or filamentous viruses are probably better experimental systems to test such statistical physics models [25].
The influence of ionic strength on the phase diagram of V2O5 suspensions has also been examined. The ionic strength cannot be decreased to less than 10−3 M because V2O5 ribbons become unstable at pH larger than 3. Moreover, multivalent ions quickly induce flocculation so that only monovalent ions were considered. Increasing the ionic strength of vanadium pentoxide suspensions by adding salts in large amounts naturally results in the flocculation of this colloid that is electrostatically stabilized. However, adding more modest salt amounts has revealed some more unexpected observations [26]. In contrast with the nematic phase of viruses that turns isotropic upon an ionic strength increase, the isotropic phase of V2O5 suspensions becomes birefringent. The destabilization of the isotropic phase due to salt addition is illustrated by Fig. 6 that depicts the whole phase diagram as a function of volume fraction and NaCl salt concentration. The isotropic/nematic transition line is thus tilted towards lower volume fractions. This observation in fact contradicts the predictions of the Onsager model. The influence of the ionic strength on the sol/gel transition is even more striking as it sharply crosses the biphasic region to reach the isotropic phase. Close inspection of the samples reveals that the two phenomena are intimately related. From a structural point of view, the main consequence of salt addition is the disappearance of the scattering peak arising from lateral interferences among ribbons and a strong increase of scattered intensity at small angles. This is a classical sign of the weakening of the electrostatic repulsive interactions and the onset of attractive interactions. These latter interactions may simply be of the van der Waals type for two reasons:
- • firstly, the Hamaker constant that sets the strength of van der Waals forces is large (H ≈ 5 × 10−20 J) in the case of vanadium pentoxide ribbons dispersed in water because of the large electronic contrast between the particles and the solvent;
- • secondly, the van der Waals forces are enhanced by the one-dimensional character of the particles.
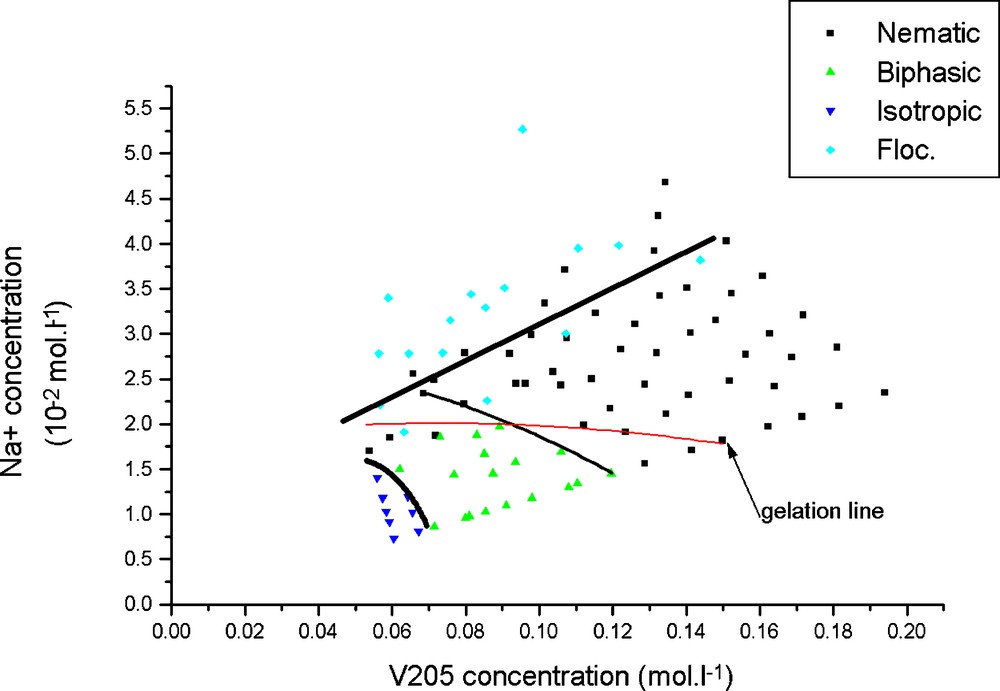
Concentration, ionic strength phase diagram showing the isotropic and nematic phases, the biphasic region in between, the flocculated state and the sol/gel transition line.
The DLVO interaction potential [27] that gathers both the electrostatic repulsions and the strong van der Waals interactions, adapted for ribbon-like particles and using typical values of Hamaker constant and surface electric potential, accounts for the ionic strength at which the crossover from repulsive to attractive interactions occurs. Altogether, the DLVO potential does explain the influence of the ionic strength upon the sol/gel and isotropic/nematic transitions. Consequently, the stability of the nematic phase is either due to the electrostatic repulsions at low ionic strength or to the van der Waals attractions at high ionic strength. The final state of these attractive nematic gels results from a balance between several competing kinetics, namely the gelation, the phase separation and the cooperative nematic alignment kinetics.
The usual nematic phase formed by cylindrical rods of circular section has uniaxial revolution symmetry. In the case of elongated lath-like particles of very anisotropic section, another nematic phase was predicted [28] for which not only the long axes of the particles are all aligned in the same direction but also their widths all point in another perpendicular direction. Because of its biaxial symmetry, this nematic phase is called a biaxial nematic. It currently raises very large interest from the liquid-crystals community because there are only very few examples of this phase [29]. Most theoretical models predict that the stability of the biaxial nematic phase should be maximum for lath-like objects whose dimensions obey the relation w2 ≈ Lt.
Interestingly, vanadium pentoxide ribbons happen to verify this rule of thumb if one considers Lp instead of L. Moreover, the evolution (called swelling law) with volume fraction of the average distance d between ribbons in the plane perpendicular to the nematic director has a complex behaviour: for volume fractions lower than 4%, d varies as 1/ϕ1/2 whereas it varies roughly as 1/ϕ for larger volume fractions. The first regime is the signature of the two-dimensional swelling of a uniaxial nematic of rods whereas the second one is the signature of the one-dimensional swelling of sheets, which is reminiscent of the fact that V2O5 gels behave as intercalation compounds at very high concentration (at very low water content, the swelling is discontinuous because the number of water molecules per V2O5 unit varies in a discrete way). In the dilute regime, V2O5 ribbons can rotate around their long axes but this rotation is probably severely restricted at high volume fraction when the average distance between ribbons is smaller than their width.
Unfortunately, the proper identification of a biaxial nematic phase by structural techniques requires the production of a single domain and the above considerations only strongly hint at the possible existence of such a phase in V2O5 gels. A simple way of creating biaxial alignment is by applying a shear flow that is intrinsically biaxial because the directions of velocity, velocity gradient and vorticity are all different. This can easily be achieved by using a Couette shear cell, which is a device that consists in two concentric cylinders with one that can rotate with respect to the other. In such an experiment, the colloid was poured between the two cylinders and was sheared by the rotating outer cylinder [30]. The organization of the ribbons was probed by sending X-rays either, radially, through the centre of the cell and the (velocity, vorticity) plane was examined or, tangentially, through the gap between the cylinders and the (velocity gradient, vorticity) plane was examined. The combination of these two perpendicular cuts in reciprocal space provides a complete description of the symmetry of the structure.
At volume fractions lower than 4%, whatever the shear rate, the radial configuration led to an anisotropic SAXS pattern. This was expected because the ribbons align with their long axes parallel to the shear flow. However, the tangential configuration only provided an isotropic pattern. Therefore, the nematic phase is clearly uniaxial. In contrast, at volume fractions larger than 4% and at shear rates as low as 100 s−1 applied for a few minutes only, both configurations led to anisotropic SAXS patterns, which demonstrates the biaxial nematic symmetry of the colloid. This biaxial orientation did not relax after hours once the shear flow was stopped. However, a major problem with this system lies in its gel character. Indeed, in this experiment, the biaxial alignment of the ribbons may as well have been induced by the shear and could have been trapped in the gel. Then, the thermodynamic origin of this biaxial gel state still remains an open question.
6 Proton NMR
In general, NMR spectroscopy is a very powerful technique to probe the alignment and dynamics of either the particles or the solvent in a liquid-crystalline suspension of anisotropic particles. Here, deuterium NMR was used to study the behaviour of the solvent in the fluid nematic phase of the suspensions of V2O5 ribbons [31]. For this purpose, water was almost completely replaced by heavy water (D2O) through successive dialyses.
The dominant interaction for a deuterium nucleus is the electric quadrupolar interaction that splits the resonance line into a doublet, which makes this technique very sensitive to molecular orientation and affords the determination of the nematic order parameter. Experiments were performed in a 2 T NMR magnetic field that is large enough to align the nematic phase. Strikingly, the resulting spectrum (Fig. 7) shows a well-resolved quadrupolar doublet, with a splitting of about 100 Hz. This means that quadrupolar interactions are not averaged to zero, which indicates that water molecules undergo anisotropic motions on the NMR timescale (10−4–10−6 s), even at such large dilutions. The line width is the same as that of pure water and is only limited by instrumental resolution. Actually, this spectrum is highly reminiscent of that of an aligned nematic sample [32]. The interpretation of the spectrum is the following: at the NMR time scale, water molecules undergo fast exchange between the bulk and adsorption sites on the ribbons surface. “Average” water molecules are then detected and their behaviour directly reflects the uniaxial symmetry of the nematic phase. The order of magnitude of the splitting implies that adsorbed water molecules are rotationally disordered with respect to the normal to the ribbon surface. The interpretation of the NMR spectrum can be checked by recording the value of the splitting as a function of the angle Ω between the nematic director and the applied magnetic field (before the nematic phase reorients along the field direction). The dependence obtained, Δν(Ω) = (3cos2Z − 1)/2|Δν(0), is precisely that expected for a uniaxial mesophase. Therefore, the phase symmetry deeply affects the dynamics of the solvent molecules.
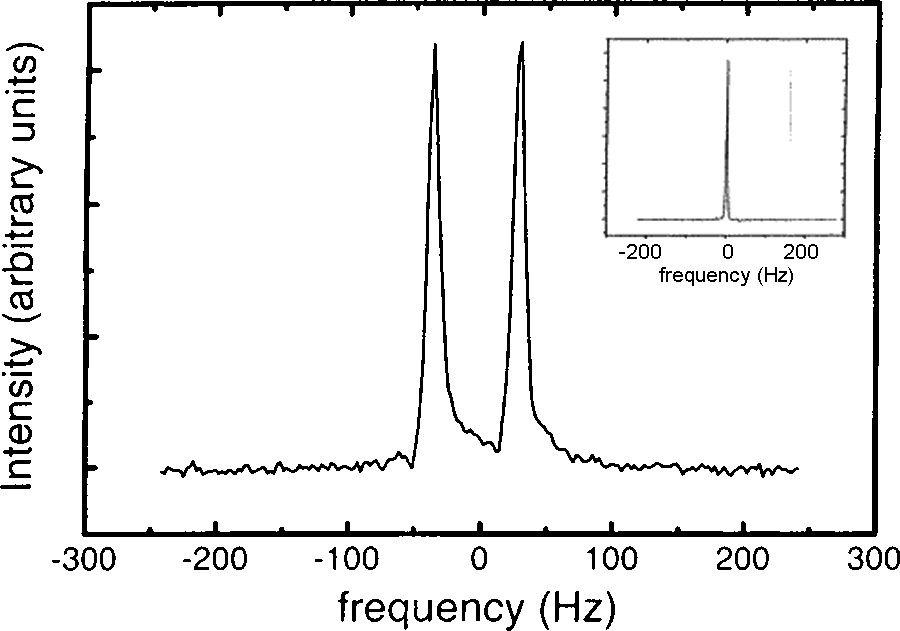
Deuterium NMR spectrum of D2O molecules in an aligned domain of a V2O5 nematic suspension (insert: NMR spectrum in pure D2O for comparison).
Conversely, water molecules can be used to probe the reorientation dynamics of the nematic phase. Indeed, when the sample is suddenly rotated, with respect to the magnetic field, the nematic phase will slowly realign within a characteristic time, typically an hour, determined by a balance between magnetic and viscous torque. Since the acquisition of an NMR spectrum takes typically a second, this reorientation can be followed by recording the evolution with time of the splitting and hence of the angle Ω. TanΩ obeys an exponential law that involves phenomenological constants (the rotational viscosity and the anisotropy of magnetic susceptibility), which agrees with theoretical models and gives experimental information on the mesophase physical properties. However, quite unexpectedly, a huge dependence with temperature of the reorientation time was observed and could be described as a power law with an exponent of −9.5. This strong dependence is in sharp contrast with the athermal character of the phase diagram and is not yet understood.
7 Various other applications of V2O5 gels in “soft matter” and biology
A first application of liquid-crystalline vanadium pentoxide suspensions was their use as an oriented solvent for the determination of the structures of biomolecules in solution by NMR [33]. Indeed, nematic solvents have been recently used for this purpose because their uniaxial symmetry creates residual dipolar couplings that bear information relative to the conformation of the macromolecules of interest [34], over a longer length scale than the couplings usually exploited. As an example, this technique was applied to the case of a particular sugar, a pentasaccharide called LewisX, involved in cellular recognition mechanisms and its conformation could be determined. Of course, in principle, this method could be extended to other biomolecules, such as water-soluble proteins, and, compared to the other organic molecules used for this purpose, it has the following advantages: the concentration of V2O5 is only of order 1%, the liquid-crystalline suspension is very stable, and there is no need for isotope labelling of the protein that can be easily recovered by flocculating the colloid. However, the very acidic pH of the suspension and the strong oxidant character of V2O5 severely limit this application. Since then, another type of mineral liquid crystal was developed and could be used for the same purpose [35].
In a very simple yet spectacular experiment, very light vanadium pentoxide foams were synthesized by reacting hydrogen peroxide with a pasty mixture of V2O5, 1-hexadecylamine, and acetone. Quite small amounts (1 g) of V2O5 were enough to produce very large quantities (2.5 L) of foam [36]. X-ray diffraction experiments with the foam showed the usual (00l) reflections arising from the stacking of the ribbons, which suggests that the local structure of the bubble walls is comparable to that of V2O5 xerogels. However, the 1-hexadecylamine remains trapped, intercalated between the ribbons. This technique of foam production could be applied to other metal oxides.
Another technique of production of vanadium pentoxide foams, which affords better control of bubble size and Plateau border thickness, was more recently reported. Foams were obtained by bubbling perfluorohexane saturated with nitrogen through a porous glass disk into homogeneous aqueous solutions of V2O5 colloidal ribbons and a nonionic surfactant, while taking care of minimizing foam drainage through solution recirculation from bottom to top. The dimensions of the Plateau borders could be adjusted by varying the foam liquid fraction whereas the bubble size was defined by the size of the holes in the porous glass disk. The resulting foam, in a monolith state, could either be used as prepared, with the surfactant trapped within the foam walls, or calcined to remove the surfactant and improve the crystallinity of the walls [37].
An original fibre-drawing technique, first devised in the case of carbon nanotubes [38], was successfully applied to the case of V2O5 ribbons [39]. This technique consists in extruding the material of interest into a rotating beaker filled with a solution of an organic polymer. Here, the organic polymer was poly(vinyl alcohol) and the solvent was water. Long (of order a meter), tough yet flexible, fibres were formed, which even allows the formation of knots. The fibre Young's modulus can reach up to about 15 GPa, comparable to fibres made of carbon nanotubes. The X-ray diffraction spectra of these fibres suggest that their local structure is quite similar to those of V2O5 xerogels. Moreover, polarized light microscopy and SAXS experiments showed that the ribbons are quite well aligned along the fibre main axis, which probably explains these exceptional mechanical properties. The degree of alignment improves when the shear rate developed during extrusion increases. These fibres have demonstrated very good properties as alcohol sensors and it was shown that a single vanadium oxide fibre can detect down to 0.1 ppm of ethanol within 16 s at 40 °C.
Vanadium pentoxide ribbons were also used instead of cylindrical micelles of organic surfactant, in order to template mesostructured silicas [40], in a way similar to the process that leads to the famous MCM and SBA mesoporous silicas [41]. Silica precursors were dissolved in V2O5 aqueous suspensions and their hydrolysis led to silica condensation and hence to solid materials. One of the interests of using V2O5 nematic suspensions is that they can be macroscopically aligned in an external magnetic field, prior to silica condensation. There again, polarized light microscopy together with SAXS experiments demonstrated the anisotropy of the materials thus produced. Then, the ribbons could be etched away by acidic attack, which liberated the porosity of the material. A porous volume of about 200 m2/g was measured by gas adsorption experiments. However, the nematic character of the suspension implies that the porosity is not ordered on a two-dimensional lattice, in contrast with the MCM and SBA materials.
Finally, it should be mentioned here that the electrical transport properties of both a vanadium pentoxide xerogel and a single vanadium pentoxide ribbon could be measured [42]. The current/voltage (I/V) characteristic of the xerogel was almost linear with an estimated conductivity of about 0.1 – 1 S/cm. The electrical conduction in V2O5 xerogels is supposed to proceed via hopping between VV and reduced VIV species, so that this property will of course depend on the degree of reduction and may therefore vary from a sample to another. The (I/V) characteristic of a single ribbon could also be measured by contacting it with electrodes, in both two-probe and four-probe configurations. An ohmic characteristic was observed and the conductivity of a single ribbon could thus be estimated to about 0.5 S/cm at room temperature. Moreover, an activation energy of ≈ 0.1 eV could be inferred from the dependence of the electrical conductivity with temperature. Building up on these results, the same team even succeeded in building an n-doped type field-effect transistor from a small number of individual vanadium pentoxide ribbons [43].
With the current fast development of hybrid organic/inorganic systems in soft matter and complex fluids, there is little doubt that vanadium pentoxide gels will find numerous other new applications. In this respect, these materials have quite appreciable structural and electronic properties such as liquid-crystallinity, both electronic and ionic conductivities, electrochromism, etc. However, they also have drawbacks such as their strong oxidizing power and the very acidic pH of the suspensions. Therefore, any formulation of new and innovative material based on them will most probably involve a delicate formulation step. Nevertheless, controlling this difficult step will certainly prove rewarding.
Acknowledgements
The author is deeply indebted to Professor J. Livage for his friendly help and guidance, to the graduate students who worked on this subject, and to the numerous colleagues who took part in some of the studies described in this review article.