1 Introduction
Contemporaneous research in the organic synthesis focuses on economy [1]. The development of rapid and selective synthetic routes toward focused libraries of functionalized heterocyclic building blocks is so important to both medicinal and organic chemists, and still constitutes a challenge from academic and industrial points of view. In modern organic chemistry, because of economic and ecological increasing pressure, investigations are now directed to discover methods that largely take into account the criterion of sustainable chemistry [2].
Xanthenes are known as an important class of heterocyclic compounds widely used as lecodye, pH-sensitive fluorescent materials for visualization of biomolecules and utilized in laser technologies due to their photochemical and photophysical properties. They have been reported to possess diverse biological and therapeutic properties such as antibacterial, antiviral, antiproliferative, and anti-inflammatory activities [3–7].
Tetrahydroxanthenones are among the most important classes of these compounds due to their distinctive structures and great potential for further transformations [8]. Consequently, the development of novel methods for the synthesis of these heterocyclic compounds has been received considerable interest in both organic and medicinal field. Several methods have been reported for the synthesis of 12-aryl-8,9,10,12-tetrahydrobenzo [α] xanthen-11-ones by the condensation of aldehydes, 2-naphtol and cyclic 1,3-dicarbonyl compounds catalyzed by Sr(OTf)2 [9], NaHSO4.SiO2 [10], H3PW12O2 [11], p-TSA/[bmim]BF4 [12], HBF4/SiO2 [13], InCl3 and/or P2O5 [14], N,N’-dibromo-N,N’-1,2- ethanediyl-bis(p-toluene sulfonamide) [BNBTS] [15], Caro,s acid.SiO2 [16], I2 [17], HClO4/SiO2 [18], and proline triflate [19].
Nitrogen-containing heterocycles, which exhibit extensive biological properties, are abundant in natural compounds [20,21]. Phenazines and quinoxalines are important classes of benzoheterocycles, which are significant in both chemistry and biology [22–24].
Quinoxaline and its derivatives represent one of the most biological active classes of compounds [25], possessing a wide and diverse spectrum of pharmacological properties [26,27], such as anticancer [28], antiviral [29], antibiotic (echinomycin), and anti-inflammatory activities [30,31]. The quinoxaline nucleus is also associated with applications in dyes [32], organic semiconductors [33,34], dehydroannulenes [35], and cavitands [36,37]. These compounds are synthesized using different types of catalysts such as ceric (IV) ammonium nitrate [38], iodine in DMSO [39], sulfamic acid [40], Yb(OTf)3 [41], oxalic acid [42], o-iodoxybenzoic acid [43], H6P2W18O62. 24H2O [44], KHSO4 [45], and CuSO4.5H2O [46]. However, these methods have some disadvantages such as:
- • the need for anhydrous conditions;
- • harsh reaction conditions;
- • the use of expensive reagents;
- • long reaction time;
- • unsatisfactory yields;
- • tedious work-up and requirement of excess of reagents or catalysts;
- • harmful volatile organic solvents;
- • no agreement with the green chemistry protocols.
Moreover, some methods are not efficient when aryl 1,2-diamines with electron-withdrawing substituents are used in the condensation reaction. Therefore, development of an efficient, safe, cheap, and environmentally friendly reagent for the synthesis of quinoxalines and 12-aryl-8,9,10,12-tetrahydrobenzo[α] xanthen-11-one derivatives is desirable.
In recent years, use of green reagents in organic reactions attracted the attention of many organic chemists. This attention can be attributed to the reduction of environmental pollution and the cost of applied methods.
Rice husk, as a thin but abrasive skin in nature, which covers the edible rice kernel, contains cellulose, hemicellulose, lignin, silica, solubles, and moisture. The worldwide annual rice husk output is about 80 million tones and over 97% of the husk is generated in developing countries [47]. In the course of decades, rice husk has found different applications in chemistry and industry. For example, unmodified rice husk has been evaluated for its ability to bind zinc (II) and other metal ions [48,49]. On the other hand, various modifications have been done on rice in order to enhance its sorption capacities for metal ions and other pollutants [50,51]. In addition, both rice husk and rice husk ash are used as potential raw materials in ceramics, cements and silica-based industries [52–54]. To the best of our knowledge and in spite of the above-mentioned applications, rice husk, as an abundant and green reagent, has not been used in organic reactions yet.
2 Experimental
2.1 General
Chemicals were purchased from Fluka, Merck, and Aldrich chemical companies. All yields refer to the isolated products. Determination of the purity of the substrate and monitoring of the reaction were accomplished by thin-layer chromatography (TLC) on a silica-gel polygram SILG/UV 254 plates.
2.2 Instrumentation
The IR spectra were run on a Perkin-Elmer bio-spectrometer. The reaction conversions were measured by GC on a Shimadzu model GC-16A instrument using a 25 m CBPI-S25 (0.32 mm ID, 0.5 _m coating) capillary column. The 1HNMR (300 MHz) and 13CNMR (75 MHz) were run on a Bruker Avance DPX-250 FT-NMR spectrometer (δ in ppm).
Microanalyses were performed on a Perkin-Elmer 240-B microanalyzer. Melting points were recorded on a Büchi B-545 apparatus in open capillary tubes. The characterization of rice husk was obtained using scanning electron microscopy (SEM-Philips XL30) with field emission gun and energy dispersive spectroscopy (EDS). Prior to placing into the microscope, the rice husk particles were coated with gold under vacuum (SCD 005 sputter coater, Bal-Tec, Swiss) and examined at an acceleration voltage of 17 KV.
2.3 Preparation of rice husk (RiH)
Rice sample (named as Hassani) was obtained from Rasht (Guilan Provience) in the north of Iran. Rice husk was obtained from a local mill, washed several times with distilled water to remove any adhering materials and dried at room temperature for 48 h. The dried RiH smashed and sieved (80–170 mesh size), washed with distilled water and dried at 110 °C for 4 h. The main components of the rice husk sample were determined as follow, using the reported methods in the literature [55,56]; silica (14.2%), cellulose (27.4%), hemicelluloses (18.3%), lignin (25.8%), inorganic residue (5.8%), solubles (3.5%) and moisture (5%).
2.4 Infrared spectra (IR) analysis
The infrared spectra of RiH is presented in Fig. 1. In the stretching region, two peaks at 3430 cm−1 and 2965 cm−1 are observed. The peak at about 3400 cm−1 is due to the hydrogen bonded–OH stretching and the peak at about 2900 cm−1 is due to the CH stretching from aliphatic saturated compounds [57].
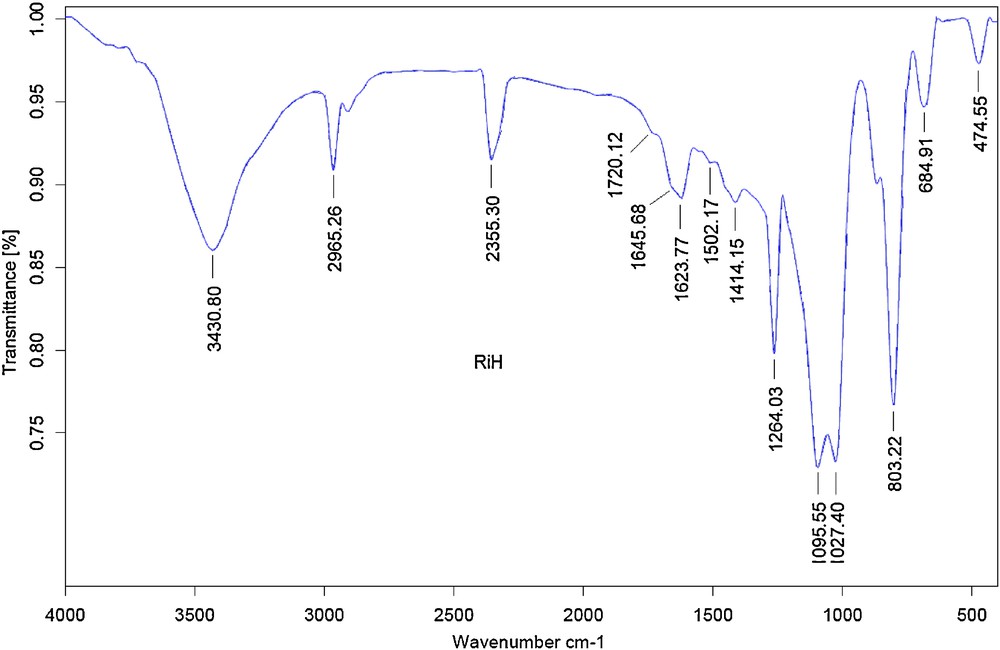
The infrared spectra of RiH.
In the double bond region, a weak peak at 1720 cm−1 is assigned to the C = O stretching of the acetyl and uronic ester groups of hemi cellulose or the ester linkage of carboxylic group of the ferulic and β-coumaric acids of lignin [58]. The peak at 1645 cm−1 was reflected C = O stretching of amide [59]. The peaks at 1623 cm−1 and 1502 cm−1 attributed to the aromatic ring vibrations, which can be only related to lignins [60].
The strong peaks between1025–1100 cm−1 are due to the structural siloxane bond, Si–O–Si. The band at 800–805 and 460–475 cm−1 in all spectra are due to the deformation of Si–O bond [61].
2.5 Scanning electron microscopy analysis
Fiber sizes and shapes are one of the most important factors for composite materials. The effective surface area which may have influence on chemical properties depends on particle size and shape.
The micrographs of the sample are presented in Fig. 2 (a, b) where the layering sequences of phyllosilicate structure can be seen distinctly [62].
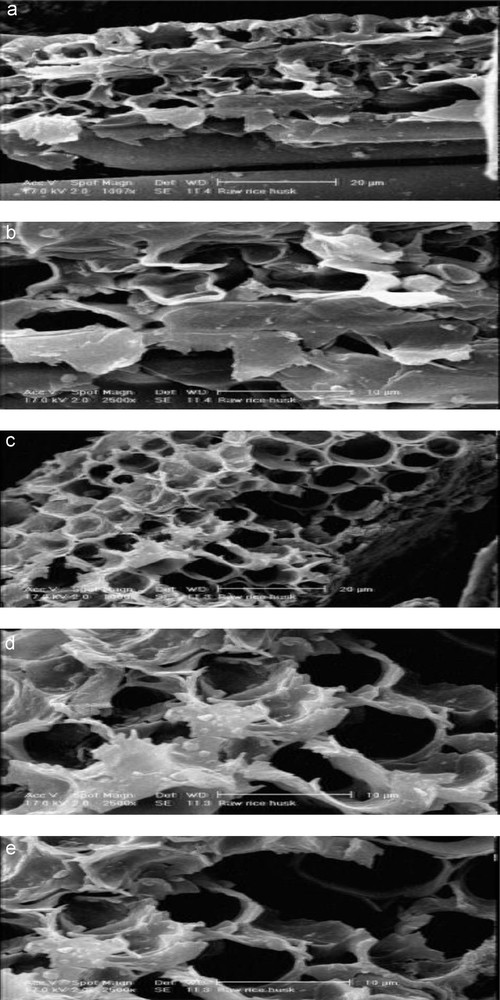
The micrographs of the RiH sample.
Fig. 2 (c–e) shows particle size and shape of rice husk. There are different types of fibre shape observed. Most of the particles are round and angular. Particle size (length) distribution is not the same. All these samples show a structure mainly formed by elongated pores (tubular). The size of the pores are about 3–10 μm.
2.6 X-ray diffraction (XRD) analysis
The XRD pattern of the sample is shown in Fig. 4. As indicated by the featureless diffractometers and the appearance of a diffuse maximum at 2θ = 25.5°, which is typical for amorphous silica [63], it can be concluded that the rice husk (Fig. 3) is completely amorphous and does not show crystalline structure.
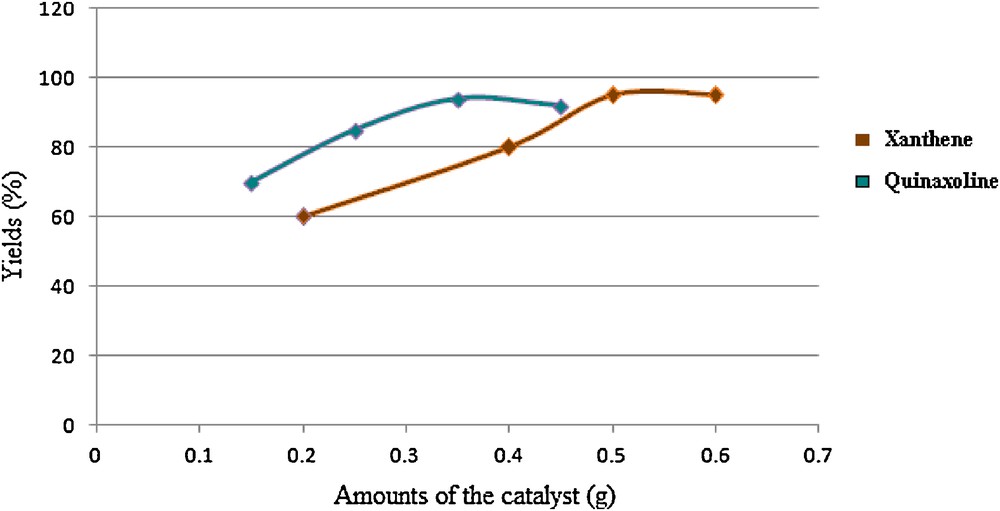
Optimization of the amounts of the catalyst.
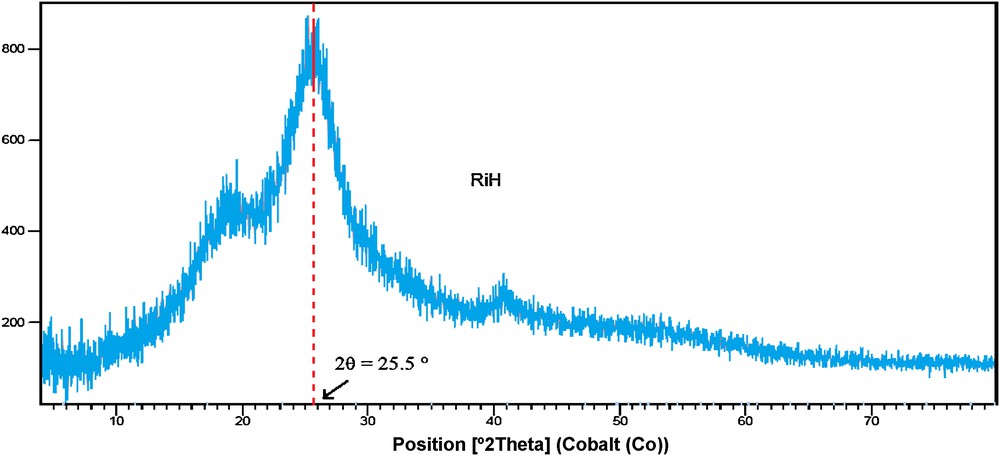
XRD patterns of RiH.
2.7 X-ray fluoroscence (XRF) analysis
X-ray fluorescence, XRF, is the emission of characteristic “secondary” (or fluorescent) X-rays from a material that has been excited by bombarding with high energy X-rays or gamma rays. XRF technology provides one of the simplest, most accurate and most economic and analytic methods for the determination of the chemical composition of many types of materials.
The loss on ignition (LOI) of RiH is determined by heating 3 g of samples at 1000 °C for 1 hour in air to remove the moisture and the co-existing unburned carbon. Table 1 shows the percentage of the elements of RiH with silica as the major element (∼16.54%). Other metallic elements are also presented in RiH as minor elements.
XRF analysis.
Elements | L.O.I | Al2O3 | SiO2 | P2O5 | SO3 | CaO | Cl |
Percentage in RiH | 82.33 | 0.07 | 16.54 | 0.28 | 0.18 | 0.32 | 0.29 |
2.8 General procedure for the synthesis of 12-Aryl -8,9,10,12-tetrahydrobenzo[a]xanthen-11-ones
A mixture of 2-naphthol (1.0 mmol), aldehyde (1.0 mmol), 5,5-dimethyl-1,3-cyclohexadione (1.2 mmol) and RiH (0.5 g) was heated at 90 °C. The reaction was monitored by TLC. After completion of the reaction, the mixture was diluted with acetone (10 mL) and filtered to separate the catalyst. The solvent was removed under reduced pressure. The crude product was purified by recrystalization in ethanol. The physical and spectral data of the known compounds were in agreement with those reported in literature [3,11,13].
2.9 General procedure for the synthesis of quinoxaline derivatives
A mixture of 1,2-dicarbonyl compound (1 mmol), o-phenylenediamine (1 mmol) and RiH (0.35 mol %) in H2O/CH3CN (2 mL/2 mL) was stirred at 50 °C. The progress of the reaction was monitored by TLC. After completion of the reaction, and evaporation of the solvents, the reaction solid product was extracted with Et2O (3 × 15 mL). The catalyst was filtered and reused for further runs. Et2O was evaporated and the solid residue recrystallized with ethanol to afford the pure product. The physical and spectral data of the known compounds were in agreement with those reported in the literature [40,43,58,59].
2.10 The spectral and analytical data for new compounds are given below
12-(4-cyanophenyl)- 9,9-dimethyl-8,9,10,12-tetrahydrobenzo[a]xanthen-11-one (Table 2, entry 8): white solid, mp 196–199 °C; IR (KBr) νmax/cm−1: 3070, 2956, 2931, 2869, 2220, 1651, 1618, 1594, 1515, 1460, 1370, 1220, 1175, 1140, 1020, 838, 802, 740; 1H NMR (400 MHz, CDCl3): d (ppm) 0.97 (s, 3H), 1.15 (s, 3H), 2.25 and 2.37 (AB system, J 16 Hz, 2H), 2.61 (s, 2H), 5.78 (s, 1H), 7.37 (d, J 8.8 Hz, 1H), 7.41–7.49 (m, 6H), 7.84 (t, J 8.4 Hz, 3H); 13C NMR (100 MHz, CDCl3): d (ppm) 27.0, 29.3, 32.3, 35.0, 41.4, 50.8, 110.1, 113.1, 116.1, 117.1, 118.9, 123.2, 125.2, 127.4, 128.6, 129.3, 129.5, 131.1, 131.6, 132.2, 147.8, 149.9, 164.6, 196.8.
Solvent-free synthesis of 9,10,12-tetrahydrobenzo[α] xanthen-11-one derivatives (ATXOs) in the presence of rice husk (RiH).
Entry | Ar | Time (min) | Yield (%)a,b | M. P. (Found) | M. P. (Lit.) | Reference |
1 | C6H5 | 45 | 95 | 150–151 | 150–151 | [13] |
2 | 4-BrC6H5 | 45 | 97 | 181–182 | 186–187 | [13] |
3 | 4-ClC6H5 | 45 | 95 | 187–188 | 187–188 | [13] |
4 | 4-OHC6H5 | 75 | 93 | 218–220 | 223–225 | [13] |
5 | 4-FC6H5 | 40 | 94 | 180–183 | 185–186 | [11] |
6 | 4-NO2C6H5 | 30 | 95 | 190–191 | 174–175 | [13] |
7 | 3-NO2C6H5 | 45 | 94 | 165–167 | 169–170 | [3] |
8 | 4-CNC6H5 | 30 | 98 | 196–199 | – | – |
9 | 4-CH3C6H5 | 50 | 94 | 170–172 | 175–176 | [13] |
10 | 4-(CH3)2CHC6H5 | 50 | 96 | 154–155 | – | – |
11 | 60 | 95 | 209–211 | – | – | |
12 | 40 | 95 | 177–180 | – | – |
a Isolated yield.
b Products were characterized by their physical constants and comparison with authentic samples ([3,11,13]).
12-(4-isoprpylphenyl)-9,9-dimethyl-8,9,10,12-tetrahydrobenzo[a]xanthen-11-one (Table 2, entry 10): white solid, mp 154–155 °C; IR (KBr) νmax/cm−1: 3050, 2950, 2900, 2870, 1642, 1618, 1590, 1504, 1460, 1368, 1220, 1140, 1020, 830, 815, 740; 1H NMR (400 MHz, CDCl3): d (ppm) 1.01 (s, 3H), 1.14 (s, 6H), 1.16 (s, 3H), 2.23 and 2.30 (AB system, J 30 Hz, 2H), 2.48–2.59 (d, J 45 Hz, 2H), 2.77 (t, 6.8 Hz,1H), 5.70 (s, 1H), 7.03 (d, J 7.2 Hz, 1H), 7.19–7.46(m, 5H), 7.78 (t, J 10 Hz, 3H), 8.05 (d, J 8 Hz, 1H); 13C NMR (100 MHz, CDCl3): d (ppm) 23.8, 23.9, 23.9, 27.4, 27.5, 29.2, 32.2, 32.3, 34.2, 40.9, 41.4, 50.8, 50.9, 114.5, 117.1, 118.0, 123.8, 124.8, 126.1, 126.3, 126.9, 128.1, 128.2, 128.3, 128.7, 131.5, 142.1, 146.5, 147.7, 163.9, 197.0.
12-(2-naphtyl)- 9,9-dimethyl-8,9,10,12-tetrahydrobenzo[a]xanthen-11-one (Table 2, entry 11): white solid, mp 196–199 °C; IR (KBr) νmax/cm−1: 3070, 2956, 2931, 2869, 2220, 1651, 1618, 1594, 1515, 1460, 1370, 1220, 1175, 1140, 1020, 838, 802, 740; 1H NMR (400 MHz, CDCl3): d (ppm) 0.96 (s, 3H), 1.14 (s, 3H), 2.32 and 2.36 (AB system, J 16.4 Hz, 2H), 2.53 and 2.62 (AB system, J 35.6 Hz, 2H), 5.92 (s, 1H), 7.35–7.44 (m, 5H), 7.50 (d, J 8.4 Hz, 1H), 7.67–7.86(m, 6H),8.08 (d, J 8 Hz, 1H); 13C NMR (100 MHz, CDCl3): d (ppm) 27.2, 29.3, 32.3, 34.9, 41.5, 50.9, 114.1, 117.1, 117.6, 123.7, 124.9, 125.4, 125.7, 126.7, 127.1, 127.2, 127.4, 128.0, 128.4, 129.0, 131.4, 131.5, 132.1, 133.3, 142.1, 147.8, 147.8, 164.0, 196.9.
12-(flournyl2-carbaldehyde)-9,9-dimethyl-8,9,10,12-tetrahydrobenzo [a] xanthen-11-one (Table 2, entry 12): white solid, mp 178–180 °C; IR (KBr) νmax/cm−1: 3070, 2956, 2931, 2869, 2220, 1651, 1618, 1594, 1515, 1460, 1370, 1220, 1175, 1140, 1020, 838, 802, 740; 1H NMR (400 MHz, CDCl3): d (ppm) 0.98 (s, 3H), 1.14 (s, 3H), 2.25 and 2.29 (AB system, J 16 Hz, 2H), 2.52 and 2.62 (AB system, J 40 Hz, 2H), 3.79 and 3.86 (AB system, J 28.8 Hz, 2H), 5.81 (s, 1H), 7.23–8.08 (m,13H); 13C NMR (100 MHz, CDCl3): d (ppm) 27.2, 27.3, 29.3, 31.9, 32.2, 32.3, 34.8, 36.9, 40.9, 41.5, 50.8, 50.9, 114.4, 115.8, 116.1, 117.1, 117.9, 119.5, 119.60, 119.63, 123.7, 124.8, 124.9, 125.1, 125.6, 126.2, 126.3, 126.7, 127.0, 127.2, 128.4, 128.8, 131.4, 131.5, 139.9, 141.5, 143.4, 143.6, 147.8,164.0, 196.9.
3 Results and discussion
In recent years, investigation on the application of silica-based reagents in the promotion of the synthesis of heterocyclic compounds became an important part of our ongoing research program [64,65]. In continuation of these studies, and based on the morphology and components of the rice husk sample, as a source of amorphous silica [66], we were interested to investigate the applicability of this reagent, as a source of amorphous silica, in the promotion of the synthesis of 12-aryl -8,9,10,12-tetrahydrobenzo[a]xanthen-11-one derivatives and quinoxaline.
First of all and in an optimized procedure, with respect to the solvent, catalyst concentration, temperature, and time, the condensation of 4-chlorobenzaldehyde (1 mmol), β-naphthol (1 mmol), and dimedone (1.2 mmol) was investigated in the presence of RiH as the catalyst. Our investigations clarified that the best result can be obtained in the absence of solvent at 90 °C using 0.5 g of RiH (Table 2, entry 3, Fig. 4 and Scheme 1). After optimization of the reaction conditions and in order to show the applicability of the proposed method, different types of aldehydes were subjected to the same reaction and the results are presented in Table 2.

Synthesis of 9,10,12-tetrahydrobenzo[α] xanthen-11-one derivatives (ATXOs) in the presence of rice husk (RiH).
It is noteworthy that the electron property of the group, on aromatic ring of aldehydes have a delicate effect on the yield of the product and reaction time. As shown in Table 2, aromatic aldehydes containing electron-withdrawing groups showed relatively similar reactivity to those containing electron-donating groups. Because of the formation of unidentified products the method is not suitable for the synthesis of ATXOs from aliphatic aldehydes. It should be noted that when other phenols such as 3,5-dimethylphenol and 3-methoxyphenol are used instead of 2-naphthol the reaction did not proceeded at all and the starting materials are recovered intact even after prolonged reaction times in the absence of solvent and/or in refluxing ethanol.
In order to show the merit of this method, Table 3 compares the results obtained from the synthesis of ATXO derivative of 4-chlorobenzaldehyde by our method with some of those reported in literature.
Comparison of the results obtained from the synthesis of 9,10,12-tetrahydrobenzo[α] xanthen-11-one (ATXO) derivative of 4-chlorobenzaldehyde in the presence of various catalysts.
Entry | Catalyst | Time (min) | Yield (%)a | Reference |
1 | Sr(OTf)2 | 300 | 88 | [9] |
2 | Dodecatungstophosphoric Acid(PWA) | 60 | 92 | [11] |
3 | HClO4-SiO2 | 66 | 95 | [18] |
4 | Proline Triflate 10 mol% | 300 | 76 | [19] |
5 | RiH | 45 | 95 | This work |
a Isolated yields.
The probable mechanism of the reaction is shown in Scheme 2.
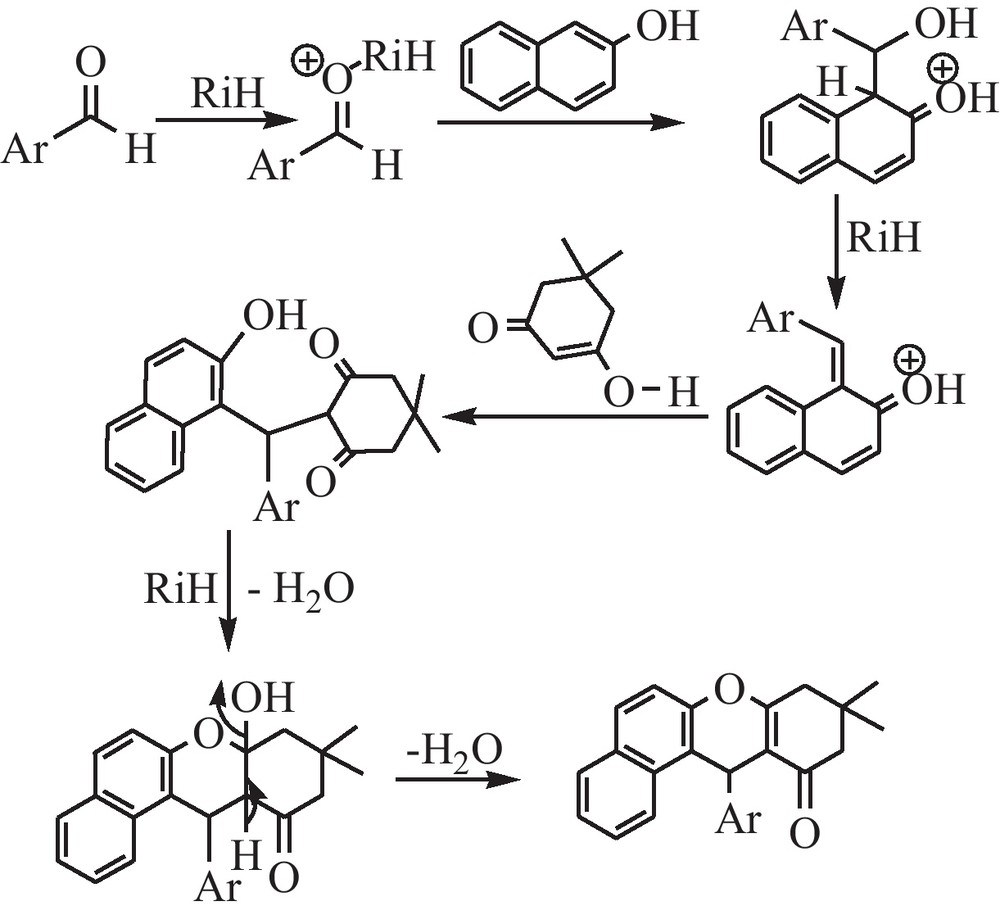
Proposed mechanistic pathway for the formation of 9,10,12-tetrahydrobenzo[α] xanthen-11-one derivatives (ATXOs) under rice husk (RiH) catalysis.
After this investigation, we were interested to study the applicability of RiH in the promotion of the synthesis of quinoxaline derivatives via the condensation of 1,2-diamines with α-diketones. In this study and in order to optimize the reaction conditions, the condensation of benzene-1,2-diamine and benzil was investigated in the presence of RiH. We have found that the best results can be obtained in a mixture of H2O and CH3CN at 50 °C using 0.35 g of RiH (Table 4, entry 1, Fig. 4 and Scheme 3).
Synthesis of quinoxaline derivatives in the presence of rice husk (RiH).
Entry | Product | Time (min) | Yield (%)a,b | M. P. (Found) | M. P. (Lit.) | Reference |
1 | 15 | 94 | 123–125 | 128–129 | 43 | |
2 | 45 | 91 | 187–190 | 193–194 | 43 | |
3 | 60 | 90 | 166–167 | 167–169 | 40 | |
4 | 40 | 94 | 146–148 | 151–152.5 | 43 | |
5 | 90 | 88 | 189–192 | 192–194 | 43 | |
6 | 10 | 96 | 235–237 | 238–240 | 67 | |
7 | 30 | 91 | 323–324 | > 300 | 68 | |
8 | 20 | 92 | 216–219 | 223–225 | 67 |
a Isolated yield.
b Products were characterized by their physical constants and comparison with authentic samples ([40,43,67,68]).

Synthesis of the quinoxaline derivatives.
In the case of quinoxaline formation reaction, with optimal conditions in hand, the reaction of various 1,2-diketones and with 1,2-diamines was examined to explore the scope of the reaction and the results are summarized in Table 4. It was observed that electron-donating groups associated with aromatic 1, 2-diketone decreased slightly the product yields and also increased the time of the reaction (Table 4, entries 4 and 5). Moreover, aromatic ring of aryl 1,2-diamines with electron-withdrawing substituents increased the reaction times (Table 4, entries 2, 5 and 7).
In order to show the merit of the presented method in the synthesis of quinoxalines, we have compared the obtained results from the synthesis of 6-Nitro-2,3-diphenylquinoxaline catalyzed by RiH with some of those reported in literature (Table 5).
Comparison of the results obtained from the synthesis of 6-nitro-2,3-diphenylquinoxaline in the presence of various catalysts.
Entry | Catalyst | Temperature (°C) | Time (min) | Yield (%)a | Reference |
1 | Ultrasound | r. t. | 60 | 37 | [69] |
2 | FeCl3 | 100 | 100 | 41 | [70] |
3 | NiCl2 | 110 | 100 | 52 | [70] |
4 | TiO2–SO4 2− | r. t. | 120 | 25 | [71] |
5 | RiH | 50 | 45 | 91 | This work |
a Isolated yields.
The plausible mechanism is that the α-dicarbonyl compound is first activated by RiH to afford (I). 1,2-Diamine attacks I which in turn converts to the final product and releases RiH for the next catalytic cycle (Scheme 4).
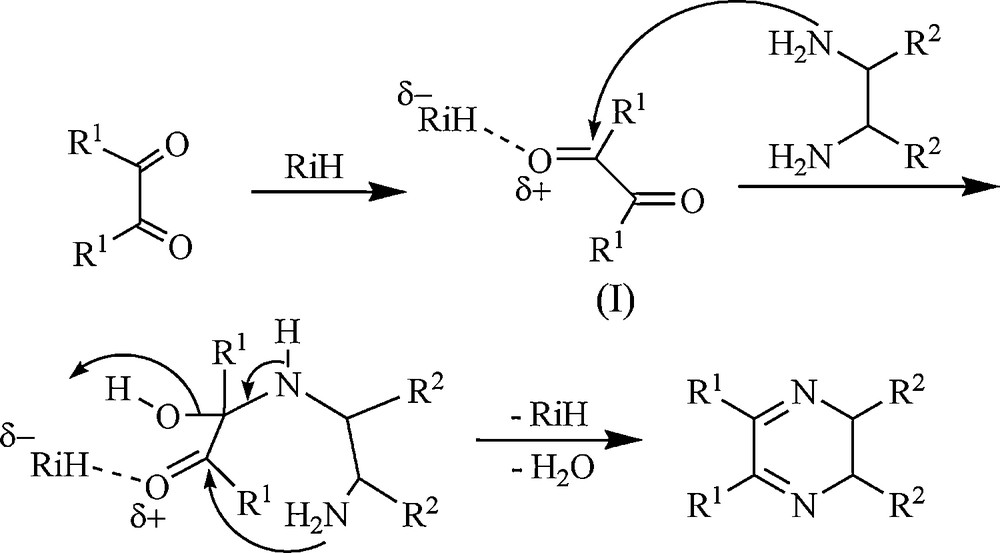
Proposed mechanistic pathway for the formation of quinoxaline derivatives under rice husk (RiH) catalysis.
After performing one reaction under the conditions described in both above experiments, the catalyst was recovered by filtration, washed with acetone (10 mL), and dried at 100 °C and tested for its activity in subsequent run and fresh catalyst was not added. It was seen that the catalyst displayed very good reusability (Fig. 5).
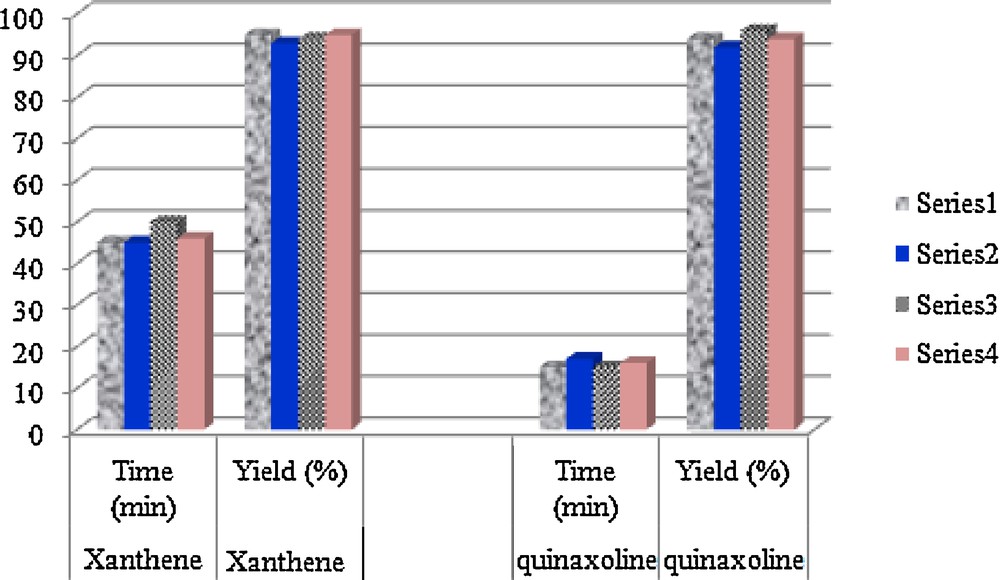
Reusability of RiH in the synthesis of 12-(4-chlorophenyl)-9,10-dihydro-9,9-dimethyl-8H-benzo[a]xanthen-11(12H)-one (Table 2, entry 3), and 2,3-diphenylquinoxaline (Table 4, entry 1). Horizontal axe is usability times of the catalyst and vertical axe is the isolated yield (%) of the product.
4 Conclusions
In conclusion, we have developed a simple, efficient and green process for the synthesis of 12-aryl-8,9,10,12-tetrahydro-benzo[a]xanthen-11-one and quinoxaline derivatives, catalyzed by rice husk (RiH) under mild conditions. The simple experimental procedure, short reaction times, good yields and cheapness, availability and reusability of the catalyst are the worthy advantages of the present method. To the best of our knowledge this article is the first report on the application of rice husk in the promotion of the organic reactions. Further work to explore this novel catalyst in other organic transformations is in progress.
Acknowledgments
We are thankful to the University of Guilan Research Council for the partial support of this work.