1 Introduction
For more than a century, acridine derivatives have been known and firstly used as pigments and dyes in various chemical industries [1]. 1,8-Dioxo-decahydroacridines and their derivatives have been widely studied as polyfunctionalized 1,4-dihydropyridines from a variety of viewpoints such as biological activities [2,3], synthesis [4,5], physicochemical properties [6,7], and structural requirements [8]. They have been explored as calcium channel blockers and used for the treatment of defibrillation and hypertension [9]. In addition, acridine derivatives have been used for the preparation of labeled medicinal conjugates such as nucleic acids, peptides, and proteins that exhibit antitumor and DNA-binding properties [10–12]. These compounds were also found to act as laser dyes and possess many important photophysical and electrochemical properties [13]. Nucleic acids coupled with acridine dyes have received increasing interest as mutagens in microorganisms [14]. The protozoal infections caused by plasmodium microorganisms have also been treated by the acridine derivatives [15]. For many years, substituted acridines have been used as antimalarials and several of them have exhibited excellent results in the chemotherapy of cancer [16]. They have attracted attention due to their properties similar to those of 1,4-dihydropyridines, which are structurally comparable to biologically important compounds such as NADH and NADPH [17].
These compounds have been generally synthesized by a three-component cyclocondensation of dimedone, aromatic aldehydes and different nitrogen sources like urea [18], methyl amine [19], and different anilines or ammonium acetate [20] via traditional heating in organic solvents in the presence of several catalysts such as triethylbenzylammonium chloride [21], amberlyst-15 [22], proline [23], B(C6F5)3 [24], NH4Cl or Zn(OAc)2.2H2O or l-proline [25], p-dodecylbenzenesulfonic acid (DBSA) [26], silica-bonded S-sulfonic acid [27], MCM-41–SO3H [28], and sulfonic acid-functionalized silica [29], under microwave irradiation [30–33], and using ionic liquids such as 1-methylimidazolium trifluoroacetate ([Hmim]TFA) [34] or Brønsted acidic imidazolium salts containing perfluoroalkyl tails [35]. The synthesis of these compounds by the classical Hantzsch's procedure [20] or reactions of aldoximes with dimedone under microwave irradiation have also been reported [36].
However, each one of these cases have their own advantages, but some of them often suffer from one or more disadvantages such as low yields, prolonged reaction time, toxic organic solvents, harsh reaction conditions, tedious work-up processes, and expensive catalyst.
Therefore, the development of efficient, simple, environmentally friendly, and high-yielding methods under mild conditions using new catalysts for the synthesis of acridine-1,8-diones is still in demand.
The multicomponent reactions (MCRs) have been proved to be powerful and efficient bond-forming tools in organic, medicinal and combinatorial chemistry from the green chemistry point of view [37]. The multicomponent coupling reactions are emerging as a useful source for building-up complex molecules with maximum simplicity and several levels of structural diversity. Also, these strategies are atom economic in nature, very flexible, and proceed through a sequence of reaction equilibrium yielding the final product.
Along with other reaction parameters, the nature of the catalyst plays a significant role in determining selectivity, yield, and general applicability. Thus, synthesis and application of a mild, reusable, inexpensive catalyst for MCRs remains an issue of interest.
Replacement of conventional Brønsted and Lewis acid catalysts with environmentally benign and reusable solid heterogeneous catalyst is an active area of current research. Solid acid catalysts as compared with liquid acid ones have some advantages such as ease of product separation, recycling of the catalyst and environmental acceptability [38].
In this regard and in continuation of our interest on application of new nanostructure heterogeneous catalysts in organic reactions [39,40], herein we wish to report a simple, mild and eco-friendly method for the synthesis of hexahydroacridine-1,8-diones via a one-pot three-component condensation of 1,3-cyclohexanedione derivatives, aldehydes and ammonium acetate or aniline, using nanorod vanadatesulfuric acid (VSA NRs) as a novel solid acid catalyst with high catalytic activity and reusability in very short reaction times under solvent-free conditions (Scheme 1).
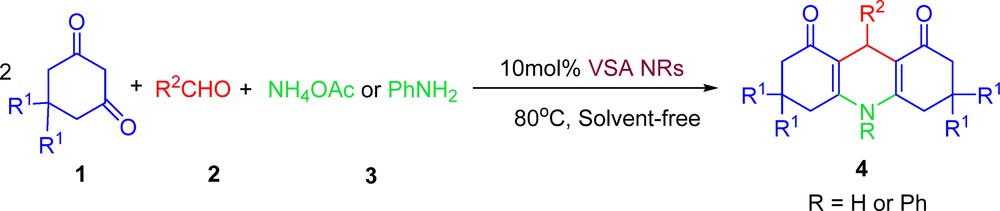
(Color online.) Catalytic synthesis of hexahydroacridine-1,8-diones using nanorod vanadatesulfuric acid.
2 Experimental
All chemicals were purchased from Merck, Fluka and Aldrich chemical companies. X-ray diffraction analysis was carried out using a D8 Advance, Bruker X-ray diffractometer using Cu Kα radiation (λ = 1.5406 Å). Transmission electron microscopy was implemented using a Philips, CM-10 TEM instrument operated at 100 kV. Melting points were determined using a Barnstead Electrothermal (BI 9300) apparatus and are uncorrected. IR spectra were obtained using a FT-IR JASCO-680 spectrometer instrument. NMR spectra were recorded by a Bruker 400 MHz Ultrashield spectrometer at 400 MHz (1H) and 125 MHz (13C) using DMSO-d6 as the solvent, with TMS as the internal standard.
2.1 Preparation of vanadatesulfuric acid
Anhydrous sodium metavanadate was prepared by drying sodium metavanadate monohydrate (NaVO3. H2O, MW = 139.94) in an oven at 250 °C for 4 h. To a mixture of chlorosulfonic acid (0.1 mol, 11.6 g, 7.7 mL) and dry CHCl3 (25 mL) in a 250-mL round-bottom flask in an ice-bath, anhydrous sodium metavanadate (0.1 mol, 12.2 g) was gradually added under rigorous stirring. After the completion of the addition, the reaction mixture was shaken for 1 h. Then, 50 mL of cold water were added to the reaction mixture, which was stirred for another 10 min. The mixture was filtered and a dark red solid of vanadatesulfuric acid, 16.3 g (91%), Mp 256 °C (dec.) was obtained. Characteristic IR bands (KBr, cm−1): 3540–3300 (OH, bs), 1640 (OH, m), 1250–1140 (SO, bs), 1050 (SO, m), 960 (VO, m), 840 (VO, m), 630 (V–O, m) [40].
2.2 General procedure for the preparation of hexahydroacridine-1,8-diones
In a round-bottom flask, the aldehyde (1 mmol), 1,3-cyclohexanedione derivatives (2 mmol), ammonium acetate or aniline (1 mmol) and VSA (10 mol%) were mixed thoroughly. The flask was heated at 80 °C with concomitant stirring. After completion of the reaction monitored by TLC (eluent: EtOAc:n-hexane, 1:3), chloroform (10 mL) was added and then the solid catalyst was filtered and separated. The solvent was evaporated and the crude products were recrystallized from ethanol to obtain the pure products in 80–93% yields based on the starting aldehyde (Table 2). The products were characterized by IR, 1H NMR, and 13C NMR through comparison of their melting points with those reported in the literature. The physical and spectral data of some selected products were found as following.
Catalytic activity evaluation and effect of temperature for the synthesis of hexahydroacridine-1,8-dionesa.
Catalytic activity | Effect of temperature | ||||||
Entry | VSA NRs | Time (min) | Yieldb (%) | Entry | Temp. (°C) | Time (min) | Yieldb (%) |
1 | 0 | 180 | Trace | 1 | 25 | 170 | – |
2 | 5 | 35 | 70 | 2 | 40 | 90 | 58 |
3 | 7 | 30 | 80 | 3 | 60 | 45 | 73 |
4 | 10 | 20 | 93 | 4 | 80 | 20 | 93 |
5 | 15 | 20 | 90 | 5 | 100 | 25 | 85 |
a Reaction conditions: dimedone (2 mmol), benzaldehyde (1 mmol), ammonium acetate (1 mmol), and VSA in mol%.
b Isolated yields.
2.2.1 9-(2,4-dichlorophenyl)-3,4,6,7,9,10-hexahydroacridine-1,8(2H,5H)-dione (4c)
White solid; yield: 90%; M.p: 313–314 °C [41]; Rf = 0.53 (n-hexane:ethyl acetate = 3:1); IR (KBr): 3272, 3070, 2953, 1643, 1609, 1485, 1365, 1222, 1136, 1022, 857, 737, 572 cm−1; 1H NMR (400 MHz, DMSO-d6) δ(ppm): 1.77–2.23 (m, 12H, 6CH2), 5.07 (s, 1H, CH), 7.21–7.30 (m, 3H, Ar), 9.50 (s, 1H, NH); 13C NMR (100 MHz, DMSO-d6) δ(ppm): 20.72, 26.29, 32.68, 36.68, 111.60, 126.54, 128.08, 130.44, 133.12, 133.29, 143.66, 151.65, 194.41; Anal. calcd. for C19H17Cl2NO2: C, 63.00; H, 4.73; Cl, 19.57; N, 3.87; O, 8.83; found: C 63.10, H 4.80, N 3.79.
2.2.2 9-(2,6-dichlorophenyl)-3,3,6,6-tetramethyl-3,4,6,7,9,10-hexahydroacridine-1,8(2H,5H)-dione (4j)
White solid; yield: 86%; M.p: 301–302 °C; Rf = 0.46 (n-hexane:ethyl acetate = 3:1); IR (KBr): 3282, 3070, 2955, 1663, 1611, 1561, 1484, 1376, 1356, 1225, 1145, 1026, 848, 778 cm−1; 1H NMR (400 MHz, DMSO-d6) δ(ppm): 0.92 (s, 6H, 2CH3), 1.00 (s, 6H, 2CH3), 1.90 (d, 2H, J = 16.4 Hz, CH2), 2.12 (d, 2H, J = 16.4 Hz, CH2), 2.21 (d, 2H, J = 17.2 Hz, CH2), 2.40 (d, 2H, J = 17.2 Hz, CH2), 5.54 (s, 1H, CH), 7.05 (t, 1H, J = 8.0 Hz, Ar), 7.2 (dd, 1H, J = 7.2 Hz, J = 0.8 Hz, Ar), 7.27 (dd, 1H, J = 7.2 Hz, J = 1.2 Hz, Ar), 9.35 (s, 1H, NH); 13C NMR (100 MHz, DMSO-d6) δ(ppm): 26.46, 26.57, 28.69, 29.03, 29.62, 31.46, 31.61, 32.91, 50.11, 50.31, 108.73, 127.79, 129.27, 133.46, 136.31, 150.87, 194.02; Anal. calcd. for C23H25Cl2NO2: C, 66.03; H, 6.02; Cl, 16.95; N, 3.35; O, 7.65; found: C 66.12, H 6.10, N 3.30.
2.2.3 9-(3,4-dimethoxyphenyl)-3,3,6,6-tetramethyl-3,4,6,7,9,10-hexahydroacridine-1,8(2H,5H)-dione (4k)
Yellow solid; yield: 85%; M.p: 287–290 °C [42]; Rf = 0.56 (n-hexane:ethyl acetate = 3:1); IR (KBr): 3280, 3072, 2956, 1660, 1611, 1561, 1488, 1376, 1356, 1225, 1145, 1027, 848, 778 cm−1; 1H NMR (400 MHz, DMSO-d6) δ(ppm): 0.89 (s, 6H, 2CH3), 1.01 (s, 6H, 2CH3), 2.00 (d, 4H, J = 16.0 Hz, CH2), 2.18 (d, 2H, J = 16.0 Hz, CH2), 2.31 (d, 4H, J = 17.2 Hz, CH2), 2.45 (d, 2H, J = 17.2 Hz, CH2), 3.64 (s. 3H, OCH3), 3.66 (s, 3H, OCH3), 4.77 (s, 1H, CH), 6.64 (dd, 1H, J = 6.4 Hz, J = 2.0 Hz, Ar), 6.75 (t, 2H, J = 2.0 Hz, Ar), 9.26 (s, 1H, NH); 13C NMR (100 MHz, DMSO-d6) δ(ppm): 26.34, 27.77, 29.13, 32.03, 32.09, 32.21, 46.96, 50.23, 53.38, 55.25, 55.30, 55.42, 111.54, 115.24, 119.43, 130.97, 139.87, 146.69, 147.85, 149.11, 197.06; Anal. calcd. for C25H31NO4: C, 73.32; H, 7.63; N, 3.42; O, 15.63; found: C 73.41, H 7.70, N 3.38.
2.2.4 9-propyl-3,3,6,6-tetramethyl-3,4,6,7,9,10-hexahydroacridine-1,8(2H,5H)-dione (4l)
White solid; yield: 80%; M.p: 255-257 °C [43]; Rf = 0.48 (n-hexane:ethyl acetate = 3:1); IR (KBr): 3259, 3067, 2954, 1640, 1597, 1485, 1381, 1234, 1141, 1005, 887, 560 cm−1; 1H NMR (400 MHz, DMSO-d6) δ(ppm): 0.75 (t, 3H, J = 7.2 Hz, CH3), 1.01 (s, 6H, 2CH3), 1.02 (s, 6H, 2CH3), 1.14–1.22 (m, 4H, CH2), 2.08 (dd, 4H, J = 16.0, J = 8.4 Hz, 2CH2), 2.30 (dd, 4H, J = 17.2, J = 8.2 Hz, 2CH2), 3.84 (t, 1H, J = 8.2 Hz, CH), 9.03 (s, 1H, NH); 13C NMR (100 MHz, DMSO-d6) δ(ppm): 14.37, 18.10, 26.09, 26.41, 28.03, 29.36, 31.93, 37.43, 50.36, 110.59, 150.33, 194.78; Anal. Calcd. for C20H29NO2: C, 76.15; H, 9.27; N, 4.44; O, 10.14; found: C 76.25, H 9.34, N 4.37.
3 Results and discussion
We found that anhydrous sodium metavanadate reacts with chlorosulfonic acid (1:1 mole ratio) to give nanorod vanadatesulfuric acid (VSA NRs). The reaction is performed easily, is clean, and the product, VSA, is isolated by dilution of the mixture with water and then simple filtration (Scheme 2).

(Color online.) Synthesis of nanorod vanadatesulfuric acid.
In the structure of metavanadate ion, the oxygen atoms are tetrahedrally coordinated to the vanadium atoms. The tetrahedral VO4 units form infinite chains in the direction of the [001] axis. The VO4 chains are mutually bonded by means of electrostatic bonds of the positive ions [44]. In the IR spectrum of vanadatesulfuric acid, the bands that appeared at 3450 and 1640 cm−1 are safely attributed to the stretching and bending vibrations of the OH group, respectively. The peaks at 1050, and 1180 cm−1 are assigned to the sulfonic acid bonds of SOH, SO stretching, and SO asymmetric stretching, respectively. These additional signals to the IR spectrum of sodium metavanadate are evidenced in that of VSA. The absorption peaks that appeared at 960, 840 and 603 cm−1 are related to VO and VO stretching, respectively [40].
Powder X-ray diffraction (XRD) pattern and transmission electron microscopy (TEM) of NaVO3 and VSA are provided in Fig. 1. The size of the VSA particles was evaluated from X-ray line broadening using the Debye–Scherrer formula. For the (001) reflection, the average size of the VSA particles was estimated to be around 16 nm. Based on the TEM image, the catalyst showed a needle-like morphology with a narrow size distribution from 15 to 20 nm and a mean size of 17 nm, confirming the results calculated from Scherrer's equation.
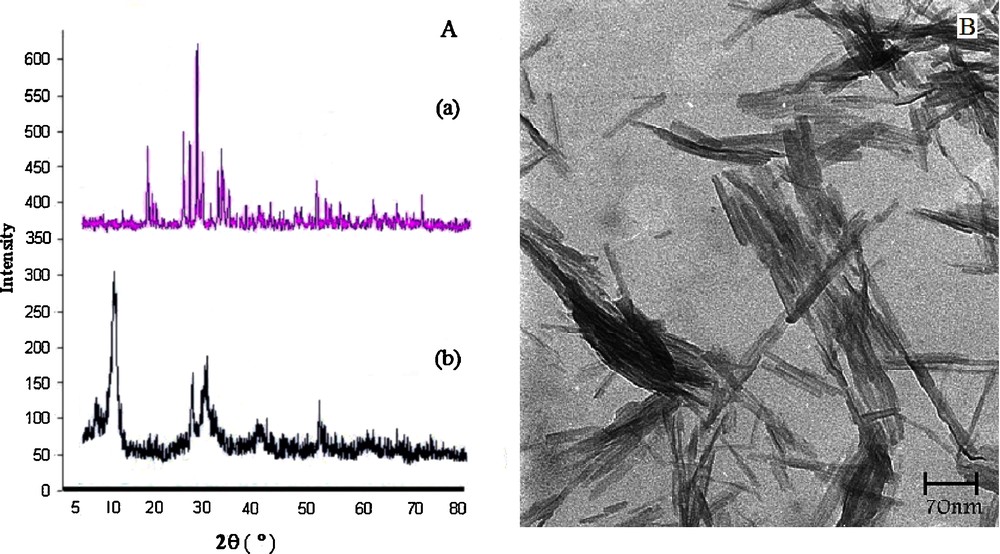
(Color online.) A. Powder X-ray diffraction patterns of (a) sodium metavanadate, and (b) nanorod vanadatesulfuric acid (VSA NRs) particles. B. Transmission electron microscopy image of VSA particles (needle-like, 15–20 nm in size).
3.1 Effect of solvent and catalyst concentration on the synthesis of hexahydroacridine-1,8-diones
We initially investigated the catalytic activity of VSA for the synthesis of hexahydroacridine-1,8-diones under different reaction conditions. For a comparison of the results between solvent and solvent-free conditions, the solvent effect was investigated in the condensation of dimedone (2 mmol), benzaldehyde (1 mmol) and ammonium acetate (1 mmol) in the presence of vanadatesulfuric acid NRs (0.1 mmol) as a typical reaction. As shown in Table 1, the solvent-free system was found as the best condition as compared with the solution tests with various solvents such as ethanol, methanol, acetonitrile and water, so that the reaction was completed after 20 min in excellent yields (93%) under solvent-free conditions.
Solvent effect on the reaction of dimedone, benzaldehyde, ammonium acetate catalyzed by VSA NRs.
Entry | Solvent (reflux) | Time (min) | Yielda (%) |
1 | CH3CH2OH | 300 | 62 |
2 | CH3OH | 450 | 65 |
3 | H2O | 350 | 58 |
4 | CH3CN | 300 | 56 |
5 | Solvent-free conditionsb | 20 | 93 |
a Isolated yields.
b At 80 °C.
After having investigated the reaction media and then chosen solvent-free conditions as the best ones, the effect of catalyst loading was studied on the solvent-free system (Table 1). For this, the parallel reactions for the synthesis of 4d as the typical product were run in the presence of various amounts of VSA NRs catalyst (5, 7, 10, and 15 mol%). As shown in Table 1, the absence of catalyst in the reaction system led to trace amounts of the product. On the other hand, an increase in the amount of catalyst causes an egregious improvement in the reaction progress, so that the product yield, which is 70% for 5 mol% of catalyst, reaches 93% in the presence of 10 mol% of catalyst. This showed that catalyst concentration plays a major role in the optimization of the product yield. The use of 15 mol % of VSA decreased the product yield to 90%. This was because beyond a certain concentration, there is an excess of catalyst sites with respect to what is actually required by the reactant molecules, and hence, the additional catalyst does not increase the rate of the reaction. Therefore, in all further reactions, 10 mol% of the catalyst were used due to the satisfactory yield of the product obtained in a reasonably short time.
With a view to improving yields, the reaction was performed using different quantities of reagents. The best results were obtained with a 2:1:1 ratio of dimedone, benzaldehyde, and ammonium acetate, respectively.
The effect of temperature on the reaction rate and on the product yields was also investigated. Faster reactions were observed when the temperature was increased to 80 °C, but the product yield decreased at higher temperature (Table 2).
3.2 Synthesis of hexahydroacridine-1,8-diones catalyzed by nanorod vanadatesulfuric acid
To demonstrate the generality of this method, in the next step, we investigated the scope of this reaction under optimized conditions (VSA NRs 10 mol%, solvent-free, 80 °C); the results were collected in Table 3. As shown in Table 3, this method is equally effective irrespective of the nature and positions of the substituents attached to the phenyl ring of the aromatic aldehyde. Strongly activating (7, 11, 15, 19 entries), weakly activating (13 entry), weakly deactivating (2, 3, 5, 6, 9, 10, 17 entries), and strongly deactivating (8, 14, 16, 20 entries) systems all give products with nearly equal ease, though it seems that benzaldehydes with electron-donating groups are deactivated and those with electron-withdrawing groups are activated under the current conditions. The versatility of the method has also been demonstrated by the tolerance of a number of functional groups such as NO2, OMe, Me and halogens. In addition, the aliphatic aldehydes such as butanal (12 entry) afforded an 80% yield in 45 min.
Preparation of substituted hexahydroacridine-1,8-dione catalyzed by VSA NRs under solvent-free conditions.
Entry | R1 | R2 | R | Product | Time (min) | Yieldb (%) | Mp (°C) | |
Founda | Reported | |||||||
1 | H | C6H5 | H | 4a | 40 | 93 | 278–280 | 279–281 |
2 | H | 4-ClC6H4 | H | 4b | 30 | 92 | 270–272 | 268–270 |
3 | H | 2,4-Cl2C6H3 | H | 4c | 35 | 90 | 313–314 | > 300 |
4 | Me | C6H5 | H | 4d | 20 | 93 | 255–257 | 258–260 |
5 | Me | 2-ClC6H4 | H | 4e | 30 | 90 | 222–224 | 220–222 |
6 | Me | 4-ClC6H4 | H | 4f | 15 | 93 | 297–299 | 298–300 |
7 | Me | 4-CH3OC6H4 | H | 4g | 35 | 85 | 281–283 | 278–280 |
8 | Me | 4-O2NC6H4 | H | 4h | 15 | 93 | 290–292 | 288–289 |
9 | Me | 4-BrC6H4 | H | 4i | 20 | 90 | 313–315 | 312–315 |
10 | Me | 2,6-Cl2C6H3 | H | 4j | 30 | 86 | 301–302 | – |
11 | Me | 3,4-(CH3O)2C6H3 | H | 4k | 40 | 85 | 287–290 | 288–291 |
12 | Me | CH3CH2CH2 | H | 4l | 45 | 80 | 255–257 | 250–252 |
13 | Me | 4-CH3C6H4 | H | 4m | 40 | 85 | 319–321 | 318–320 |
14 | Me | 3-O2NC6H4 | H | 4n | 25 | 90 | 293–295 | 294–296 |
15 | Me | 2-CH3OC6H4 | H | 4o | 45 | 80 | 250–252 | 247–249 |
16 | Me | 2-O2NC6H4 | H | 4p | 25 | 92 | 297–299 | 293–295 |
17 | Me | 2-BrC6H4 | H | 4q | 30 | 91 | 307–309 | > 300 |
18 | Me | C6H5 | C6H5 | 4r | 30 | 90 | 250–252 | 254–256 |
19 | Me | 4-CH3OC6H4 | C6H5 | 4s | 45 | 80 | 221–223 | 220–222 |
20 | Me | 3-O2NC6H4 | C6H5 | 4t | 25 | 92 | 273–275 | 276–278 |
a All products were characterized by 1H NMR and IR spectroscopy and comparison with those reported in the literature [24,41–43,45,46].
b Isolated yields.
A standard leaching experiment was conducted to prove that the reaction is heterogeneous. A model reaction was preceded for 10 min in the presence of catalyst in ethanol as the solvent, and then catalyst was removed by filtration. The reaction was allowed to proceed without catalyst. There was no change in the progress of the reaction, even after 10 h under reflux, indicating that no leaching has occurred and therefore no homogeneous catalyst was involved.
The activity of the recycled VSA was also examined according to typical experiment conditions. It is noteworthy that the catalyst was recovered simply by filtration without any acidic or basic work-up, even after its fourth use. Two types of experiments were performed (a) with make-up catalyst—the loss of catalyst (< 4%) was compensated through addition of fresh catalyst—and (b) without make-up of catalyst (Fig. 2). For this purpose, after completion of the typical reaction between benzaldehyde, ammonium acetate, and dimedone, the catalyst was recovered from the reaction mixture and then the recovered catalyst was oven-dried at 120 °C for 2 h prior to use. The dried recovered catalyst was then added to fresh substrates under the same experimental conditions. It was found that VSA NRs act as catalysts for five runs without a noticeable decrease in the product yield and in its catalytic activity.
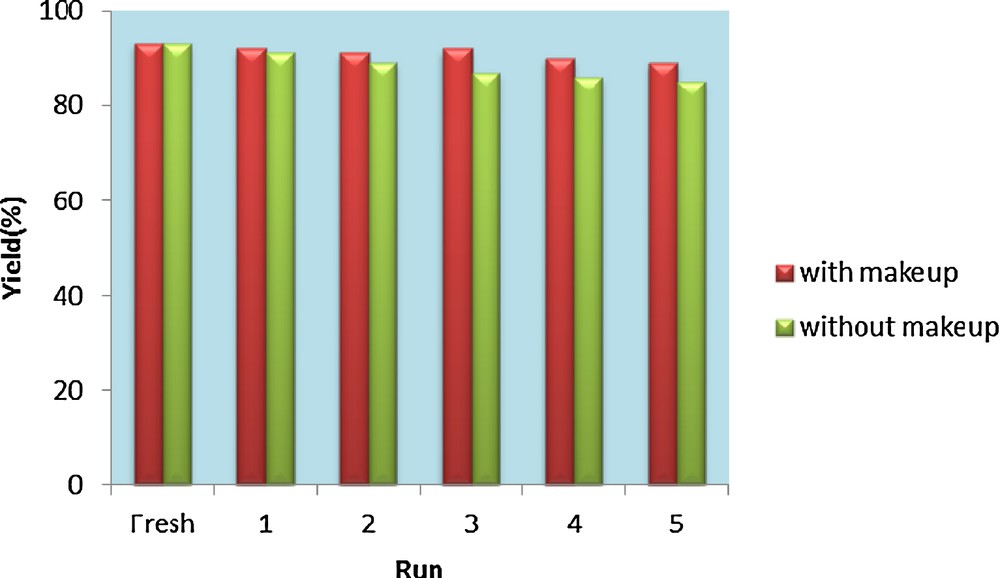
(Color online.) Reusability of vanadatesulfuric acid in the synthesis of hexahydroacridine-1,8-diones of benzaldehyde, ammonium acetate and dimedone.
It is well known that vanadium-based catalytic systems, including vanadium phosphorous oxides catalysts such as (VO)2P2O7, have been proved to change structurally when they are used in liquid-phase oxidations [47]. This point was investigated in the current study. The XRD diffraction patterns and infrared spectra of fresh and used VSA catalysts used in the synthesis of hexahydroacridine-1,8-diones are presented in Fig. 3. Both the fresh catalyst and those recovered after the fifth use exhibited similar XRD patterns indicating that the structural properties of the catalysts were not affected by the reaction medium (Fig. 3A). Also infrared spectra of fresh and used VSA catalysts confirmed that the structure of the catalyst remained unchanged after recycling (Fig. 3B).

(Color online.) A. X-ray diffraction patterns of the recovered catalyst after the fifth use. B. FT-IR spectra of vanadatesulfuric acid, (a) before use and (b) after having been reused five times.
3.3 Comparative results in terms of efficiency and greenness
In order to show the advantages of our method over previously reported ones, some of our results are compared in Table 3 to those of some other methods. These results showed that the yield/time ratio and turnover number (TON) and turnover frequency (TOF) of the present method are better or comparable to the other reported results for the synthesis of hexahydroacridine-1,8-diones. A catalyst may be described by its turnover number (TON). Each time the complete catalyst cycle occurs, we can consider one catalytic turnover to have been completed (moles of product formed per mole of catalyst). The catalytic reaction rate can be conveniently given in terms of the turnover frequency (TOF) measured in turnovers per unit time (often per hour). In an industrial catalytic process, TON is a value that indicates the amount of feed or substrate converted per measured amount of catalyst.
Finally, the two important parameters (E-factor and RME) from the point of view of green chemistry were evaluated to evidence the green character of the reaction according to literature reports [48]. According to [48], a lower E-factor and higher RME values suggest a more green character for a reaction. Table 4 shows a comparison between calculated E-factors and RME of the reactions catalyzed by vanadatesulfuric acid NRs and some other acid catalysts. It can be seen that the reactions using VSA NRs are considerably cleaner and more competent than those involving almost all other catalysts. In particular, the waste produced (E-factor) during the reaction is very much less than with CeCl3, Amberlist-15 or [Hmim]TFA. However, it is to be noted that the E-factor ignores recyclability factors such as the reused catalyst and recycled solvents, which obviously increases the accuracy of E-factor evaluation.
Comparison of the efficiency of various catalysts in the synthesis of hexahydroacridine-1,8-diones.
Entry | Catalyst | Conditions | Time (h) | Yielda | TON/TOFb | E-factor | RMEc | Ref. |
1 | CAN | PEG/50 °C | 3.5–4 | 93–98 | 19.2/4.8 | 0.67 | 0.85 | [49] |
2 | CeCl3·7H2O | [bmim][BF4]/100 °C | 6 | 82–95 | 8.8/1.5 | 7.63 | 0.54 | [43] |
3 | Zn(OAc)2 | H2O/(reflux) | 2–3 | 84–94 | 091/0.36 | 0.83 | 0.64 | [25] |
4 | Amberlist-15 | CH3CN (reflux) | 4.5–6.5 | 90 | 0.86/0.17 | 23.65 | 0.70 | [22] |
5 | [Hmim]TFA | Solvent-free/80 °C | 4.5–7 | 78–89 | 1.69/0.37 | 273.43 | 0.74 | [34] |
6 | SBSAN | Solvent-free/50 °C | 2–5 | 88–93 | 21.3/10.7 | 0.17 | 0.80 | [50] |
7 | VSA NRsd | Solvent-free/80 °C | 20–45 min | 80–93 | 9.3/14 | 0.15 | 0.80 | – |
a Isolated yield.
b TOF = turnover number (TON)/hours.
c Reaction mass efficiency.
d Refers to our catalyst.
4 Conclusion
In this paper, we have described a rapid and very efficient one-pot three-component reaction between 1,3-cyclohexanediones, benzaldehyde derivatives, and ammonium acetate (or aniline) for the synthesis of hexahydroacridine-1,8-dione derivatives using nanorod vanadatesulfuric acid (VSA NRs) catalyst under solvent-free conditions. Nanorod structure vanadatesulfuric acid (VSA NRs) catalyst showed some advantages. It is insoluble in common organic solvents, causes low corrosion, and shows environmental acceptability. Also, the products could be easily separated from the reaction mixture and the catalyst is recoverable without notable decrease in its activity. This novel catalytic system exhibits some advantages, such as environmentally benign character, mild reaction conditions, short reaction times, high yields, easy handling, as well as good reusability. Moreover, the non-hygroscopic and inexpensive characters of this transformation reaction are other advantages of this procedure. Finally, some greenness aspects have been investigated and compared with data from previous reports.
Acknowledgements
We are thankful to the Yasouj University for their partial support of this work.