1 Introduction
Multicomponent reaction (MCR) strategy has sufficient advantages over conventional linear-type synthesis due to its flexible, convergent and atom-efficient nature [1,2]. In recent years, the synthesis of combinatorial small-molecule heterocyclic libraries has emerged as a valuable tool in the search for novel lead structures [3]. Thus, the success of combinatorial chemistry in drug discovery is considerably dependent on further advances in heterocyclic MCR methodology and, according to current synthetic requirements, ecologically pure MCRs such as solvent-free or ‘on-water’ reactions are particularly welcome.
In recent years, the concept of “privileged medicinal scaffolds”1 [4,5] has emerged as one of the guiding principles of the drug discovery process. It involves the utilization of molecular frameworks with inherent potential for biological activity. These privileged scaffolds commonly consist of a rigid hetero ring system that assigns a well-defined orientation of appended functionalities for target recognition [6].
The chromene moiety often appears as an important structural element in both biologically active and natural compounds. It is widely performed in natural alkaloids, flavonoids, tocopherols, and anthocyanins [7]. Moreover, in recent years functionalized chromenes have played an ever-increasing role in the synthetic approaches to promising compounds in the field of medicinal chemistry [8].
Among the different types of chromene systems, 2-amino-4H-chromenes (or 2-amino-4H-benzo[b]pyranes) are of particular utility as they belong to privileged medicinal scaffolds serving for the generation of small-molecule ligands with highly pronounced spasmolitic, diuretic, anticoagulant, and antianaphylactic activities [9]. The current interest in 2-amino-4H-chromene derivatives bearing nitrile functionality arises from their potential application in the treatment of human inflammatory TNFα-mediated diseases, such as rheumatoid and psoriatic arthritis, and in cancer therapy [10].
In the course of our studies on the electrochemical transformations of organic compounds [11], we have successfully applied the electrocatalytic procedure to form a 2-amino-4H-chromene scaffold from salicylaldehydes and two different C–H acids [12].
Recently, we have also accomplished solvent-free and ‘on water’ transformations of salicylaldehydes and malononitrile [13] or cyanoacetates [14], salicylaldehyde, malononitrile and cyanoacetates [15], nitroalkanes [16] or pyrazolin-5-ones [17] into substituted 2-amino-4H-chromenes. But an efficient convenient and ecologically reasonable procedure for the synthesis of substituted 2-amino-4H-chromenes based on multicomponent reaction of salicylaldehydes, malonitrile and cyanoacetamides has yet to be developed. Considering our preliminary results in the field of solvent-free and ‘on water’ reactions of carbonyl compounds and CH acids, we were prompted to design a convenient and facile environmentally benign chemical methodology for the efficient synthesis of the new type of the substituted 2-amino-4H-chromenes based on the multicomponent reaction of salicylaldehydes, malononitrile and cyanoacetamides.
2 Results and discussion
As it follows from the introduction, we were prompted to design a convenient facile and ecologically reasonable methodology for the efficient synthesis of new substituted 2-amino-4H-chromenes based on the multicomponent reaction of salicylaldehydes, malononitrile, and cyanoacetamides.
Thus, in the present study we report on the new multicomponent transformation of salicylaldehydes 1a–f, malononitrile 2 and cyanoacetamides 3, 5a,b into substituted 2-amino-4H-chromene 4a–f, 6a,b (Scheme 1, Tables 1 and 2).
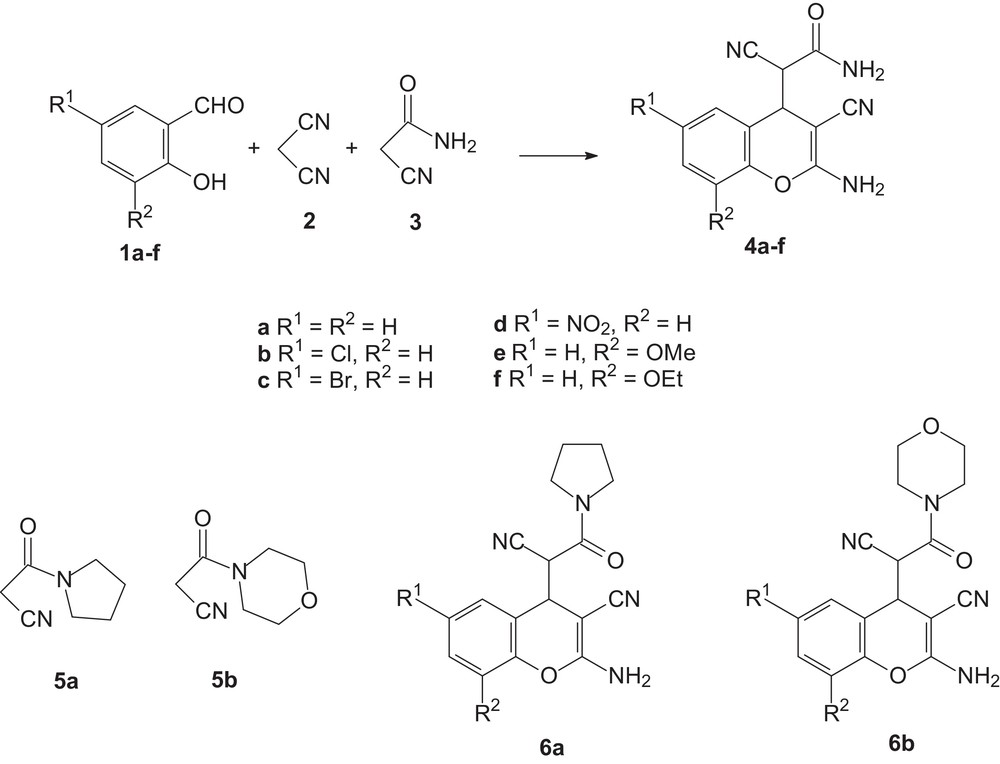
Multicomponent transformation of salicylaldehydes 1, malononitrile 2 and cyanoacetamides 3 or 5 into substituted 2-amino-4H-chromenes 4 or 6.
Multicomponent transformation of salicylaldehyde 1a, malononitrile 2 and cyanoacetamide 3 into 2-amino-4H-chromene 4aa.
Entry | Catalyst, % mol | Solvent | Time, h | Tempera-ture, °C | Yield (%)b |
1 | NaOAc, 5 | – | 0.25 | 20 | 25 |
2 | KF, 5 | – | 0.25 | 20 | 30 |
3 | Na2CO3, 5 | – | 0.25 | 20 | 28 |
4 | NaOH, 5 | – | 0.25 | 20 | 35 |
5 | NaOH, 5 | – | 0.5 | 20 | 58 |
6 | NaOH, 10 | – | 0.5 | 20 | 60 |
7 | NaOH, 5 | H2Oc | 1.0 | 60 | 71 |
8 | NaOH, 5 | H2Oc | 2.0 | 60 | 83 |
9 | NaOH, 5 | H2Oc | 3.0 | 60 | 95d |
a 5 mmol of salicylaldehyde 1a, 5 mmol of malononitrile 2, 5 mmol of cyanoacetamide 3.
b 1H NMR data.
c 3 mL H2O.
d Isolated yield.
On-water multicomponent transformation of salicylaldehydes 1a-f, malononitrile 2 and cyano-acetamides 3, 5a,b into 2-amino-4H-chromenes 4a-f, 6a,ba.
Entry | Salicylaldehyde | R1 | R2 | Cyanoacetamide | Product | Yield (%)b |
1 | 1a | H | H | 3 | 4a | 95 (3:1) |
2 | 1b | Cl | H | 3 | 4b | 90 (7:5) |
3 | 1c | Br | H | 3 | 4c | 81 (2:1) |
4 | 1d | NO2 | H | 3 | 4d | 85 (6:1) |
5 | 1e | H | MeO | 3 | 4e | 88 (2:1) |
6 | 1f | H | EtO | 3 | 4f | 80 (3:1) |
7 | 1a | H | H | 5a | 6a | 82 (8:1) |
8 | 1a | H | H | 5b | 6b | 87 (1:1) |
a 5 mmol of salicylaldehyde 1, 5 mmol of malononitrile 2, 5 mmol of cyanoacetamide 3, 5a or 5b, 3 mL H2O, 60 °C, 3 h.
b Isolated yield, in parentheses ratio of diastereomers.
The solvent-free reaction of aldehyde 1a, malononitrile 2 and cyanoacetamide 3 in the presence of NaOAc, KF, Na2CO3 or NaOH with 15 min grinding (entries 1-4, Table 1) resulted in the formation of 2-amino-4H-chromenes 4a in the 25, 30, 28 and 35% yields, respectively. Under increasing reaction time (30 min) in the presence of NaOH, 2-amino-4H-chromene 4a was obtained in 58% yield. An increasing quantity of NaOH had no sufficient influence on the yield of 4a (entry 5, Table 1).
Under ‘on-water’ conditions in the presence of NaOH at 60 °C, 2-amino-4H-chromene 4a was formed in 71, 83 and 95% yields in 1, 2 and 3 h of reaction time (entries 7–9, Table 1). It should be mentioned that compared to the only known previous synthesis of 4a, which was carried out using the same reagents by electrochemical induction in an alcohol as the solvent [12], chromene 4a under ‘on-water’ conditions was obtained in higher yield (95 instead of 73%) and with a higher diastereomeric ratio (3:1 instead of 1:1), using an easier methodology.
Under optimum conditions ‘on-water’ reaction thus found (5 mol% of NaOH as catalyst, 60 °C, 3 h reaction time) substituted 2-amino-4H-chromenes 4a-f and 6a,b were obtained in 80–95% yields (Table 2). As practically pure 2-amino-4H-chromenes 4a–f and 6a,b were formed at the end of the reaction, the reaction mixture was only filtered off in the end of the process, washed with water, and dried to isolate pure substituted 2-amino-4H-chromenes 4a–f and 6a,b. The liquid phase after filtration (solution of NaOH in water) was used three times in the synthesis of 2-amino-4H-chromene 4a, with a slight decrease of the yield in the last experiment to 85%.
According to 1H and 13C NMR analysis, the 2-amino-4H-chromenes 4a–f, 6a,b thus obtained were mixtures of two diastereoisomers (Table 2, entries 1–8). From the thermodynamic point of view, the more abundant diastereoisomer should possess a (R*, S*)-configuration (Fig. 1). For compounds 4a and 6b, NMR HSQCED and HMBC spectra were recorded to establish the position of all C-atoms. The comparison of experimental and calculated [18] NMR 13C spectra indicated a (R*, S*)-configuration for the main isomer of 4a and for the main isomer of 6b (Fig. 1).
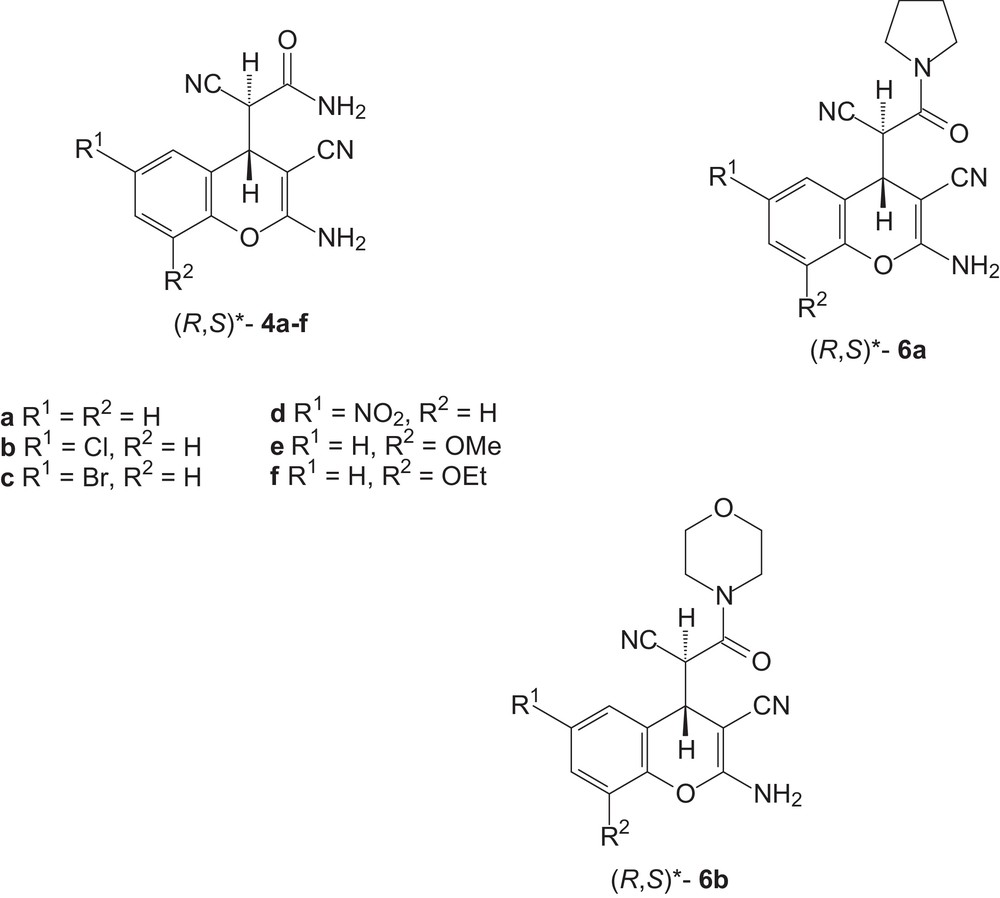
Proposed configuration for the more abundant diastereoisomers of 4a–f, 6a and 6b.
With the above results taken into consideration and the mechanistic data on the solvent-free cascade formation of 2-amino-4H-chromene scaffold from salicylaldehydes and malononitrile [12], the following mechanism for ‘on-water’ sodium hydroxide catalyzed multicomponent transformation of salicylaldehydes 1, malononitrile 2, and cyanoacetamide 3 into substituted 2-amino-4H-chromenes 4 is proposed.
As a first step, the NaOH-initiated deprotonation of malononitrile leads to the formation of the malononitrile anion:
Then a Knoevenagel condensation of the malononitrile anion and salicylaldehyde 1 takes place, with elimination of the hydroxide anion and formation of benzylidenemalononitrile 7 (Scheme 2, [19]).

Knoevenagel adduct 7 formation.
Next, three reaction pathways are possible for Knoevenagel adduct 7 (Scheme 3).

Mechanism of multicomponent transformation of salicylaldehydes 1, malononitrile 2 and cyanoacetamides 3 into substituted 2-amino-4H-chromenes 4.
The Michael addition of malononitrile to the Knoevenagel adduct 7 followed by intramolecular cyclization leads to the corresponding (2-amino-3-cyano-4H-chromen-4-yl)malononitrile 8 (pathway A).
(2-Amino-3-cyano-4H-chromen-4-yl)malononitrile 8 was detected in the reaction mixture when the solvent-free reaction of salicylaldehyde 1, malononitrile 2 and cyanoacetamide 3 in the presence of NaOH was completed in shorter reaction times (1 h and 2 h) in 13% and 5% yields based on the starting salicylaldehyde 1.
Recently, O′Callaghan et al. have noted that in alcoholic solution, (2-amino-3-cyano-4H-chromen-4-yl)malononitrile 8 could exist in equilibrium with the corresponding 2-imino-2H-chromene-3-carbonitrile 9 and malononitrile under certain reaction conditions [20]. If such an equilibrium exists in our case, the uptake of stronger CH acid (malononitrile in our case) from the equilibrium by salicylaldehyde 1 could facilitate the base-promoted addition of weaker CH acid 3 (cyanoacetamide) to 2-imino-2H-chromene 9, which results in the full conversion of 8 into the desired 2-amino-4H-chromene 4.
3 Conclusions
Thus, sodium hydroxide as a catalyst can produce under ‘on-water’ conditions the selective multicomponent transformation of salicylaldehydes, malononitrile and cyanoacetamides into substituted 2-amino-4H-chromenes in excellent yields, from 80 to 95%. This new process opens an efficient and convenient multicomponent way to create substituted 2-amino-4H-chromenes — promising compounds for different biomedical applications.
This ‘on-water’ multicomponent catalytic procedure utilizes simple equipment; it is easily carried out and is valuable from the viewpoint of environmentally benign diversity-oriented large-scale processes. This efficient approach to substituted 2-amino-4H-chromenes represents a new synthetic concept for the modern synthesis of bioactive compounds that integrates a multicomponent strategy with ‘on-water’ reaction procedure, and allows for the combination of the synthetic virtues of MCR with ecological benefits of ‘on-water’ reactions; therefore, it makes the MCR strategy a step closer to a notion of “ideal synthesis” [21].
4 Experimental
4.1 General remarks
All melting points were measured with a Gallenkamp melting point apparatus and are uncorrected. 1H and 13C NMR spectra were recorded with a Bruker Avance II-300 spectrometer at ambient temperature. Chemical shifts values are relative to Me4Si. IR spectra were registered with a Bruker ALPHA-T FT-IR spectrometer in KBr pellets. Mass-spectra (EI = 70 eV) were obtained directly with a Finningan MAT INCOS 50 spectrometer. High-resolution mass spectra (HRMS) were measured on a Bruker micrOTOF II instrument using electrospray ionization (ESI). The internal calibration was done with Electrospray Calibrant Solution (Fluka). A syringe injection was used for solutions in acetonitrile, or methanol, (flow rate 3 μL/min). Nitrogen was applied as a dry gas; the interface temperature was set at 180 °C. All chemicals were purchased from commercial sources.
4.2 General procedure
A mixture of salicylaldehyde 1 (5 mmol), malononitrile (0.33 g, 5 mmol), cyanoacetamide (5 mmol), and sodium hydroxide (0.02 g, 0.5 mmol) in water (5 mL) was stirred at 60 °C for 3 h. Then the reaction mixture was allowed to cool. The resulting solid was filtered off, rinsed twice with cold water (2 mL × 5 mL), and then dried to give the corresponding pure 2-amino-4H-chromene.
4.2.1 2-(2-amino-3-cyano-4H-chromen-4-yl)-2-cyanoacetamide (4a)
White solid (1.21 g, 95%); diastereomeric ratio 3:1; mp 169–171 °C; νmax (KBr): 3431, 3337, 2937, 2190, 1680, 1650, 1606, 1428 cm−1; HRMS (ESI): found m/z 277.0688; calcd for C13H10N4NaO2 [M + Na]+ 277.0696; major diastereomer: 1H NMR (300 MHz, DMSO-d6) δ 3.94 (d, 3JH,H = 5.2 Hz, 1H, CH), 4.26 (d, 3JH,H = 5.2 Hz, 1H, CH), 7.05–7.45 (m, 6H, Ar, NH2), 7.59 (s, 1H, CONH2), 7.86 (s, 1H, CONH2) ppm; 13C NMR (75 MHz, DMSO-d6) δ 36.9, 47.7, 51.5, 116.2, 116.9, 119.4, 119.8, 124.5, 128.7, 129.4, 149.9, 163.1, 165.0 ppm; minor diastereomer: 1H NMR (300 MHz, DMSO-d6) δ 3.95 (d, 3JH,H = 4.7 Hz, 1H, CH), 4.29 (d, 3JH,H = 4.7 Hz, 1H, CH), 7.05–7.45 (m, 6H, Ar, NH2), 7.53 (s, 1H, CONH2), 7.73 (s, 1H, CONH2) ppm; 13C NMR (75 MHz, DMSO-d6) δ 36.9, 48.7, 50.0, 116.2, 116.9, 119.8, 120.9, 124.9, 128.4, 129.1, 149.8, 163.1, 164.8 ppm.
4.2.2 2-(2-amino-6-chloro-3-cyano-4H-chromen-4-yl)-2-cyanoacetamide (4b)
White solid (1.30 g, 90%); diastereomeric ratio 7:5; mp 158–159 °C; νmax (KBr): 3405, 3312, 2935, 2202, 1676, 1649, 1600, 1424 cm−1; HRMS (ESI): found m/z 311.0301; calcd for C13H9ClN4NaO2 [M + Na]+ 311.0306; major diastereomer: 1H NMR (300 MHz, DMSO-d6) δ 4.04 (d, 3JH,H = 4.4 Hz, 1H, CH), 4.29 (d, 3JH,H = 4.4 Hz, 1H, CH), 7.11 (d, 3JH,H = 8.8 Hz, 1H, Ar), 7.26 (s, 2H, NH2), 7.39 (dd, 3JH,H = 8.8 Hz, 4JH,H = 2.3 Hz, 1H, Ar), 7.52 (d, 4JH,H = 2.3 Hz, 1H, Ar), 7.56 (s, 1H, CONH2), 7.70 (s, 1H, CONH2) ppm; 13C NMR (75 MHz, DMSO-d6) δ 36.8, 48.5, 49.5, 116.7, 117.5, 119.4, 122.8, 128.0, 128.2, 129.0, 148.6, 162.8, 164.6 ppm; minor diastereomer: 1H NMR (300 MHz, DMSO-d6) δ 3.98 (d, 3JH,H = 5.1 Hz, 1H, CH), 4.27 (d, 3JH,H = 5.1 Hz, 1H, CH), 7.12 (d, 3JH,H = 8.8 Hz, 1H, Ar), 7.28 (d, 4JH,H = 2.5 Hz, 1H, Ar), 7.30 (s, 2H, NH2), 7.41 (dd, 3JH,H = 8.8 Hz, 4JH,H = 2.5 Hz, 1H, Ar), 7.68 (s, 1H, CONH2), 7.88 (s, 1H, CONH2) ppm; 13C NMR (75 MHz, DMSO-d6) δ 36.6, 47.4, 51.0, 116.7, 118.0, 119.4, 121.3, 128.2, 128.3, 129.2, 148.7, 162.8, 164.8 ppm.
4.2.3 2-(2-amino-6-bromo-3-cyano-4H-chromen-4-yl)-2-cyanoacetamide (4c)
White solid (1.35 g, 81%); diastereomeric ratio 1:2; mp 171–173 °C; νmax (KBr): 3440, 3324, 2935, 2191, 1675, 1643, 1595, 1428 cm−1; HRMS (ESI): found m/z 354.9794; calcd for C13H9BrN4NaO2 [M + Na]+ 354.9801; major diastereomer: 1H NMR (300 MHz, DMSO-d6) δ 4.04 (d, 3JH,H = 4.2 Hz, 1H, CH), 4.29 (d, 3JH,H = 4.2 Hz, 1H, CH), 7.05 (d, 3JH,H = 8.7 Hz, 1H, Ar), 7.26 (s, 2H, NH2), 7.51 (d, 3JH,H = 8.7 Hz, 1H, Ar), 7.56 (s, 1H, CONH2), 7.66 (s, 1H, Ar), 7.70 (s, 1H, CONH2) ppm; 13C NMR (75 MHz, DMSO-d6) δ 36.7, 47.4, 49.6, 116.2, 118.4, 119.4, 123.2, 130.9, 131.0, 131.8, 149.1, 162.7, 164.6 ppm; minor diastereomer: 1H NMR (300 MHz, DMSO-d6) δ 3.98 (d, 3JH,H = 4.9 Hz, 1H, CH), 4.27 (d, 3JH,H = 4.9 Hz, 1H, CH), 7.06 (d, 3JH,H = 8.7 Hz, 1H, Ar), 7.30 (s, 2H, NH2), 7.41 (s, 1H, Ar), 7.54 (d, 3JH,H = 8.7 Hz, 1H, Ar), 7.69 (s, 1H, CONH2), 7.87 (s, 1H, CONH2) ppm; 13C NMR (75 MHz, DMSO-d6) δ 36.4, 47.4, 51.1, 115.9, 116.7, 119.4, 121.7, 130.9, 131.1, 132.1, 149.2, 162.7, 164.7 ppm.
4.2.4 2-(2-amino-3-cyano-6-nitro-4H-chromen-4-yl)-2-cyanoacetamide (4d)
White solid (1.27 g, 85%); diastereomeric ratio 6:1; mp 183–184 °C; νmax (KBr): 3439, 3312, 2931, 2192, 1680, 1648, 1595, 1421 cm−1; HRMS (ESI): found m/z 322.0542; calcd for C13H9N5NaO4 [M + Na]+ 322.0547; major diastereomer: 1H NMR (300 MHz, DMSO-d6) δ 4.12 (d, 3JH,H = 4.0 Hz, 1H, CH), 4.45 (d, 3JH,H = 4.0 Hz, 1H, CH), 7.32 (d, 3JH,H = 9.0 Hz, 1H, Ar), 7.44 (s, 2H, NH2), 7.59 (s, 1H, CONH2), 7.79 (s, 1H, CONH2), 8.21 (dd, 3JH,H = 9.0 Hz, 4JH,H = 2.5 Hz, 1H, Ar), 8.45 (d, 4JH,H = 2.5 Hz, 1H, Ar) ppm; 13C NMR (75 MHz, DMSO-d6) δ 36.8, 48.6, 49.6, 116.6, 117.6, 119.0, 122.0, 124.8, 124.9, 143.8, 154.3, 162.2, 164.5 ppm; minor diastereomer: 1H NMR (300 MHz, DMSO-d6) δ 4.07 (d, 3JH,H = 5.0 Hz, 1H, CH), 4.45 (d, 3JH,H = 5.0 Hz, 1H, CH), 7.40 (d, 3JH,H = 9.3 Hz, 1H, Ar), 7.48 (s, 2H, NH2), 7.72 (s, 1H, CONH2), 7.89 (s, 1H, CONH2), 8.28 (dd, 3JH,H = 9.3 Hz, 4JH,H = 2.5 Hz, 1H, Ar), 8.50 (d, 4JH,H = 2.5 Hz, 1H, Ar) ppm; 13C NMR (75 MHz, DMSO-d6) δ 36.5, 47.3, 51.1, 116.6, 117.9, 119.2, 120.5, 125.1, 125.2, 143.4, 154.3, 162.2, 164.6 ppm.
4.2.5 2-(2-amino-3-cyano-8-methoxy-4H-chromen-4-yl)-2-cyanoacetamide (4e)
White solid (1.25 g, 88%); diastereomeric ratio 2:1; mp 161–163 °C; νmax (KBr): 3425, 3352, 2898, 2182, 1692, 1641, 1581, 1220 cm−1; HRMS (ESI): found m/z 307.0799; calcd for C14H12N4NaO3 [M + Na]+ 307.0802; major diastereomer: 1H NMR (300 MHz, DMSO-d6) δ 3.83 (s, 3H, CH3), 3.91 (d, 3JH,H = 4.7 Hz, 1H, CH), 4.25 (d, 3JH,H = 4.7 Hz, 1H, CH), 6.90–7.30 (m, 5H, Ar, NH2), 7.52 (s, 1H, CONH2), 7.71 (s, 1H, CONH2) ppm; 13C NMR (75 MHz, DMSO-d6) δ 37.1, 48.5, 49.9, 55.7, 111.7, 116.8, 119.6, 119.7, 121.8, 124.6, 139.1, 147.1, 163.0, 164.7 ppm; minor diastereomer: 1H NMR (300 MHz, DMSO-d6) δ 3.83 (s, 3H, CH3), 3.89 (d, 3JH,H = 5.2 Hz, 1H, CH), 4.21 (d, 3JH,H = 5.2 Hz, 1H, CH), 6.75–7.35 (m, 5H, Ar, NH2), 7.58 (s, 1H, CONH2), 7.84 (s, 1H, CONH2) ppm; 13C NMR (75 MHz, DMSO-d6) δ 37.0, 47.6, 51.4, 55.7, 112.1, 116.8, 119.4, 119.7, 120.6, 124.3, 139.2, 147.1, 163.0, 164.9 ppm.
4.2.6 2-(2-amino-3-cyano-8-ethoxy-4H-chromen-4-yl)-2-cyanoacetamide (4f)
White solid (1.19 g, 80%); diastereomeric ratio 3:1; mp 167–169 °C; νmax (KBr): 3434, 3322, 2936, 2190, 1678, 1654, 1580, 1426 cm−1; HRMS (ESI): found m/z 321.0954; calcd for C15H14N4NaO3 [M + Na]+ 321.0958; major diastereomer: 1H NMR (300 MHz, DMSO-d6) δ 1.36 (t, 3JH,H = 6.9 Hz, 3H, CH3), 3.91 (d, 3JH,H = 5.2 Hz, 1H, CH), 4.11 (q, 3JH,H = 6.9 Hz, 2H, CH2), 4.22 (d, 3JH,H = 5.2 Hz, 1H, CH), 6.75–7.30 (m, 5H, Ar, NH2), 7.57 (s, 1H, CONH2), 7.82 (s, 1H, CONH2) ppm; 13C NMR (75 MHz, DMSO-d6) δ 14.6, 37.1, 47.7, 51.4, 64.3, 113.4, 116.9, 119.7, 119.8, 120.4, 124.3, 139.5, 146.3, 163.0, 164.9 ppm; minor diastereomer: 1H NMR (300 MHz, DMSO-d6) δ 1.36 (t, 3JH,H = 6.9 Hz, 3H, CH3), 3.92 (d, 3JH,H = 4.7 Hz, 1H, CH), 4.11 (q, 3JH,H = 6.9 Hz, 2H, CH2), 4.24 (d, 3JH,H = 4.7 Hz, 1H, CH), 6.75–7.30 (m, 5H, Ar, NH2), 7.51 (s, 1H, CONH2), 7.70 (s, 1H, CONH2) ppm; 13C NMR (75 MHz, DMSO-d6) δ 14.6, 37.0, 48.6, 49.9, 64.3, 113.0, 116.8, 119.4, 119.7, 121.9, 124.7, 139.4, 146.3, 163.0, 164.7 ppm.
4.2.7 2-amino-4-(1-cyano-2-oxo-2-pyrrolidin-1-ylethyl)-4H-chromen-3-carbonitrile (6a)
White solid (1.26 g, 82%); diastereomeric ratio 8:1; mp 152–153 °C; νmax (KBr): 3475, 2979, 2185, 1636, 1576, 1446, 1413 cm−1; HRMS (ESI): found m/z 331.1167; calcd for C17H16N4NaO2 [M + Na]+ 331.1165; major diastereomer: 1H NMR (300 MHz, DMSO-d6) δ 1.55–1.95 (m, 4H, CH2), 2.84–2.97 (m, 1H, CH2), 3.19–3.41 (m, 2H, CH2), 3.49–3.62 (m, 1H, CH2), 4.20 (d, 3JH,H = 6.2 Hz, 1H, CH), 4.28 (d, 3JH,H = 6.2 Hz, 1H, CH), 7.04–7.40 (m, 6H, Ar, NH2) ppm; 13C NMR (75 MHz, DMSO-d6) δ 23.6, 25.5, 36.9, 45.5, 46.1, 46.3, 50.5, 116.0, 116.8, 119.9, 120.5, 124.5, 128.3, 129.1, 150.0, 161.4, 163.1 ppm; minor diastereomer: 1H NMR (300 MHz, DMSO-d6) δ 1.55–1.95 (m, 4H, CH2), 2.84–2.97 (m, 1H, CH2), 3.19–3.41 (m, 2H, CH2), 3.49–3.62 (m, 1H, CH2), 4.14 (d, 3JH,H = 6.3 Hz, 1H, CH), 4.25 (d, 3JH,H = 6.3 Hz, 1H, CH), 7.04–7.40 (m, 6H, Ar, NH2) ppm; 13C NMR (75 MHz, DMSO-d6) δ 23.7, 25.4, 37.2, 45.8, 46.1, 46.6, 51.0, 115.5, 117.0, 119.4, 119.9, 124.3, 128.3, 129.3, 150.0, 161.6, 163.1 ppm.
4.2.8 2-amino-4-(1-cyano-2-morpholin-4-yl-2-oxoethyl)-4H-chromen-3-carbonitrile (6b)
White solid (1.41 g, 87%); diastereomeric ratio 2:1; mp 145–147 °C; νmax (KBr): 3335, 2929, 2204, 1648, 1581, 1442, 1419 cm−1; HRMS (ESI): found m/z 347.1120; calcd for C17H16N4NaO3 [M + Na]+ 347.1115; major diastereomer: 1H NMR (300 MHz, DMSO-d6) δ 3.20–3.75 (m, 8H, 4CH2), 4.20 (d, 3JH,H = 5.7 Hz, 1H, CH), 4.66 (d, 3JH,H = 5.7 Hz, 1H, CH), 7.05–7.45 (m, 6H, Ar, NH2) ppm; 13C NMR (75 MHz, DMSO-d6) δ 37.0, 42.2, 44.7, 46.1, 49.9, 65.7 (2CH2O), 116.1, 116.8, 119.9, 120.3, 124.6, 129.2 (2CH), 149.9, 162.1, 163.1 ppm; minor diastereomer: 1H NMR (300 MHz, DMSO-d6) δ 3.20–3.75 (m, 8H, 4CH2), 4.11 (d, 3JH,H = 6.6 Hz, 1H, CH), 4.58 (d, 3JH,H = 6.6 Hz, 1H, CH), 7.05–7.45 (m, 6H, Ar, NH2) ppm; 13C NMR (75 MHz, DMSO-d6) δ 37.5, 42.4, 43.2, 46.2, 50.7, 65.4 (2CH2O), 116.0, 117.2, 119.7, 120.0, 124.5, 128.6 (2CH), 150.0, 162.4, 163.2 ppm.
Acknowledgments
The authors gratefully acknowledge the financial support of the Russian Foundation for Basic Research (Project No. 13-03-00096а).
1 The term “privileged scaffolds or structures” was originally introduced by Merck researchers in their work on benzodiazepins.