1 Introduction
The development of new synthetic routes for certain compounds that are not easily accessible in an ecocompatible manner is quite a challenging objective in the field of contemporary synthetic chemistry. The regular and unavoidable induction of chemical wastes from various chemical transformations in chemical laboratories, bulk chemical synthesizing industries, and pharmaceuticals have greatly degraded the global environment. Hence, it is necessary to develop sustainable methods for compounds of synthetic interest. Multicomponent reactions (MCRs) have played a vital role in organic synthesis [1] and are considered as one of the premiere methods for rapid building of novel and complex molecular structures [2,3]. MCRs have also emerged as a powerful route for the synthesis of diverse biologically active heterocyclic scaffolds [4] and also in combinatorial synthesis [5]. Copper (I)-catalysed Huisgen's click coupling reaction (CuAAC) is a well-known MCR that can be used in highly efficient bond-forming processes such as diverse chemical synthesis [6], combinatorial chemistry in drug discovery [7], bioconjugation [8], and materials and surface science [9]. On the other hand, 1,2,3-triazolic systems serve as powerful pharmacophores [10] and potential targets in drug discovery due to their broad biological spectrum [11]. Apart from the pharmacophoric values, triazoles also act as corrosion-control agents, light stabilizers, fluorescent whiteners, and optical brighteners [12]. According to Wong et al. [13], the β-hydroxy 1,2,3-triazole can replace the peptide group in HIV-1 protease inhibitors. Most of these scaffolds have been synthesized by the reactions among epoxides, azides, and suitable terminal alkynes using the click chemistry approach, which is a 1,3-cycloaddition process [14]. Such strategies for the β-hydroxy triazoles have resulted in minimal by-product expulsions in the consecutive reaction steps. However, its requirement of additives, insignificant reactivity behaviour, and formation of undesirable side products by self-condensation of reactants were some of its drawbacks. Hence, ionic liquids (IL), both as green solvents and basement for many catalysts, can be used for catalyst recycling in many organic transformations [15].
Therefore, in order to devise an ecocompatible synthetic strategy for the synthesis of β-hydroxy triazoles, this study uses immobilized Cu(I) in IL to synthesize these potent pharmacophores. Thus, the IL-supported catalyst, along with the IL, was recycled for the synthesis of target products without much decrease in the corresponding yield percent. Subsequently, this resulted in a concise and simple method for the synthesis of β-hydroxy triazole scaffolds using catalyst loading at 5 mol% Cu(I)/g of the IL. It is pertinent to mention that the present investigation is an outcome of continuous efforts for the synthesis of bioactive molecules using ecocompatible methods [16].
2 Results and discussion
A preliminary experimentation was undertaken for the synthesis of β-hydroxy triazoles in which styrene oxide (1a) was treated with sodium azide and phenylacetylene (2a) in the presence of 5 mol% of Cu(I)/g of [bmim]Br. The reaction reached its completion at 80 °C, and the product, β-hydroxytriazole (3a), was obtained in 95% yield (Scheme 1). Further increment in the mole percentage of Cu(I) under the same conditions did not show any improvement in the yield of 3a. Hence, 5 mol% of Cu(I) loaded in 1 g of [bmim]Br was found to be sufficient for the synthesis of β-hydroxy triazole scaffolds, starting from ∼1 milimolar concentration of the reactant species in moderate temperature conditions (Table 1). In addition, epoxides and terminal alkynes were used to get better results from the reaction conditions mentioned above. Other ILs, such as [bmim]BF4, [bmim]PF6, [bmim]OH, and some common solvents such as toluene, PEG-200, DMF, CH3CN (ACN), and water were also tested as reaction media for carrying out the synthesis. Out of these, [bmim]OH did not yield the reaction product; instead, the initial reactant species were recovered after a reasonable period of time. It might be due to the deactivation of the catalyst as a result of the acid–base reaction between the Lewis acid and the basic IL. Thus, this combination for the transformation of reactants to products was ruled out. PEG-200 (Table 1, entry 11), like PEG-400 used in some protocols [18], has been also observed to carry out the click reaction smoothly, even in the absence of an argon atmosphere, but the possibility of catalyst recycling was the major issue associated with polyethylene glycols. Moreover, other organic solvents (Table 1) were not promising enough for the reaction under consideration. Water, the cheapest universal solvent for click reactions [19], also resulted in poor yield, which is due to oxidation of Cu(I) to Cu(II) during the reaction, thereby decreasing the overall yield towards minima (Table 1, entry 13). However, by using some suitable additive or stabilizer, it might also be possible to get these privileged structures under aqueous conditions.

Synthesis of β-hydroxy triazole scaffold.
Click reaction of styrene epoxide, NaN3 and phenylacetylene under different conditionsa.
Entry | Catalyst (mol %) | Solvent | Temp. (̊C) | Time (in hr)b | % of yield (3a)c |
1. | Cu(I) (0) | [bmim]Br | 80 | 50 | 0 |
2. | Cu(I) (2.5) | [bmim]Br | 80 | 20 | 75 |
3. | Cu(I) (5) | [bmim]Br | 80 | 10 | 90 |
4. | Cu(I) (5) | [bmim]Br | 90 | 15 | 90 |
5 | Cu(I) (7.5) | [bmim]Br | 80 | 15 | 90 |
6. | Cu(I) (5) | [bmim]BF4 | 80 | 15 | 90 |
7. | Cu(I) (5) | [bmIm]PF6 | 80 | 15 | 90 |
8. | Cu(I) (5) | [bmim]OH | 80 | 20 | 0 |
9. | Cu(I) (5) | DMF, ACN | 80 | 30 | 25 |
10. | Cu(I) (5) | Toluene | 80 | 30 | 27 |
11. | Cu(I) (5) | PEG-200 | 80 | 20 | 85 |
12. | Cu(I) (5) | CH3CN | 80 | 30 | 15 |
13. | Cu(I)(5) | H2O | 80 | 40 | 20 |
14. | CuCl2 (10) | [bmim]Br | 80 | 35 | 0 |
a Molar ratios: 1:1:1 equivalents of 1a, sodium azide, phenylacetylene.
b Time required to complete the reaction (TLC).
c Yield of isolated and purified products.
A variety of terminal alkynes were chosen and subjected to Huisgen's 1,3-cycloaddition process at 80 °C in IL containing 5 mol% of Cu(I) in 1 g of [bmim]Br. It was found that aliphatic terminal alkynes, such as 1-hexyne, 1-octyne, trimethylsilylacetylene, etc., reacted reasonably with styrene oxide and sodium azide under subjected reaction conditions to produce β-hydroxytriazoles in high yield (Table 2, entries 14, 15,19). The formation of product 3a was mediated through the in situ preferential SN2 attack at the benzylic position by the azide in a regioselective manner. Cyclohexene oxide has also shown equal pace in coupling with sodium azide and phenylacetylene, 1-ethynyl-4-methylbenzene and 1-ethynyl-3-methylbenzene, leading to the formation of N-cyclohexyltriazoles in excellent yield (entries 20,23,24). Further, the stereochemistry of the collected product 3t was exactly in trans as seen in literature records observed from the coupling constants of the ring hydrogens [20].
Synthesis of β-hydroxy triazole scaffolds by click reaction of varied epoxides, NaN3 and terminal alkynesa.
Entry | Epoxide | Alkyne | Product | Timeb (in hr) | Yieldc,d (%) | Reference |
1 | 1a | 2a | 3a | 10 | 95 | [17] |
2 | 1b | 2a | 3b | 12 | 93 | [18] |
3 | 1c | 2a | 3c | 12 | 92 | [18] |
4 | 1d | 2a | 3d | 12 | 93 | [18] |
5 | 1e | 2a | 3e | 8 | 94 | [18] |
6 | 1f | 2a | 3f | 9 | 85 (8:2) | [21] |
7 | 1g | 2a | 3g | 8 | 93 | [17] |
8 | 1h | 2a | 3h | 8 | 95 | [17] |
9 | 1i | 2a | 3i | 6 | 92 | [18] |
10 | 1j | 2a | 3j | 9 | 93 | [18] |
11 | 1k | 2a | 3k | 11 | 92 | [18] |
12 | 1i | 2b | 3l | 10 | 93 | [18] |
13 | 1l | 2c | 3m | 13 | 93 | [21] |
14 | 1a | 2d | 3n | 13 | 87 | [21] |
15 | 1a | 2e | 3o | 10 | 92 | [21] |
16 | 1a | 2c | 3p | 11 | 94 | [21] |
17 | 1m | 2a | 3q | 14 | 90 (8:2) | [21] |
18 | 1n | 2a | 3r | 14 | 92 (9:1) | [21] |
19 | 1a | 2f | 3s | 10 | 91 | [17] |
20 | 1o | 2a | 3t | 10 | 95 | [17] |
21 | 1a | 2g | 3u | 8 | 89 | [17] |
22 | 1a | 2h | 3v | 8 | 89 | [17] |
23 | 1o | 2i | 3w | 10 | 95 | [22] |
24 | 1o | 2j | 3x | 10 | 95 | [22] |
a 1:1:1 equivalents of reactants 1, NaN3 and 2 was taken with 5 mol% of Cu(I) catalyst loaded in [bmim]Br.
b Time taken for completion of reaction (TLC).
c Yield of isolated and purified products.
d All compounds gave C, H and N analysis within ±0.04 and satisfactory spectral (IR, 1H NMR 13C NMR and ESI-MS) data.
Synthetic strategy was further extended to varied epoxides such as propylene oxide, epichlorohydrin, 2-(but-3-en-1-yl) oxirane, 2-(p-tolyl) oxirane, 2-([benzyloxy] methyl) oxirane 2-butyloxirane, and various aryloxy-1, 2-epoxy propane and different types of terminal alkynes such as 1-ethynyl methylbenzenes, ethynylcyclohexane, prop-2-yn-1-ylbenzene, tert-butyl 2-ethynylcyclopentanecarboxylate, tert-butyl (2-methylbut-3-yn-2-yl) carbamate and 2-ethynylpyridine under similar reaction conditions to deliver corresponding β-hydroxytriazoles scaffolds in excellent yields (Table 2). Moreover, the formation of the major regiomers depends on the type of the terminal epoxide used (Scheme 2), and the structures of all the major regiomers were confirmed by comparing the spectral data with that reported in the literature [17,18,21,22].
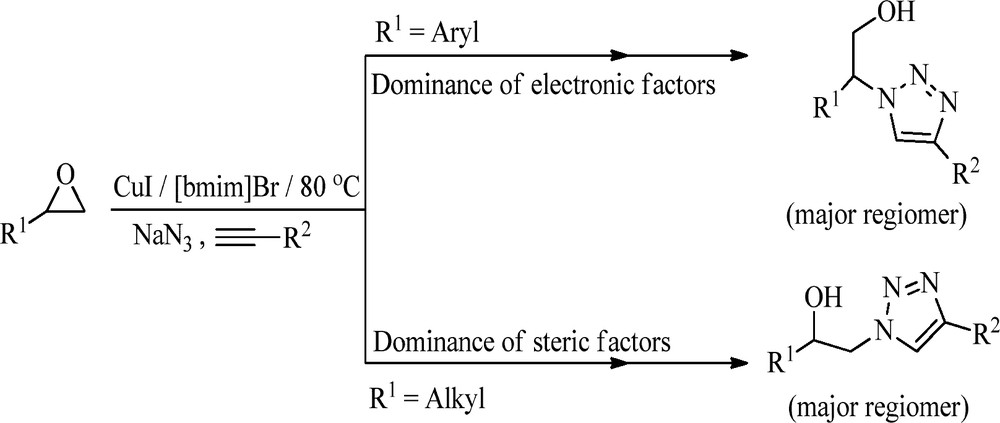
Differentiation of products depending on the nature of epoxides.
Finally, the study focuses on the recycling of the IL containing catalyst for the consecutive reaction runs. Hence, after the work-up, the IL phase that contained Cu(I) was recovered and subjected to the same reaction after drying in a hot air oven at 100 °C for about 20–30 min. It was expectedly found that the isolated yields obtained after the second (93% yield) and third (90% yield) cycles were not much lower than that of the first run (Table 3). However, a decrease in the corresponding percentage yield in case of second and third reaction runs may be observed due to in situ accumulation of Na+ (probably NaOH) during work-up, which tends to deactivate the catalyst. Thus, 1–2 drops of dilute HCl should be added to the IL phase before reducing under vacuum evaporation. Further, successive decreases in the percentage yield in case of fourth, fifth, and sixth runs may be because the regular contact of catalyst with water during the operation might have led to irreversible oxidation of Cu from +1 to +2 oxidation state in the presence of excess chloride ions.
Effectiveness of recycled Cu(I) immobilized in [bmim]Br for click reactiona.
Entry | Cycle | Temp. (°C) | Time (in hr)b | % of yield (3a)c |
1. | 1st run | 80 | 11 | 95 |
2. | 2nd run | 80 | 11 | 93 |
3. | 3rd run | 80 | 11 | 90 |
4. | 4th run | 80 | 15 | 86 |
5. | 5th run | 80 | 20 | 80 |
6. | 6th run | 80 | 25 | 70 |
a Reaction conditions: 1:1:1 equivalents of 1a, sodium azide, 2a and recycled [bmim]Br containing Cu(I) at 80 °C.
b Time required to complete the reaction (TLC).
c Yield of isolated and purified products.
3 Conclusion
This study presents a facile, efficient, and an ecocompatible method for the regioselective synthesis of β-hydroxy triazoles using the click chemistry approach. Operational simplicity, complete regioselectivity, reuse of supported catalyst, excellent reaction output, and moderate reaction conditions are some of the notable features of the envisaged protocol. In addition, this protocol is only limited to terminal alkynes and epoxides. Products with a modest yield were also obtained using internal alkynes and alkynes bearing electron withdrawal functionality (such as acetylene dicarboxylic acid), but it lacked appreciable regioselectivity.
4 Experimental
The chemicals used in the experiments were purchased from Sigma-Aldrich, Himedia and SDFCL and were used as received without any further purification. The melting points were recorded in open capillaries using the ANALAB Melting Point Apparatus. The IR spectra were recorded as KBr discs or as neat, using PerkinElmer FT-IR spectrometer. 1H (400 MHz) and 13C (100 MHz) NMR spectra were recorded as CDCl3/DMSO-d6 solutions with tetramethylsilane (TMS) as an internal standard on a Bruker AVANCE III HD spectrometer. Mass analysis was carried out using Nexera UHPLC @ 130 Mpa with SIL-30AC Nexera auto sampler coupled with an LC-MS 8030 tandem mass spectrometer (Shimadzu Corporation, Tokyo, Japan). All the compounds were analysed in the full scan mode with nitrogen as the interface gas.
4.1 General experimental procedure1 for the synthesis of beta-hydroxy triazoles 3
An equimolar mixture of epoxides 1 and NaN3 was stirred for 10 min in 1 g of IL i.e. [bmim]Br, loaded with Cu(I) at 5 mol% w.r.t. reactant concentration. To the resultant, were added an equimolar ratio of terminal alkynes 2 via a syringe and the reaction was further maintained under stirring at 80 °C for a specified period of time. After completion of the reaction (as indicated by TLC), 10 mL of distilled water were added under continuous stirring, and solid crude product obtained was simply collected by filtration. Purification of crude products was carried out by recrystallization from ethanol. The filtrate containing IL and the Cu(I) catalyst was immediately reduced under low pressure and further dried at 100 °C for 20–30 min in a hot air oven, for the consecutive reaction runs. Physical data of some representative compounds are given below.
4.1.1 Compound 3a
2-phenyl-2-(4-phenyl-1H-1,2,3-triazol-1-yl) ethanol. Pale yellow solid; Mp 125–127 °C (Lit. Mp 125–127 °C) [17]. IR (KBr, νmax/cm−1): 3450, 3070, 2935, 1460, 1370, 1220, 1090, 750, 710, 685. 1H NMR (300 MHz, CDCl3): δ = 8.15 (s, 1H; NCH = C), 7.77 (m, 2H, Ar–H), 7.45 (M, 2H, Ar–H), 7.40–7.28 (m, 6H, Ar–H), 5.77 (dd, J = 8.6, 4.8 Hz, 1H), 4.38–4.14 (m, 2H), 3.38 (brd. s, 1H, exchanges with D2O) ppm. 13C NMR (100 MHz, CDCl3): δ = 149, 137.9, 131.5, 130, 129.5, 129, 128.5, 128, 126.9, 122.1, 68.1, 65.2 ppm. ESI-MS m/z: 266 [M + H]+. Anal. calcd for C16H15N3O: C, 72.43; H, 5.70; N, 15.84%. Found: C, 72.44; H, 5.69; N, 15.85%.
4.1.2 Compound 3s
2-(4-hexyl-1H-1,2,3-triazol-1-yl)-2-phenyl ethanol. Pale yellow solid; Mp 64.5–66.5 °C (Lit. Mp 64.2–66.3 °C) [17]. IR (KBr, νmax/cm−1): 3385, 2927, 2855, 1630, 1447, 1375, 1215, 1065, 772, 672, 535. 1H NMR (300 MHz, CDCl3): δ = 7.65 (s, 1H, NCH = C), 7.45–7.25 (m, 5H, Ar–H), 5.65 (dd, J = 8.8, 4.6 Hz, 1H), 4.43–4.35 (m, 1H), 4.20–4.10 (m, 1H), 3.35–3.40 (brd. s, 1H, exchanges with D2O), 2.46 (m, 2H), 1.65–1.55 (m, 2H), 1.35–1.25 (m, 6H), 0.92 (t, 3H) ppm. 13C NMR (100 MHz, CDCl3): δ = 155, 140, 129, 128.5, 128, 122, 69, 67.5, 35, 32, 26.5, 26.3 ppm. ESI-MS m/z: 274 [M + H]+. Anal. calcd for C16H23N3O: C, 70.30; H, 8.48; N, 15.37%. Found: C, 70.31; H, 8.47; N, 15.36%.
4.1.3 Compound 3t
2-(4-phenyl-1H-1,2,3-triazol-1-yl)cyclo hexanol. White solid; Mp 168.5–170.5 °C (Lit. Mp 167.8–171.8 °C) [17]. IR (KBr, νmax/cm−1): 3460, 3095, 2955, 1530, 1465, 1375, 1290, 1085, 770, 695, 585. 1H NMR (300 MHz, DMSO-d6): δ = 8.64 (s, 1H, NCH = C), 7.80–7.90 (m, 2H, Ar–H), 7.45 (t, J = 7.7, 2H, Ar–H), 7.30–7.37 (m, 1H, Ar–H), 4.70–4.77 (brd. s, 1H, exchanges with D2O), 4.25–4.30 (m, 1H), 3.80–3.68 (m, 1H), 2.20–2.14 (m, 8H) ppm. 13C NMR (100 MHz, DMSO-d6): δ = 149, 132.1, 129.5, 128.1, 123.2, 121.9, 72.1, 66.3, 35.2, 32.2, 25.5, 24 ppm. ESI-MS m/z: 244 [M + H]+. Anal. calcd for C14H17N3O: C, 69.11; H, 7.04; N, 17.27%. Found: C, 69.13; H, 7.06; N, 17.25%.
Acknowledgements
I heartily thank IIIM Jammu, SAIF, and Punjab University, Chandigarh, for providing microanalyses and spectra. I also express my deepest gratitude to Dr. Anil Kumar (Ph. D supervisor) and Dr. Vijai Kumar Rai, for giving me an opportunity to work on MRPs from time to time, sanctioned vide F. No. 39-788/2010 (SR) and F. No. 39-764/2010 (SR) respectively, by UGC – New Delhi.
1 Synthesis of some novel triazolic heterocycles is being carried out by using this green protocol; they may act as potential drug candidates with a wide-range biological spectrum.