1 Introduction
In recent two decades, heteropoly acids (HPAs) with the keggin-type structure have attracted a great deal of interest because of their extensive applications in both acid-catalyzed and redox reactions [1]. However, the application of these catalysts is limited by their short lifetime, low surface area (<102 m2/g), low thermal stability, and difficult recovery process because of their high solubility in water and polar organic solvents [2]. Therefore, serious efforts have been made for the conversion of HPAs to heterogeneous catalysts, which have several advantages over homogeneous systems such as high thermal stability, high surface area, high resistance against degradation, and easy recovery of the catalyst. There are several methods for heterogenization of homogeneous systems of which the most attractive is the catalyst encapsulation in the nanocavity of a suitable support with a certain structure and an appropriate size of nanocavities. Therefore, zeolites, among various supports, are good choices for this purpose [3]. However, zeolite Y has been used by several researchers as a support for immobilization of HPAs because of its appropriate structure [4]. Zeolite Y contains aluminum atoms in its structure and shows basicity, which may disrupt the formation of HPA in the supercage of zeolite or increase the degradation of it. Hence, zeolite Y should be dealuminated [5]. This process not only enhances the stability of the catalyst, but also the secondary mesoporous system would be formed in the zeolite Y matrix for loading of HPA molecules. Therefore, dealumination of zeolite Y is a critical step in heterogenization of HPAs. In the past researches [4a–c], zeolite Y, which was applied for encapsulation of HPA, was dealuminated by hydrothermal treatment while 0.043 mmol/g catalyst loading was performed in the case of molybdophosphoric acid (MPA). Consequently, the zeolite capacity for catalyst loading can be increased by modification of dealumination process.
Tetrahydrobenzo[b]pyrans have attracted a great deal of interest because they are present in a wide variety of biologically active natural products [6] and exhibit several pharmaceutical properties such as antibacterial [7], antioxidant [8], antiallergic [9], potassium channel activator [10], and insulin-sensitizing activities [11]. These compounds have also been known as diuretic, anticoagulant, anticancer, spasmolytic, and antianaphylactic agents [12]. Furthermore, some of 2-amino-4H-pyrans have been applied as pigments [13], photoactive materials [14], potential biodegradable agrochemicals [15], and similarly have been used in the synthesis of tacrine analogues (cholinesterase inhibitors) [16] and blood anticoagulant warfarin [17]. The extensive applications of these heterocycles have promoted a wide variety of studies to explore their synthesis. A straightforward method for the preparation of tetrahydrobenzo[b]pyran is the three-component condensation of aldehydes, β-diketones, and malononitrile, which occurs in the presence of molecular iodine [18], sodium selenate [19], (S)-proline [20], and rare earth perfluorooctanoate(RE(PFO)3) [21]. Ionic liquids [22,23] and ultrasonic [24] and microwave [25] irradiation have also been used for this purpose. However, most of these methods have at least one of the limitations such as low yields of products, long reaction times, harsh reaction conditions, difficult workup procedures, effluent pollution, and the use of complex, inaccessible, toxic, or expensive catalysts. Therefore, development of a mild and efficient method for the synthesis of these valuable heterocycles to overcome previous shortcomings is still a challenge.
Herein, we wish to report the preparation procedure of encapsulated MPA in modified dealuminated zeolite Y (MPA–MDAZY) and its application in the one-pot multicomponent synthesis of tetrahydrobenzo[b]pyrans as a highly efficient and reusable catalyst.
2 Experimental section
All materials were commercial reagent grades and were prepared from Merck or Fluka. Infrared spectra were obtained using a Shimadzu 435-U-04 spectrophotometer (KBr pellets). 1H NMR spectra were recorded on a Bruker AVANCE 300 MHz. Melting points were determined on a Barnstead Electrothermal apparatus. Zeolite NaY was purchased from Sigma–Aldrich chemical company. X-ray diffraction (XRD) powder diffraction was carried out on Advanced Bruker D8 powder diffraction using Cu Kα radiation (λ = 1.54 Å). The amount of Al in the MPA–MDAZY sample was determined by inductively coupled plasma optical emission spectroscopy spectrometer Varian model 730-ES.
2.1 General procedure for the preparation of MPA–MDAZY
Zeolite NaY was dealuminated chemically by means of ethylenediaminetetraacetic acid (H4EDTA). The rate of H4EDTA addition, essential to minimize amorphization of the zeolite, was kept low at 0.01 g of H4EDTA per 1 g of zeolite per 1 h. Dealumination was carried out under stirring at 97 °C. The process was continued for 4 h after all of the acid had been added. The dealuminated samples were washed with hot distilled water, dried, and ion exchanged with 10 wt % ammonium chloride solution [26]. MPA was synthesized into the supercage of MDAZY according to the reported procedure [27]. Two grams of MDAZY and 7.2 g of molybdenum (VI) oxide were mixed in 70 mL deionized water and then stirred for 24 h at room temperature. Then, 0.48 g phosphoric acid (99% purity) was added, and the mixture was stirred for 3 h at a temperature of 90 °C. Next, the synthesized sample (MPA–MDAZY) was filtered off and dried at 110 °C. The samples were individually immersed in hot water. After that they were agitated for 1 h, filtered, and dried at 90 °C. To remove the unreacted species of metal cations in the lattice, the samples were exchanged with an aqueous solution of NaCl (1 M) for 4 h under vigorous stirring. Finally, the catalyst was filtered, washed with distilled water, and dried at 90 °C.
2.2 General procedure for preparation of tetrahydrobenzo[b]pyran derivatives (4)
A mixture of aromatic aldehyde 1 (1 mmol), malononitrile 2 (1 mmol), 1,3-cyclohexanedione 3 (1 mmol), and MPA–MDAZY (140 mg) in ethanol was prepared and placed in a round-bottomed flask and stirred under reflux conditions. After the completion of the reaction as indicated by thin layer chromatography (TLC) (n-hexane/EtOAc, 2:1), the mixture was cooled down to room temperature and diluted with ethanol (10 mL). The mixture was filtered, and the remaining solid material was washed with a mixture of EtOH/Et2O (1:1, 10 mL) and dried for the next use. The filtrate was concentrated, and the precipitate was filtered, washed with cold water, dried, and recrystallized in EtOH to afford pure product in good to excellent yields (Table 1).
Synthesis of 5-oxo-5,6,7,8-tetrahydrobenzo[b]pyrans catalyzed by MPA–MDAZY and MPA–DAZY.
Entry | 1 | 2 | 3 | Product (4) | MPA–MDAZY | MPA–DAZY | Mp (°C) [Ref.] |
Time (min)/yielda (%) | Time (min)/yielda (%) | ||||||
1 | PhCHO | 2a | 3a | 4a | 30/95 | 30/83 | 223–225 [33] |
2 | 2-Cl-C6H4CHO | 2a | 3a | 4b | 50/85 | 70/75 | 213–215 [33] |
3 | 3-Cl-C6H4CHO | 2a | 3a | 4c | 90/88 | 110/80 | 229–230 [34] |
4 | 4-Cl-C6H4CHO | 2a | 3a | 4d | 70/90 | 80/82 | 211–214 [35] |
5 | 2,4-Cl2-C6H3CHO | 2a | 3a | 4e | 60/80 | 95/70 | 118–120 [18] |
6 | 4-Br-C6H4CHO | 2a | 3a | 4f | 25/75 | 40/69 | 208–210 [35] |
7 | 3-NO2-C6H4CHO | 2a | 3a | 4g | 20/90 | 35/86 | 210–212 [36] |
8 | 4-Me-C6H4CHO | 2a | 3a | 4h | 50/50 | 95/50 | 212–214 [18] |
9 | 2-MeO-C6H4CHO | 2a | 3a | 4i | 60/65 | 110/60 | 193–196 [37] |
10 | 3-PhO-C6H4CHO | 2a | 3a | 4j | 45/79 | 80/72 | 190–192 [38] |
11 | 4-Me2N-C6H4CHO | 2a | 3a | 4k | 35/87 | 50/85 | 197–200 [39] |
12 | PhCHO | 2a | 3b | 4l | 15/80 | 25/80 | 213–215 [40] |
13 | 2-Cl-C6H4CHO | 2a | 3b | 4m | 20/75 | 40/68 | 208–210 [40] |
14 | 3-NO2-C6H4CHO | 2a | 3b | 4n | 30/87 | 45/80 | 204–206 [40] |
15 | 4-NC-C6H4CHO | 2a | 3b | 4o | 30/75 | 50/65 | 233–235 [41] |
16 | 4-Cl-C6H4CHO | 2b | 3a | 4p | 20/85 | 55/76 | 152–155 [42] |
17 | 2,4-Cl2-C6H3CHO | 2b | 3a | 4q | 75/70 | 110/70 | 175–177 [43] |
18 | 3-NO2-C6H4CHO | 2b | 3a | 4r | 20/82 | 40/75 | 176–178 [42] |
a Isolated yields.
3 Results and discussion
3.1 Preparation of the catalyst and characterization
In the previously reported works [4a–c], zeolite Y, which was applied for encapsulation of MPA, was dealuminated by hydrothermal treatment. In the present research, the chemical procedure was used for the dealumination process to modify the zeolite Y structure for MPA loading. Next, MPA was encapsulated in MDAZY, and the obtained MPA–MDAZY was characterized by Fourier transform infrared (FT-IR), XRD, and atomic absorption spectroscopy. The catalytic activities of encapsulated MPA in both dealuminated zeolites were compared with each other in the synthesis of tetrahydrobenzo[b]pyrans. The results showed the superiority of the chemical method in the dealumination process rather than the hydrothermal method.
The MPA loading was determined using atomic absorption spectroscopy. On the basis of the obtained results, the amount of catalyst loading was estimated 0.049 mmol/g in MPA–dealuminated zeolite Y (DAZY). In contrast, according to the previous reports [4a–c], the amount of catalyst loading in hydrothermal DAZY was estimated about 0.043 mmol/g. As a result of zeolite dealumination by EDTA treatment, more dealumination occurred, and the extraframework aluminum species were removed from the zeolite structure. Therefore, a mesoporous structure was formed with a larger diameter size (≥1.5 nm) [28]. Consequently, the amount of catalyst loading in the supercage of zeolite was increased [2b]. The Al content of MPA–MDAZY was 282,258 ppm, which was determined by inductively coupled plasma optical emission spectroscopy.
The FT-IR spectra of MDAZY and MPA–MDAZY showed wide strong bonds at 1637 and 3200–3500 cm−1, which are attributed to OH stretching vibration absorption (Fig. 1). The FT-IR spectrum of MPA encapsulated into zeolite confirmed the existence of MPA in the zeolite matrix. A little shift in all characteristic peaks of MPA in the FT-IR spectrum of MPA–MDAZY [observed at 1637, 1405, ˜1040(PO), 945–965(MoOt), 791 (MoOeMo) (Ot, terminal oxygen; Oe, edge-sharing oxygen), 727, 575, and 455 cm−1] reflects the interaction of Keggin-type structure with zeolite Y mostly through corner-shared [27,29]. These results were similar to those that were previously obtained using the hydrothermal synthesis method [30]. The only exception is that in the method used in this research all peaks were observed with high intensity because of the high catalyst loading. On the other hand, all of the adsorption complexes were removed from the zeolite surface; therefore, the presence of infrared bands of MPA in the MPA–MDAZY spectra proved the presence of MPA in the supercage of zeolite, not on the external surface.
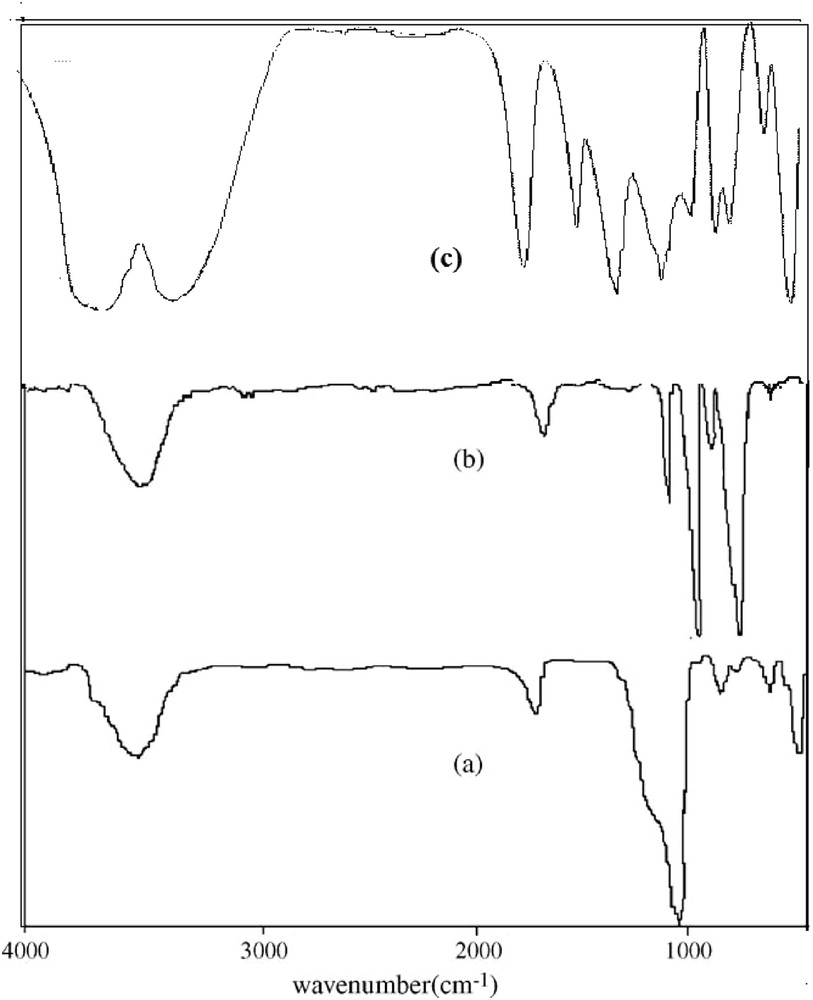
FT-IR spectra of (a) MDAZY, (b) MPA, and (c) MPA–MDAZY.
Fig. 2 shows the XRD patterns of the MDAZY and MPA–MDAZY that have almost identical crystallinity with those of the parent NaY zeolite. Upon dealumination of zeolite NaY with EDTA treatment, the intensity of mean peaks slightly reduces, indicating that the framework of the zeolite structure is still maintained. In addition, in MPA–MDAZY compounds, the intensity of the main peaks of zeolite considerably reduced because of the high amount of catalyst within zeolite cavity. In general, in the XRD pattern of MPA–MDAZY, the disappearance of the corresponding peak of pure MPA (2θ = 7.2) confirmed the encapsulation of MPA in the supercage of zeolite, not on the external surface [29,31]. Finally, peaks in the regions of 220, 311, and 331 reflections were related to the locations of cations in the channels and cages of zeolite. A high-intensity peak in the region of 331 confirmed the presence of encapsulated metallocomplex in the mesoporous structure [32]. In the EDTA-based chemical operation, the more secondary mesopores structure was formed and therefore, the loading of catalyst increased in comparison with the hydrothermal method and consequently, the intensity of main zeolite peaks is reduced in XRD spectra.

XRD patterns of (A) NaY zeolite, (B) MDAZY, and (C) MPA–MDAZY.
3.2 MPA–MDAZY application in the synthesis of tetrahydrobenzo[b]pyran
The catalytic activity of MPA–DAZY was investigated in the three-component synthesis of tetrahydrobenzo[b]pyran from an aldehyde, malononitrile, and dimedone. To aim this, first, the reaction conditions were optimized, and dimedone was reacted with malononitrile and benzaldehyde in the presence of various amounts of MPA–DAZY. Furthermore, the effects of solvent and temperature were evaluated in the model reaction. The best result was obtained using 140 mg (0.686 mol % of MPA) of MPA–DAZY in ethanol at 80 °C, which led to 95% yield after 30 min (Table 2, entry 1). To show the efficiency of MPA–DAZY, the model reaction was performed in the absence of the catalyst and only a poor yield of product was achieved after 4 h (Table 2, entry 2). The result indicated that the presence of MPA–DAZY is necessary for this reaction. The ability of zeolite Y, DAZY, and MPA were compared with MPA–DAZY in the three-component preparation of 2-amino-3-cyano-7,7-dimethyl-5-oxo-4-phenyl-4H-5,6,7,8-tetrahydrobenzo[b]pyran (Table 2). As the results revealed encapsulation of MPA in MDAZY nanocavities increased the catalytic activity of the catalyst.
The effect of encapsulation of MPA in MDAZY nanocavity on its catalytic activity.
Entry | Reagent (reagent amount) | Time | Yielda,b (%) |
1 | MPA–MDAZY (140 mg) | 30 min | 95 |
2 | – | 4 h | Trace |
3 | Zeolite Y (140 mg) | 30 min | 64 |
4 | Dealuminated zeolite Y (140 mg) | 30 min | 55 |
5 | MPA (0.686 mol %) | 30 min | 78 |
a Aldehyde (1 mmol), malononitrile (1 mmol), and dimedone (1 mmol) were used in ethanol at 80 °C.
b Isolated yield.
After optimization of the reaction conditions, this process was expanded to several aldehydes 1, including electron-donating and electron-withdrawing groups, which undergo multicomponent reactions with malononitrile 2 and 5,5-dimethyl-cyclohexane-1,3-dione 3 to afford a range of substituted tetrahydrobenzo[b]pyrans (4a–k) in good to excellent yields (Scheme 1). As shown in Table 1, aromatic aldehydes bearing electron-withdrawing groups (such as nitro or halogens) provide better yields than those bearing electron-donating groups (such as methoxy or methyl). Furthermore, the catalytic activity of MPA–MDAZY was compared with that of MPA–DAZY, which was prepared by hydrothermal dealumination in the synthesis of tetrahydrobenzo[b]pyran derivatives under optimized reaction conditions. As shown in Table 1, in all cases the activity of MPA–MDAZY was more than the activity of MPA–DAZY, which was reflected in less reaction times and more yields of products when MPA–MDAZY was used. Hence, chemical treatment in the dealumination process of zeolite Y desirably enhanced the catalytic activity of encapsulated MPA in the zeolite matrix as a result of increase in MPA loading in zeolite pores.

Synthesis of 5-oxo-5,6,7,8-tetrahydrobenzo[b]pyran derivatives catalyzed by MPA–MDAZY.
3.3 Chemoselectivity of the catalyst
It is important to note that the selective synthesis of mono- and bis-tetrahydrobenzo[b]pyran from terephthalaldehyde can be achieved by the developed method. The reaction of terephthalaldehyde with equimolar amounts of malononitrile and dimedone under optimized conditions selectively produced mono-tetrahydrobenzo[b]pyran in 89% yield after 15 min. Although, similar reaction of terephthalaldehyde with two equivalents of malononitrile and dimedone led to 87% bis-tetrahydrobenzo[b]pyran after 40 min (Scheme 2).
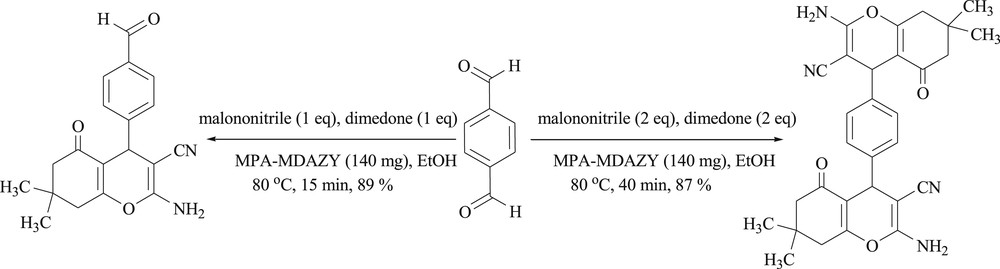
Selective synthesis of mono- and bis-tetrahydrobenzo[b]pyran.
3.4 Reusability of the catalyst
The reusability of MPA–MDAZY was investigated in the synthesis of tetrahydrobenzo[b]pyran from benzaldehyde, malononitrile, and dimedone. After completion of the reaction, the catalyst was separated by simple filtration, washed with 1:1 mixture of EtOH/Et2O, completely dried at 120 °C for 3 h, and reused in the model reaction (Table 3). The results indicated that after 10 runs, the catalytic activity of MPA–MDAZY was not significantly reduced, which confirmed the existence of MPA in the supercages of zeolite, and no leaching of initial MPA occurred during catalyst recovery and reuse.
4 Conclusions
MPA–MDAZY was prepared by chemical dealumination of zeolite Y and encapsulation of MPA in the zeolite matrix. Furthermore, it was shown that the chemical method increases the dealumination and subsequently, the MPA loading is enhanced in zeolite Y nanocavities. Therefore, the catalytic activity of MPA–MDAZY is significantly more than MPA–DAZY (in which zeolite Y is dealuminated by hydrothermal procedure). MPA–MDAZY can serve as an efficient heterogeneous catalyst for the synthesis of tetrahydrobenzo[b]pyran derivatives by the condensation of aldehydes, β-diketone, and malononitrile in ethanol. This methodology has some advantages such as short reaction times, operational simplicity, high yields, selectivity, and use of MPA–MDAZY as a highly efficient, stable, nontoxic, safe, reusable, and environmentally benign reagent.
Acknowledgments
The authors are grateful to the research council of Hakim Sabzevari University for partial support of this work.