Version française abrégée
Le rapport isotopique de l'oxygène des groupes carbonate et phosphate de l'émail dentaire (
Nous avons étudié les variations de la composition isotopique de l'oxygène des carbonates (

Location map of the four Miocene sites.
Carte des quatre sites étudiés du Miocène du bassin d'Aquitaine (SW France).
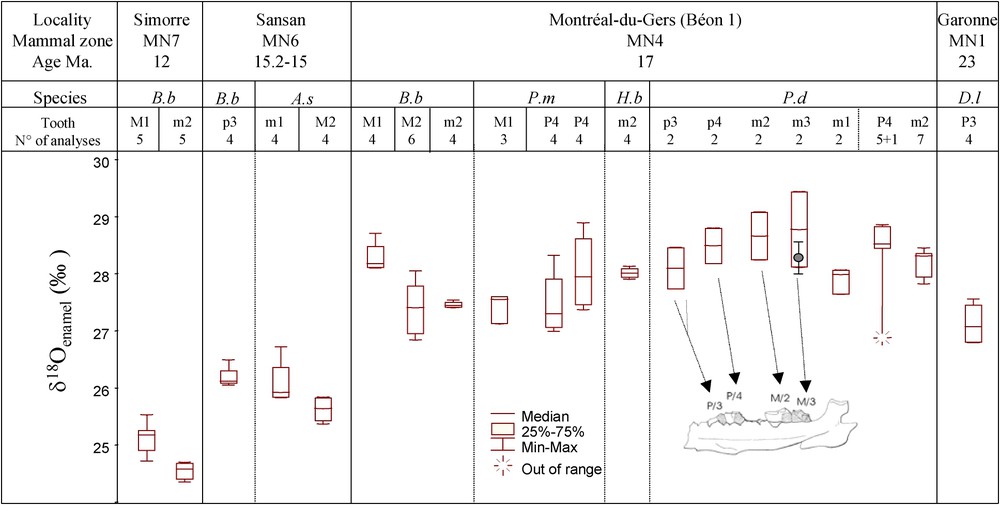
Box plots of the 20 mean teeth
Box plots of the 20 mean teeth
Les valeurs de
Entre 18 et 12 Ma, la déplétion isotopique observée dans le bassin d'Aquitaine, situé à la paléolatitude 40°N [32], peut être liée à une baisse de la température de l'air si le montant des précipitations est resté stable [36]. Selon la relation de Dansgaard (pente de
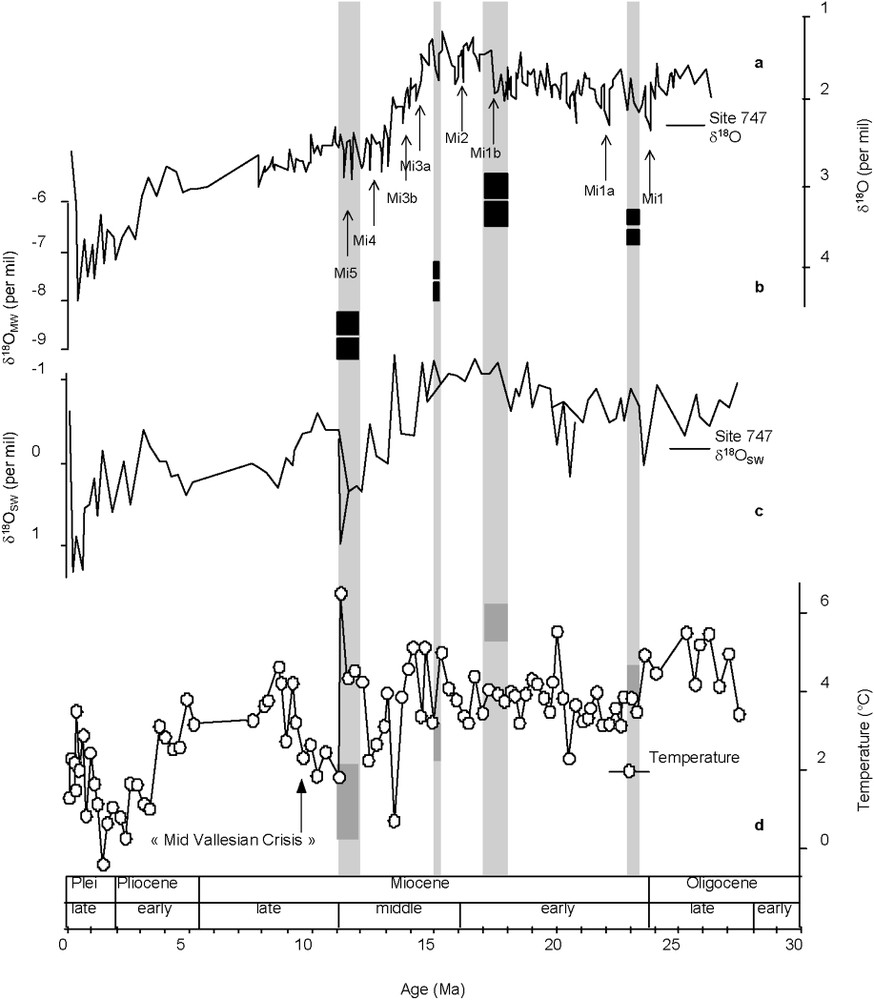
Following Billups et Schrag [2]: (a)
Modifiée d'après Billups et Schrag [2] : (a)
Modifiée d'après Billups et Schrag [2] : (a)
1 Introduction
Carbonate and phosphate oxygen isotope ratios of mammal tooth enamel (
In this study, oxygen isotopic compositions of enamel carbonate (
2 Sampling and analytical techniques
Four Miocene mammal localities in Aquitaine (France) were studied (Fig. 1): Grenade-sur-Garonne (MN1;
West European palaeoenvironments were affected by two major tectonic events between 17–14 and 9–8 Ma [28]. During the Early and Middle Miocene, the western part of the Aquitaine region has been invaded by the sea several times [19], and the four localities were close to their contemporary coastline. The ‘Astarac region’ (MN7) faunas are characterized by the co-occurrence of B. brachypus and A. simorrense. The environment was most likely a wet forest. At Montréal-du-Gers (MN4), fossils were collected from clay beds representing a wet paludal and/or lake-side environment, surrounded by a wooded savannah-like biome where H. beonense, P. mirallesi, B. brachypus, and P. douvillei occurred [38]. In this locality, a jaw with an incomplete tooth row (two premolars and two molars) belonging to an adult P. douvillei was studied. A single specimen of D. lemanense found in Grenade-sur-Garonne (MN1) was sampled.
Between 2 and 7 fragments were analysed for each tooth, giving an indication of intra-tooth variability.
Diagenesis tests were used in order to check both the crystallinity and isotopic integrity of the fossil teeth. Scanning Electron Microscopy observations showed that crystalline structures are preserved. The constant crystal index for the fossil teeth and full width half maximum as measured by X-ray diffraction (XRD) analysis [30] show that all of the fossil teeth (except the P4 [P. douvillei, MN4] and the P3 [D. lemanense, MN1]) are well preserved. PO chemical bonds in apatite are more resistant to alteration process than the CO bonds [34,37], so phosphate oxygen isotopic composition (
The teeth were drilled either on appropriate parts of broken surfaces of the same tooth or along the labial or lingual surface of a loph(id) perpendicular to the occlusal plane. Eighty isotopic analyses were performed. Five milligrams of powdered enamel were obtained using an agate mortar and pestle, sieved through a 125-μm mesh. Organic matter was removed using standard procedures [11]. Washed and treated enamel powder (1.5–2 mg) is acidified with phosphoric acid (103%) releasing CO2 gas for isotopic analyses using a Micromass Optima mass spectrometer.
3 Results and discussion
The results of the isotopic analysis are presented in Fig. 2, together with the provenance, species and number of analyses for each tooth. The box-plots exhibit a general decrease of the
3.1 Intra-individual variability: P. douvillei
No significant trend (Fisher test:
3.2 Inter-species variability
The average
The species recovered from Montréal-du-Gers lived in different contemporary ecosystems: swamps (B. brachypus and P. douvillei), open woodland (P. mirallesi) and savannah-like open environments (H. beonense). The similarity of the
3.3 Variability with respect to the geological age
The
In order to isolate the effect of the geological age on the variability of the
3.4 Isotopic shift and temperature changes
If we assume that the observed isotopic shift is mainly a temperature effect at this palaeolatitude of about 40°N [32] and that the precipitation amount remained stable throughout the period of our study, as shown for the Neogene of western Europe [36], then we estimate – using Dansgaard's calibration of
In order to reconstruct more precisely Tertiary palaeoclimates, we will have (i) to cross our geochemical data with other independent continental proxies (fauna, pollen), (ii) to increase significantly the time resolution sampling of the continental records, and (iii) to reconstruct quantitatively the changes in terms of seasonal distributions [17].
Acknowledgements
We thank Mr R. Guiraux (teeth preparation and technical advice) and Mr D. Excoffon (XRD and SEM analyses), Dr. S. Papadimitriou and Dr D. Kroon for comments on a preliminary draft. This is the ISEM contribution No. 2005-093.