1 Introduction
Physico-chemistry offers efficient tools in the field of normal and pathological biomineralizations or nano-inorganics concretions which are complex to identify [1].The obtained data are discriminant for diagnosis, guiding the design specific therapeutic strategies [2]. Furthermore, physico-chemistry provides insights and breakthroughs in biology. Evidence of specific mineral morphology or composition may be evidence of physiological or pathological mechanisms. These mechanisms reveal conditions of the mineral formation. Minerals may show singular morphologies or surface states that prove an identified origin or cause for initial nucleation, such as the bacterial activity as the initiator of calcifications in prostatic stones [3].
Biominerals may be observed under “solid state” methodologies. In this domain, enamel is the best inorganic candidate for characterization by Materials Science techniques. Enamel is exemplary for calibrating physicochemical investigations. Indeed, enamel is the most mineralized biological tissue (97% mineral) and based on a non-collagenous matrix scaffold in contrast to bone or dentine.
Different from the two previous mineralized tissues, enamel loses its capacities to regenerate after the end of its mineralization. Consequently, the decay of enamel exposes patients to several complications. At the end, a definitive loss of normal dental behavior (mechanic, chemical endurance, etc.) may occur. Heavy and expensive surgeries could be the lone therapeutic issues. Additionally, prevalence of dental diseases has increased over the past 20 years. According to the World Health Organization [4], an estimated 5 billion people worldwide suffer from dental caries and in industrialized countries, the treatment of dental diseases accounts for 5–10% of total health care expenditure and remains a financial burden for societies.
The present review summarizes the last ten years of data on enamel based on physical, chemical, and materials characterization methodologies. This review is addressed to both the medical community and physicists. Each technique will be presented with the same design: basic science principles followed by a summary of its late applications in biology and enamel.
2 Biological patterns of enamel and amelogenesis
Enamel is original because of its highly organized crystalline architecture is preceded by a labile scaffold [5]. Enamel is made of 97% of mineralized calcium phosphate, namely hydroxyapatite (HAP), the remainder consisting of 3–4% of water and residual protein [6,7]. Amelogenesis results from the secretion of an acellular matrix by epithelial cells, the ameloblasts. Mineralization is completed during the maturation stage of ameloblasts which intervene in several ways by controlling the availability of ions for growing minerals while degrading the extracellular matrix scaffold.
Two structures, the intraprismatic and interprismatic enamel, are imbricated. With the same composition, they differ in their crystal orientation. The major component of intraprismatic and interprismatic enamel is hexagonal HAP crystals, space group symmetry P63/m, with lattice parameters a = 9.43 Å and c = 6.88 Å [8]. The basic unit of the enamel is HAP crystal of formula Ca5(PO4)3(OH) and presents numerous substitutions of Ca2+ and PO43− [9]. In a biological context, the crystal surface is modulated by enamel matrix proteins (EMPs) chelation which controls the surface energy and crystal growth [10].
At first, crystals form mineral nanofibrils which align lengthwise and aggregate into fibrils which themselves will form thicker fibers. Fibrils and fibers will assemble in the intraprismatic and interprismatic enamel. Prisms present differing arrangements along the enamel thickness, which gives strong mechanical and physical properties to enamel continuously aggressed in the buccal environment. The boundary between intraprimastic and interprismatic enamel is a narrow space filled with an organic material and called the prism sheath. At both the internal and external limits of enamel, an aprismatic layer in which all crystals are parallel is described [11].
Several EMPs play a major role in enamel, notably three major species, amelogenins (AMEL), ameloblastin (AMBN), and enamelin (ENAM).
AMEL (90% of the total proteins) is a family of isoform proteins due to numerous alternative spliced RNAs. These proteins may be cleaved into different peptides in order to regulate further mineralization. They may be degraded by the extracellular proteolysis from specific enzymes. These peptides have a preponderant role in amelogenesis [12]. They are key mineralization proteins via the formation of labile nanospheres on the mineral surface. These nanospheres regulate the development of crystals and form a Ca2+ and PO43− ion reservoir. Thus, the amount of amelogenin peptides control the thickness of enamel and their quality and supramolecular organization drive the crystal and prism pattern.
The second major peptide, AMBN (5% of total proteins) is expressed throughout amelogenesis. Located at the boundary between intra- and inter-prismatic enamel, AMBN has also been called sheathlin [13]. AMBN ensures the supracrystalline organization of enamel prisms and the initiation of mineralization [14,15].
ENAM (1% of the total proteins) is the immediate environment of the crystal, responsible for its growth and elongation [16]. The native protein inhibits the crystal growth while ENAM proteolytic products promote the formation of apatite, due to their association with amelogenins.
Knowledge on the mechanisms through which EMPs self-assemble, organize and modulate the crystal growth in a coordinated manner is progressively emerging. In general, hydrophobic domains are known to be responsible for the scaffold of the matrix, while their hydrophilic counterparts are involved in the regulation of crystal nucleation and growth. AMBN and ENAM bind AMEL nanospheres via lectin-type domains and therefore stabilize amelogenins within the matrix.
In summary, macromolecular self-assemblies of AMEL, AMBN, and ENAM control enamel morphogenesis and participate in the control of mineralization.
In the development of teeth, long and thin mineralized enamel beads are formed almost simultaneously with EMP release in the extracellular compartment. These “mineral cords” are extremely long (>100 microns) and their initial diameter minimal (10 nm). This incipient mineral phase occupies 10–20% of the enamel volume. The major axes of these crystals are co-aligned and arranged parallel with oblique or longitudinal directions in intra- and extra-prismatic enamel, respectively [17].
To lead to mature fully-mineralized enamel, gradual differentiation of ameloblasts in effector/secreting cells is necessary. The first stage is secretion in which ameloblasts export the bulk of EMPs required for the deposition of the different layers of enamel: the secretion begins with the internal aprismatic enamel. Ameloblasts migrate allowing Tomes' process to grow and produce interprismatic and intraprismatic enamel. They secrete the external prismatic enamel and finally, the last outer aprismatic enamel [18]. The second stage is enamel maturation. The ameloblasts produce active extracellular proteases, metalloproteinase MMP20 and kallikrein 4 KLK4 which cleave EMPs. Their proteolysis and removal from the matrix permit the lateral growth of the crystal [10,19].
3 Polarized light microscopy
Light Microscopy measurements are very common techniques and widely available in laboratories. This is also a preponderant technique in biological tissues. Polarized light microscopy allows the specific observation of birefringent structures. Birefringence is the optical intrinsic property of a material measured by using its refractive index that depends on the polarization and propagation direction of light. Between crossed polarizers, birefringent compounds will be lighted while the others will be dark. The birefringence value is the difference between the refractive index in the direction of light propagation and the refractive index perpendicular to this direction.
Apatite is a weakly negative uniaxial birefringent mineral (0.007). However, studies of the ultrastructure of enamel have shown that the birefringence value is more complex to determine and the result of different optical contributions, intrinsic mineral values, mineral organization contributions, water influence and non-mineral matrix contributions [20]. Recently, Sousa et al. have described a new model of calculation for the interpretation of observed enamel birefringence [21]. Arnold et al. [22] used polarized light microscopy to show the early organization of enamel prisms in developing teeth. The enamel birefringence was significant in their samples.
Birefingence properties are very sensitive to any modification of the organization within enamel. Youravong et al. [23] have shown by this method in 2005 that lead contamination had a significant impact on enamel mineralization. Even if enamel appeared clinically normal, it was hypomineralized as shown by clear modifications in enamel birefringence. The principal cause was proposed to be the acidic environment during the tooth growth. Enamel was less resilient to acidic challenges and the patients seemed to harbor a high carious risk. Crombie et al. [24] proposed in 2013 a study on Molar-Incisor Hypomineralization (MIH). This pathology may be induced by a contamination by endocrine disruptors during the perinatal period and affects molar enamel with, in some cases, an expression on the incisor too. This study had shown that the highly variable nature of MIH affected enamel (hardness, porosity, mineral content, etc.). Polarized light microscopy permits a very clear estimation of the affected enamel volume and offers the possibility to perform further investigations with high precision.
The carious process modifies the status of mineral in enamel and may increase the contributions on optical properties of non-mineral and aqueous entities. Direct interpretations of modification of the enamel birefringence allow the detection of structural modification by clinical observations. Indeed, the different contributions on the whole enamel birefringence value are strongly dependent of the mineral state.
Caries management requires an early intervention that is before significant mineral loss. Meharry et al. [25] and Lo et al. [26] present in their recent studies the advantage of polarized light microscopy in the evidence of early enamel decay. The polarized light microscopy enabled its detection by the modifications in enamel birefringence.
This microscopy offers an accessible methodology but this technique is not easily quantitative and presents a lack of accuracy. Other physicochemical techniques are necessary in order to investigate enamel.
4 Electron microscopy
Electron microscopy gives access to nanostructural observation. By contrast to optical microscopy, the probe is a focused electron beam accelerated by electromagnetic coils. Several interactions between this beam and matter may occur.
The observations may be performed through the sample. These methodologies are available by transmission electron microscopy (TEM). In this case, the quantic nature of electrons (both particle and wave) is used. The wavelength of an electron depends on its speed. The wavelength limits the spatial resolution of an image. Since the first commercial electron microscopes (late 1930s) [27], improvements have been obtained for magnetic electron acceleration devices. These technical refinements allowed increase of resolution. Electrons may be partially absorbed by the sample. In this case, a direct image is obtained at high magnification on the detector. As waves, electrons may be diffracted by a crystallized compound. This diffraction phenomenon gives access to the crystalline phase.
Electrons may also interact with matter by electronic phenomena from surface atoms. Secondary electrons are generated after interactions between the incident electron beam and the surface atoms. Conduction band electrons may receive a small part of the beam's energy and are ejected outside the surface. As these electrons come from the superficial part of the sample, they are very sensitive to any variation of orientation. Therefore the detector allows us to reconstruct the sample topology.
Back-scattered electrons result from a second type of interaction. This time, the electrons interact with the atomic nuclei. Probe electrons are reflected by elastic scattering in a direction very close to the incident beam. Heavy elements provoke a more intense scattering than lighter elements. Thus, the resulting image will be strongly dependent on the atomic weight of the atoms within the sample. This methodology allows composition estimation.
Finally, electrons may induce ejections of the inner shell of the atom and provoke the apparition of a hole. In this case, an electron from the outer shell would fill the hole and release an X-ray photon. This photon has a specific energy (dependent on the element and the transition). Energy-dispersive X-ray spectroscopy (EDS) consists of studying these X-ray photons and allows a detection of the chemical elements of the sample. Chemical maps are thus possible, thank to a detection in phase with movement of the electron beam. Fig. 1 shows chemical maps of a carious tooth. We observed a clear difference of intensity between the normal dentine and enamel. The high mineralization of enamel is demonstrated by a stronger signal of calcium and phosphorus in enamel than dentine. In dentine, the increase of the carbon contribution is attributed to the proteins. Carious propagation in dentine and enamel induces rapid loss in calcium and phosphorus, landmarks of the demineralization. The increase of carbon signal in carious dentine is provoked by organic matters which fill the empty volume of demineralization.
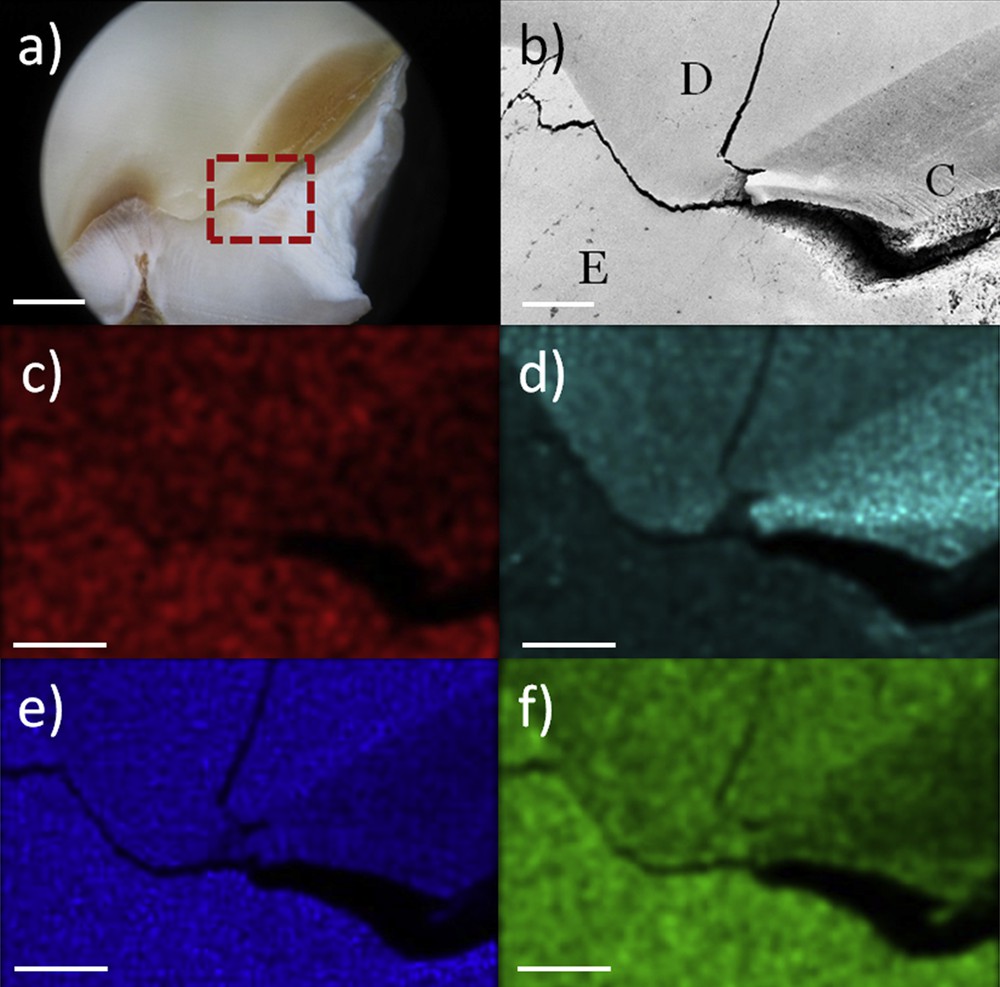
a) Optical image of the enamel-dentine junction in a carious tooth (scale bar: 1 mm), red square area is observed by SEM: b) secondary electrons image of the junction (E: enamel, D: dentine, C: carious dentine), c) calcium map (Kα 3.688 kV), d) carbon map (Kα 0.277 kV), e) oxygen map (Kα 0.524 kV), f) phosphorus map (Kα 2.012 kV) – (scale bar: 80 μm).
4.1 Transmission electron microscopy (TEM)
Transmission electron microscopy offers the opportunity to study both organization and composition of enamel prisms. Consequently, this technique has been the first choice for the study of enamel diseases since several decades. In the early 1950s, Matsumiyas et al. proposed the first study by TEM of carious enamel [28]. In 1967, Johnson described the structure of carious enamel and showed the conservative structure of apatite [29].
Successive improvements in detection systems allowed more precise images and intimate characterization. Ultrastructure of enamel was described by Frank et al. [30]. Pictures of imbricated structures were proposed and the enamel prisms were individually described. Observations by Newman et al. on outer enamel had allowed an explanation of the singular morphology of the enamel prism by an imperfect synchronization of two or more circadian rhythms [31].
In the past 10 years, several studies on the structure and the morphology of enamel have been performed with the support of TEM experiments. Yanagisawa and Miake [32] showed in 2003 the natural mechanism of demineralization/remineralization of enamel in oral cavity. In this paper, the authors published high-resolution images of enamel sections which proved the rhombic unit cell and they demonstrated the perforation of enamel crystallites by the center during carious process. A pathological defect on mineralization, MIH, was studied by TEM by Xie et al. [33]. For this pathology, TEM observations showed that the prism structure of enamel was less dense with wide empty structures. The authors considered that the mechanical properties would be reduced by these structural modifications.
The hierarchical assembly in enamel was described by Cui and Ge [9] in 2007. They described by TEM, scanning electron microscopy (SEM) and atomic force microscopy (AFM) the different levels of organization of enamel, from the individual crystal of hydroxyapatite to the enamel layer.
In 2009, Beniash et al. [34] proposed an elegant study on the transformation of amorphous calcium phosphate into apatite during the early stage of amelogenesis. Based on a mouse model, the authors suggested that EMPs moulded early enamel rods before the crystallization of the amorphous apatite. This study enforces the concept that enamel proteins are major players in the mineralization process.
4.2 Scanning electron microscopy (SEM)
SEM is the main technique used in oral science (more than 1200 articles on PubMed over the past ten years with 100–140 articles per year). This technique gathers easy preparation (optical or electronic transparency not mandatory) and is of wide access in research laboratories. The quick measures allow the imaging of enamel with high precision. Several pathologies have been analyzed by SEM [35,36].
The first observations with secondary electrons were performed on a SEM prototype by Boyde and Stewart [37] in 1963. Boyde and Jones [38] were the first to use backscattered electrons in order to illustrate dental tissues.
Carious decay was a major and early subject of interest [39]. Each technical improvement allowed the refinement of the understanding of mineral dissolution and reprecipitation [40–42].The authors [41] proposed a study of the dissolution of enamel with strict control of the environmental ionic concentration. The current theory was that the dissolution was favored in undersaturated solutions. But this article proposed another theory based on a size effect, where enamel has a singular endurance to dissolution when the crystal size approached the nanoscale. They confirmed their theory by complementary observations by AFM and EDS.
Tooth bleaching has become a common and popular esthetic procedure. Bleaching is an easy method to wash the sample surface. Effects and consequences of this practice are regularly investigated. Miranda et al. [43] showed that bleaching deeply affected enamel with different consequences: cavities, holes, and porosities. These modifications may have severe impacts on oral health. Several studies [44–46] are focused on the real impact of different bleaching agents. Improvements of each procedure were compared to the microstructure of enamel observed by SEM. The enamel decay is quite different in function of the bleaching technique, however each time the enamel showed evidence of defects in enamel which are consequences of these procedures.
5 X-ray diffraction (XRD)
X-ray diffraction allows the determination of a chemical phase and the estimation of crystallinity. Comparison with reference samples allows us to determine with precision the characteristics of a material. More information may be obtained by X-ray diffraction. The mean size of crystallites may be calculated by using the Scherrer Formula with some approximations of the morphology of these crystallites. Furthermore, an X-ray diffraction pattern may show the texture of the material, i.e. the organization of the crystallite itself in space. Improvements of X-ray generators and optics allow an increase in the signal quality. The probe size may be smaller without information loss and this reduction permits a selective analysis of the material. The increase of signal-noise ratio, for example by the use of synchrotron radiation, allows the possibility of rapid and efficient scanning of chemistry and orientations of mineral.
Early physical studies on teeth show the huge interest for these samples [47–49]. Teeth are highly mineralized and permit a well defined pattern. The hydroxyapatite was described as the main chemical phase of enamel. High crystallinity of this phase was also confirmed. Enamel is a highly anisotropic biological mineral. Laboratory experiments showed that patterns were very well defined and rings were thin (Fig. 2). Compared to dentine, the enamel crystallites were well aligned and organized in short range, as shown by textures of rings in enamel's diffractrogram. The widths of peaks in the radial integrations suggested that enamel crystallites were larger than dentine crystallites.

Typical XRD patterns: a) Enamel, b) Dentine, c) Radial integrations. Data collections were performed at 295 K with a MAR345 Image Plate detector by using a monochromatic wavelength (λCu Kα = 1.541 Å) at a sample-detector distance of 300 mm.
X-ray diffraction has often been used for the characterization of carious enamel. Gutierrez et al. [50] in 2005 tried to evidence correlation between caries risk and XRD results. No correlation was observed, but the authors showed a possible link between enamel micropore form and carious disposition after a study by small-angle X-ray scattering (SAXS). Comparable methodology was used by Yagi [51] in order to characterize the enamel near early carious lesion. They demonstrated that enamel in early caries would be included in voids between nanorods. These voids were detected by SAXS. In the same study, even if the XRD patterns were similar, normalizations showed significant signal loss in the carious area. Effects of remineralization in response to the carious phenomenon were observed by Tanaka et al. [52]. The results of XRD and SAXS showed the reverse phenomenon during remineralization and confirmed the probable link between void creation and caries. Recent study by Egan et al. [53] showed significant results for the detection of caries in teeth. They used high energy X-ray to obtain tomography of a carious tooth. They correlated tomography images and XRD of different parts of the tooth to obtain a 3D map by crystallite sizes. These maps permitted a localization of the enamel decay within the tooth. As tomography is a current methodology in clinical practice, this recent evolution may end with a direct detection of carious volumes inside teeth.
Reyes-Gasga et al. [54] proposed in 2012 a study by XRD and electron diffraction in order to solve the real crystallographic structure of enamel. Indeed, the hydroxyapatite unit cell may be hexagonal [8] or monoclinic [55]. This last structure seems to have improved thermodynamic stability. This study showed the hexagonal organization of enamel, even if the monoclinic form would stay more stable.
Another parameter of enamel, the orientation of crystals, may be followed by XRD. Recently, Raue et al. [56] proposed an explanation for the different textural observations in enamel. They supposed that the unit crystallographic cell may form different stacked organizations along the c-axis. They excluded a regular and dense stacking and proposed two different organizations: unit cells are rotated around the c-axis during stacking; or unit cells are stacked in the same orientation and their arrangement is not ordered. This indecision is solved by using X-ray beam size smaller or equal to the unit cell size. The open question of orientation of mineral crystals at enamel surfaces was studied by Fujisaki et al. [57]. They designed an evaluation of strain of apatite by measurements with XRD and modification of the (004) peak along the c-axis. They demonstrated that the incisor showed a tensile strain, while a posterior tooth showed a more complex strain pattern, varying with the measurement site. This paper offered a new way to diagnose any modification of the mechanical behavior of enamel. A comparable aim was explored by Eimar et al. [58]. They correlated measures of enamel hardness with XRD evaluation, for mineral organization and phase, and infrared spectroscopy, for the estimation of the organic content. They proved that hardness is not dependent on the organic phase but is strongly associated with the size of crystallites along the c-axis. This correlation has been attributed to a polycrystalline material behavior following the Hall–Petch model. This model explains that the optimal size of apatite nanocrystals provides enamel with the greatest hardness and sustainability over a human lifetime.
6 Multiple technique results
Several other techniques (Fourier-Transformed Infrared spectroscopy-FTIR, Raman Spectroscopy, X-ray Fluorescence, etc.) may be used for specific investigations in enamel. Some techniques may be partially destructive. For example, FTIR of enamel may require the preparation of a pellet of the sample into KBr. The sample must be diluted and destroyed. If we have favored easily producible and accessible techniques in this review, their uses offer opportunity to resolve questions around modifications on the composition or morphology. This part summarizes bibliographical data of these last few years on physicochemical techniques scarcely used alone, because they may give incomplete or uncertain results or may be hard to manage.
A multi-technical study in 2013 [59] proposed a routine measurement of crystallinity both XRD and Fourier Transform Infrared of sound enamel teeth. The crystallinity index is a discriminant value in bone and dental science. The authors made TEM and SEM observations in order to correlate their calculations with enamel morphologies. This paper proved that the both techniques offered reliable estimations of this index and evidenced that these methodologies are equivalent.
A recent study [60] proposed a characterization of deciduous enamel in terms of morphology, composition and structure. The authors used XRD, SEM, EDS, FTIR and X-ray Photoelectron Spectroscopy (surface specific technique). Two patterns were described for the prism organization. Statistical differences were shown for the elemental ratio Ca/P between the inner and outer part of enamel. These results suggested the coexistence of two mineral phases, hydroxyapatite and carbonated apatite, in deciduous enamel.
These characterizations of the sound enamel allow comparisons with pathological enamel. Sa et al. [61] proposed a compositional, structural, and mechanical study of genetic hypomature enamel. Hypomature enamel may present several clinical issues, as modification of color or decrease of mechanical properties. These parameters were studied by SEM, EDS, XRD and Raman Spectroscopy. The mechanical behavior was specially studied by micro-computed tomography and Vickers microhardness. The decay of physical properties was attributed to the retention of the organic phase during amelogenesis. This excess induced modification of the spatial repartition of apatite and led to a hypomature enamel. These results could also explain the difficulties that dentists may experience during the clinical management of these teeth. Indeed, the excess of organic compounds could modify the adhesive behavior of the tooth during the prosthetic therapy.
7 Synchrotron radiation (SR) techniques
SR is a radiation which is produced by ultrarelativistic (i.e. with a speed close to the light) charged particles when their direction is modified by magnet fields. This radiation is characterized by a high flux (or intensity of photons), high brilliance (small divergence of the beam) and a broad spectrum, form microwaves to hard X-rays.
The first synchrotron was designed in 1946 and emitted visible light. The correlation between the radius of the device and energy of the emitted beam was directly demonstrated. However, in the beginning, this radiation was considered as a parasitic mode. In the 1960s, the first dedicated devices were built in order to use SR. Successive generations of light sources were designed, which led to an increase of brightness (number of photons through a section). Current sources, the third, offer a beam brightness between 1016 and 1020, more than 1013 brighter than conventional X-ray tubes.
A precise selection of energy range allows us to obtain a high-collimated and brilliant monochromatic beam. This probe may be used in spectroscopic devices. The excellent ratio signal–noise allows the reduction of the beam size without loss of the resolution. Several techniques were already used in different pathological calcifications [1].
Synchrotron radiation was recently used by Al-Jawad et al. [62] for the study of enamel. This work showed with high resolution the preferred orientation of crystallite in enamel and an evolution of lattice parameter through the enamel, i.e. a difference of crystallinity. This last result suggested chemical modifications in apatite within enamel. The same team confirmed these results in 2011 and 2013 [63,64]. After an analysis by Rietveld refinement, they suggested that high crystallinity may have an evolutionary origin in line with mastication requirement and that the organization of crystallites in enamel would be longer than the mineralization. Similar results on the crystal orientation were found by Xue et al. in 2008 with a laboratory diffractometer [65]. This study proved the availability of structural information with common laboratory equipment and the possible transposition of SR results to the laboratory.
As said previously, one of the advantages of SR is the possibility to use a small probe without loss of flux or brilliance. This characteristic allows a precise localization of trace elements detected by X-ray fluorescence (SR-XRF). Harris et al. [66] used SR-XRF for imaging the localization of copper and zinc in carious teeth. They showed that localizations of these elements were strongly linked to the carious state and proposed different origins for copper (bacterial, extra-buccal or from the pulp). Estimations of trace elements in enamel were also proposed by Souza-Guerra in 2014 [67]. The authors showed in their preliminary study the spatial repartition of Ca, K, Zn, Pb, Mn, Cu, and Sr in different parts of the enamel. They found a high rate of pollutant ions (Pb, Mn) in the superficial enamel. Moreover, they showed the chemical heterogeneity of enamel and its variation of composition in trace elements.
Trace elements may be implicated in the suspected remineralization process. As shown by Matsunaga [68], zinc was studied on its effects on reprecipitation of apatite. The authors used Extended X-ray Absorption Fine Structure (EXAFS) on the Zn K-edge. This technique, often only available with SR, offers the opportunity to determine the environment of a probe element. By studying of the distance between Zn and O, the authors showed that Zn was incorporated into the apatite structure newly remineralized. The preponderant role of Zn in mineralization of apatite was also described in bone by the same team [69], more precisely in matrix vesicle-mediated mineral deposition.
The highly brilliant beam of SR allows the use of the X-ray computed tomography (CT) in order to obtain 3D representation of teeth without cutting. This technique consists of calculations from serial pictures obtained after short X-ray irradiation a volume representation. CT is commonly used in private practice as complements of radiology. SR-CT permits highly resolved reconstructions where the scientists may study modification of density, mineralization or organization within enamel in a whole uncut sample. This technique was often used for studying of archeological teeth [70,71]. The authors underlined the difference of dental development between modern men and Neanderthals. If these studies did not entirely answer to the evolutionary mechanism responsible to this difference, they showed preliminary and mandatory data for understanding human odontology.
8 Conclusion
Materials Science offers a relevant approach for the studies of enamel and natural mineralized biological tissues. Technical improvements of the detection systems and recent increases in resolution allow fine descriptions of the biological phenomenon. These observations may evidence some pathophysiological mechanisms.
The emergence of tabletop systems (SEM with EDS detection, AFM, XRD, etc.) opens new perspectives for the understanding of tissue mineralization. Their availability and price are no more a limitation. Furthermore, the association between medicine and physical science offers opportunities for transdisciplinary investigations and exchanges.
Economic considerations should be also retained. These physical techniques also offer new methodologies for diagnosis. Comparisons between costs of these investigations and those of late diagnosis and surgery demonstrate the financial interest to use early fine physical techniques. For example, a diagnosis by Synchrotron radiation has a direct cost around 1.000 euro, but this investigation may avoid a graft or renal transplantation, which is estimated to be 50.000 euro without considering useless pain, discomfort and eventually morbidity.
Consequently, the interface of physics and medicine offers opportunities to increase basic, health and applied knowledge. This interface is still active and has retained a renewed attention during this last decade.
Acknowledgments
The authors thank the grant funding “Once Upon a Tooth” IDEX grant (AD – Grant number: ANR-11-IDEX-0005-02)