1 Introduction
Reduction of structural Fe in clay minerals by bacteria was first reported by Stucki, Wu, and co-workers [23,38,42,46]. Iron-reducing bacteria are ubiquitous in soils and sediments and are likely the dominant mediator of redox cycling of Fe in the environment, which plays a key role in the transformation of soil minerals and the fate of other soil constituents and contaminants. In recent years, increased attention has focused on the role of microorganisms in catalyzing Fe redox cycling in smectite clay minerals, including reduction of Fe(III) in the clay mineral structure and the dissolution and transformation of Fe (hydr)oxides. Studies involving abiotic Fe reduction, primarily using dithionite as the reducing agent, have shown strong links between clay surface chemistry and structural Fe oxidation state. Do bacteria assert a similarly large influence on the chemical and physical properties of soil clay minerals through bacteria-mediated Fe reduction in soil minerals? The purpose of this paper is to summarize some of the recent findings related to the effects of bacterial reduction on clay properties and behavior.
2 Iron reduction coupled with carbon oxidation
The stoichiometry for formate oxidation by Fe3+ in solution is given by:
They pointed out that these results are strong evidence that structural Fe(III) reduction in the clay is a product of bacterial respiration and that the bacteria directly couple carbon oxidation with Fe(III) respiration. Further investigation [24], revealing that the bacteria can grow when Fe(III) in the smectite is the sole electron acceptor, supports this hypothesis.
Still undetermined is whether this coupling occurs because of close membrane contact with the clay surface or through an electron shuttle. Some studies of bacterial cultures have reported an increased rate or extent of structural Fe reduction if an electron shuttle (e.g., AQDS or other humic substance) is added to the culture medium [8,9,25,30]. While the presence of a non-bacterial electron shuttle enhances bacterial reduction of smectite, its presence apparently is required in order to reduce structural Fe in illite [8].
3 Effects of bioreduction on clay properties and chemical behavior
3.1 Specific surface area
One of the most important properties imparted by clay minerals to soils and sediments is a large, chemically active surface area. Lear and Stucki [27] reported that dithionite reduction of structural Fe in smectite decreases its specific surface area, which led Kostka et al. [26] to investigate whether such a phenomenon occurs if reduction is mediated by bacteria. Their results (Fig. 1) illustrate that bioreduction decreases specific surface area of ferruginous smectite (SWa-1) by about 30% and Upton montmorillonite by almost 50%. Reoxidation restored most, but not all, of the surface area. This result was confirmed by Kim et al. [21], who, using environmental-cell transmission electron microscopy, observed a decrease in the interlayer spacing in bacteria-reduced nontronite.
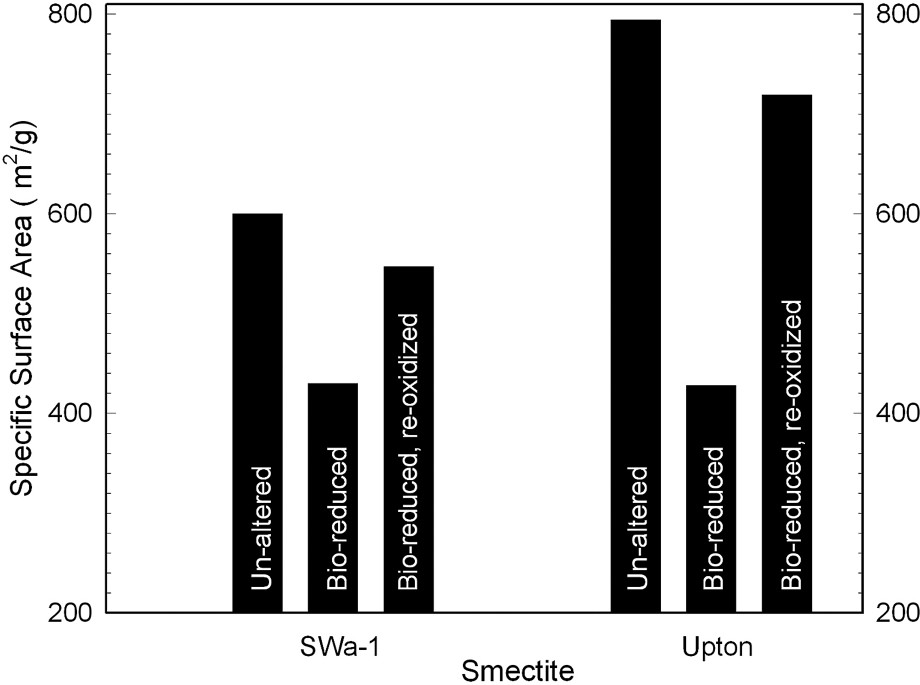
Effects of bacterial reduction of structural Fe on specific surface area of ferruginous smectite (SWa-1) and Upton, Wyoming, montmorillonite (data from [26]).
Effets d'une réduction bactérienne sur la surface spécifique d'une smectite ferrifère (SWa-1) et de la montmorillonite d'Upton (Wyoming, USA) [26].
3.2 Basal spacing and cation fixation
These observations are also consistent with the decrease in interlayer spacing found in dithionite-reduced smectites [47]. Such profound changes in surface area with redox cycling undoubtedly alter the chemical behavior of the minerals toward plant nutrients and environmental pollutants that enter the soil. Consequently, the chemical properties of soil minerals must be regarded as being dynamic rather than static, and fluctuation should be expected with variations in bacterial activity.
A decrease in specific surface area and the associated collapse of superimposed smectite layers clearly will also affect other important soil mineral properties. As the layers collapse, a fraction of the interlayer cations likely will become less exchangeable. This phenomenon has been confirmed for K+, Ca2+, Cu2+, Zn2+, and Na+ [20,27] in dithionite-reduced smectites. Shen and Stucki [32] further suggested this as a possible mechanism for in situ conversion of smectite to illite. Similar results are expected for bacteria-reduced smectite, and may be the explanation for the illite found by Kim et al. [22]. Cation fixation by bacteria-reduced smectites has yet, however, to be reported in the literature.
The decrease in interlayer spacing also increases the thickness of smectite particles, raising the number of layers per packet from 2–6 to more than 50 [40] in dithionite-reduced smectite. This transformation clearly impacts particle size and settling properties of the clay, as well as the permeability and hydraulic conductivity [33]. Again, similar effects are predicted in bacteria-reduced smectites, but have yet to be reported.
3.3 Swelling in water
Reduction of structural Fe oxidation state is well known to alter the swellability of smectites in water [11,15,16,41]. Gates et al. [17] were the first to report this effect for bacteria-reduced smectites with cultures of Pseudomonas (Figs. 2 and 3). Using a pure culture of the dissimilatory Fe(III)-reducing bacterium Shewanella oneidensis, Kostka et al. [26] similarly showed that bacterial-reduction is accompanied by a loss of clay swelling (Fig. 4).

Effects of bacterial reduction on the swelling of ferruginous smectite (SWa-1), as measured by the water content of the smectite in equilibrium with applied swelling pressures of 0.1, 0.3, and 0.5 MPa (data from [17]).
Effets d'une réduction bactérienne sur le gonflement d'une smectite ferrifère (SWa-1), mesurés par ses teneurs en eau à des pressions de 0,1, 0,3 et 0,5 MPa [17].
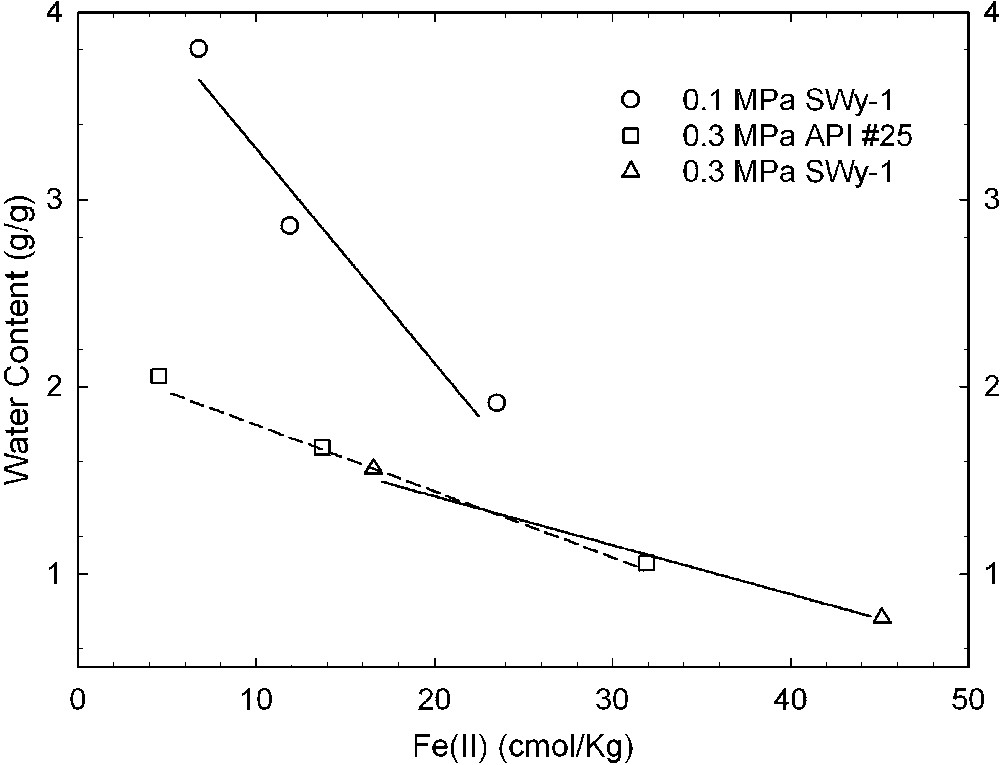
Effects of bacterial reduction on the swelling of two different samples of Wyoming montmorillonite, API #25 and SWy-1, as measured by the water content of the smectite in equilibrium with applied swelling pressures of 0.1, 0.3, and 0.5 MPa (data from [17]).
Effets d'une réduction bactérienne sur le gonflement de deux échantillons différents de montmorillonite du Wyoming, API #25 et SWy-1, mesurés par les teneurs en eau des smectites en équilibre avec des pressions de gonflement de 0,1, 0,3 et 0,5 MPa [17].
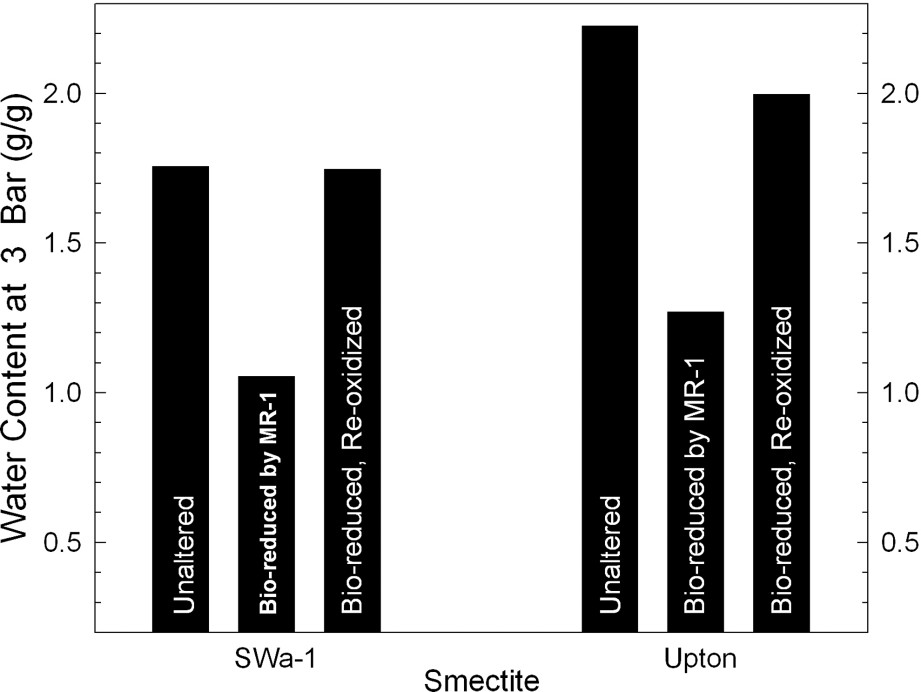
Effects of bacterial reduction of structural Fe on swelling pressure of ferruginous smectite (SWa-1) and Upton, Wyoming, montmorillonite (data from [26]).
Effets de la réduction du fer structural Fe sur la pression de gonflement de lac smectite (SWa-1) et de la montmorillonite d'Upton (Wyoming) [26].
These results are completely consistent with observations from dithionite reduction of the same smectites and follow naturally from the observed loss of specific surface area. Regardless of the source of structural Fe reduction (i.e., bacteria or dithionite), the conversion of structural Fe(III) to Fe(II) significantly perturbs the interlayer attractive forces of the smectite and promotes a more compact fabric of interparticle associations [22,41].
3.4 Layer charge and cation exchange capacity
The addition of electrons to the clay mineral structure to evoke reduction of structural Fe(III) to Fe(II) results in an increase in layer negative charge and cation exchange capacity (Fig. 5). In ferruginous smectite (SWa-1) reduced by S. oneidensis, the CEC increase is from 0.82 to 1.5 mequiv g−1, and in Upton montmorillonite the increase is from 0.85 to 0.99 mequiv g−1. The change in CEC varies directly, although not necessarily linearly, with the structural Fe(II) content. Ancillary reactions, such as structural dehydroxylation [31,36,37], cause the CEC vs. structural Fe(II) relationship to be more complicated than simply 1:1. Nevertheless, the increased electron content of the reduced smectite is reflected in the CEC. Upon re-oxidation, the CEC decreases, but not to the level of the unaltered clay. This discrepancy could be related to either incomplete re-oxidation or incomplete reversal of the structural changes occurring during reduction. Perhaps both of these factors apply. Further studies are necessary to gain a better understanding of the relationship between layer charge and adjustments in crystal structure due to stress created by Fe reduction.
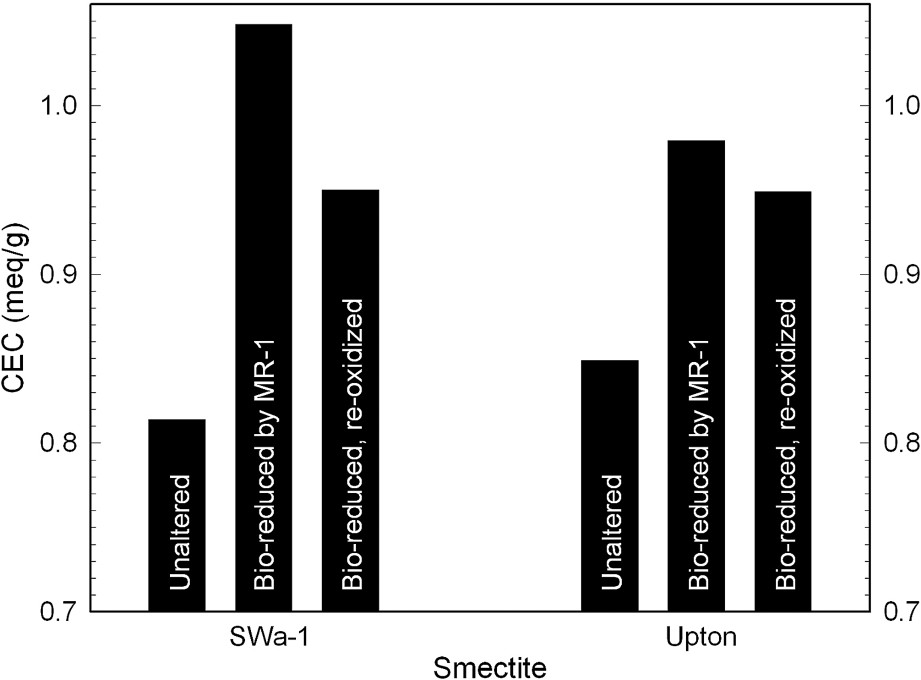
Effects of bacterial reduction of structural Fe on the cation exchange capacity of ferruginous smectite (SWa-1) and Upton, Wyoming, montmorillonite (data from [26]).
Effets d'une réduction bactérienne sur la capacité d'échange cationique d'une smectite ferrifère (SWa-1) et de la montmorillonite d'Upton (Wyoming) [26].
Favre et al. [12] linked the oxidation state of Fe in the smectite clays of rice paddy soils to an increase in the CEC, thus confirming that the Fe redox state affects the CEC in the field, as well as in the laboratory. The level of reduction observed in the rice paddy soils was comparable to that found in the laboratory with enrichment cultures.
3.5 Interlayer and interparticle forces
Changes in layer charge undoubtedly contribute to the interlayer attractive forces reflected in the enhanced cation fixation and decreased specific surface area described above, but other forces may also be operating. The traditional belief is that increased layer charge promotes greater attraction for interlayer cations and thereby contributes to interlayer attraction and collapse. This scenario could apply to reduced smectite, thereby promoting a more illitic or micaceous type of structure. Perhaps other forces (e.g., van der Waal's) also contribute to the tendency for reduced smectite to become more collapsed.
Forces that tend to resist the collapse of the interlayers are also present. Structural Fe reduction evidently increases the hydration energy of basal surfaces [43] and increases their Brønsted basicity [2,3], which results in enhanced hydrogen bonding at the surface. These factors work in opposition to the interlayer attractive forces, creating a resistance to layer collapse. The net effect of structural Fe(II) on clay swelling is, therefore, determined by the balance between these opposing forces, i.e., the greater attractive force between clay layers vs. the greater affinity of the basal surfaces for water. Exploration of these phenomena in bacteria-reduced smectites has only just begun.
3.6 Degradation of pesticides and other organic chemicals
During the past decade, studies demonstrated that abiotic reduction of structural Fe in smectite clay minerals greatly activates their surfaces with respect to degradation of environmental organic pollutants, including pesticides [44,48,50], 2,4,6-trinitrotoluene [18], nitroaromatics [19,49], carbon tetrachloride [1], and chlorinated and nitroaliphatic organic chemicals [2–7,45]. Studies also demonstrated changes in toxicity of pesticides to mammalian cells when they were reacted with reduced smectites [34,35]. In three of the foregoing studies, structural Fe reduction was accomplished by bacteria [6,7,37,48].
The effects of bacterial reduction on pesticide degradation are rather difficult to characterize because of the presence of organic matter (growth media, cell debris, metabolic exudates) in the resulting reduced clay suspensions. Xu et al. [48] surmounted this problem by comparing results from bacteria-reduced and bacteria-reduced-reoxidized smectites. They presumed that the organic material from the bacterial culture was the same or similar in both states of the system, and that the effect of re-oxidation by bubbling O2 through the suspension was primarily that of changing the oxidation state of structural Fe in the smectite. The amount of alachlor (Fig. 6) and atrazine (Fig. 7) that was either degraded or adsorbed by bacteria-reduced ferruginous smectite was about 30 and 100% greater, respectively, than with the reduced–re-oxidized smectite. This trend is consistent with observations from dithionite-reduced samples.
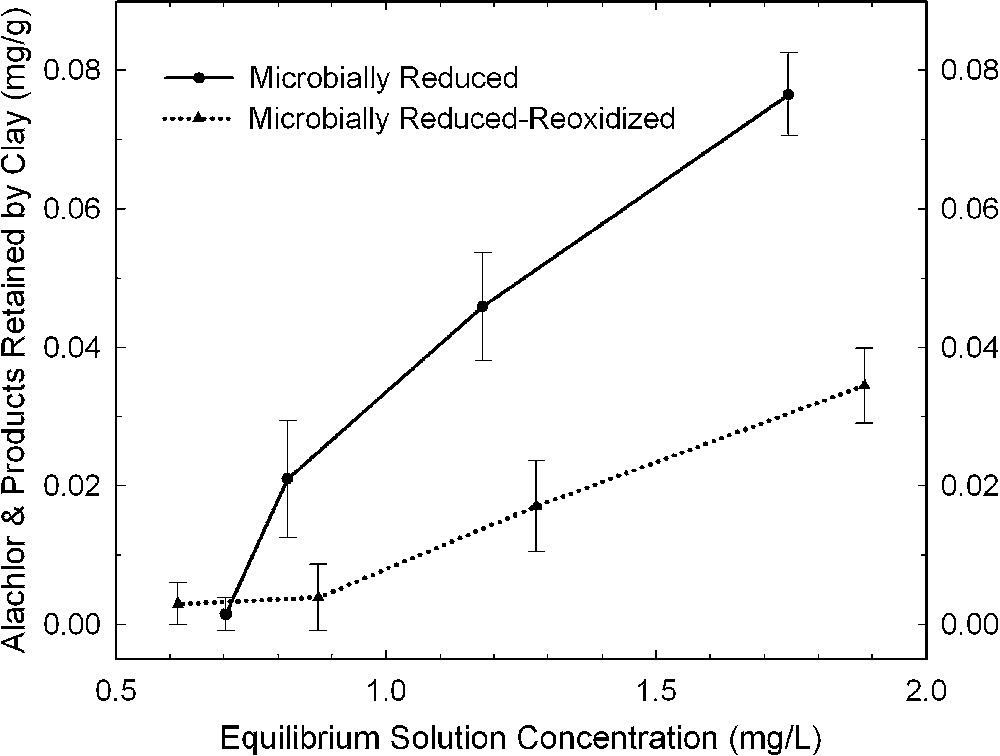
Amount of alachlor removed from solution by bacteria-reduced ferruginous smectite (SWa-1) before and after re-oxidation (data from [48]).
Quantités d'alachlor retirées de la solution par une smectite ferrifère (SWa-1), réduite par voie bactérienne avant et après ré-oxydation [48].

Amount of atrazine removed from solution by bacteria-reduced ferruginous smectite (SWa-1) before and after reoxidation (data from [48]).
Quantités d'atrazine retirées de la solution par une smectite ferrifère, réduite par voie bactérienne (SWa-1), avant et après ré-oxydation [48].
Chlorinated aliphatics are also altered by bacteria-reduced smectites, whereas the effect of oxidized smectite is much less. Cervini-Silva et al. [6,7], using a Fe-reducing bacterium (S. oneidensis strain MR-1) and a Fe(III)-reducing enrichment culture from a Chinese rice-paddy soil (designated PS), investigated the effects of bacterial reduction on the degradation of pentachloroethane (PCA) to tetrachloroethene (TCE) and of 1,1,1-trichloroethane (TCA) to dichloroethene (DCE). Results revealed that the rate of loss of PCA was greater in the presence of bacteria-reduced smectite than in the presence of the reduced–reoxidized smectite (Fig. 8). The formation of TCE, the hydrolysis product, increased commensurate with the loss of PCA (Fig. 8). TCA also degraded rapidly in the presence of bacteria-reduced smectite (Fig. 9), yielding increasing amounts of its hydrolysis product, DCE, as TCA degradation progressed. The effect was similar regardless of which bacteria were mediating the structural Fe reduction.
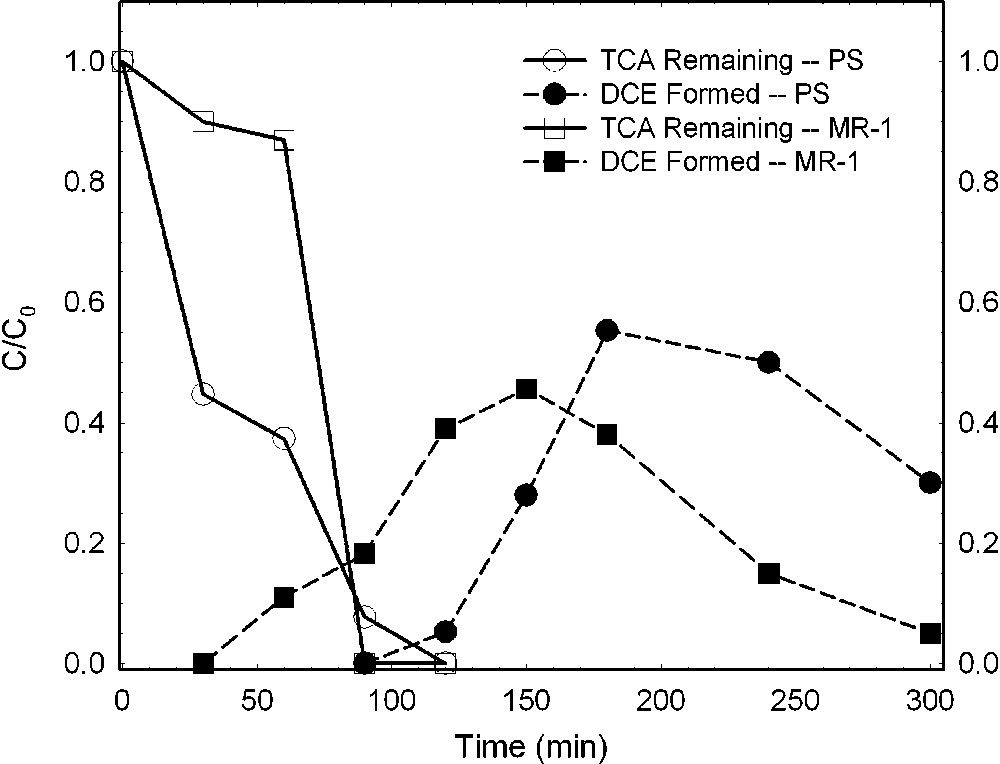
Dehydrochlorination of 1,1,1-trichloroethane (TCA) to dichloroethene (DCE) by ferruginous smectite reduced by Shewanella oneidensis (strain MR-1) or an enrichment culture from rice paddy soil (PS) (data from [6,7]).
Déshydrochlorination du 1,1,1-trichloréthane (TCA) en dichloréthylène (DCE) par une smectite ferrifère réduite, soit par des bactéries Shewanella oneidensis (souche MR-1), soit par une culture enrichie en provenance d'un sol de rizière (PS) [6,7].
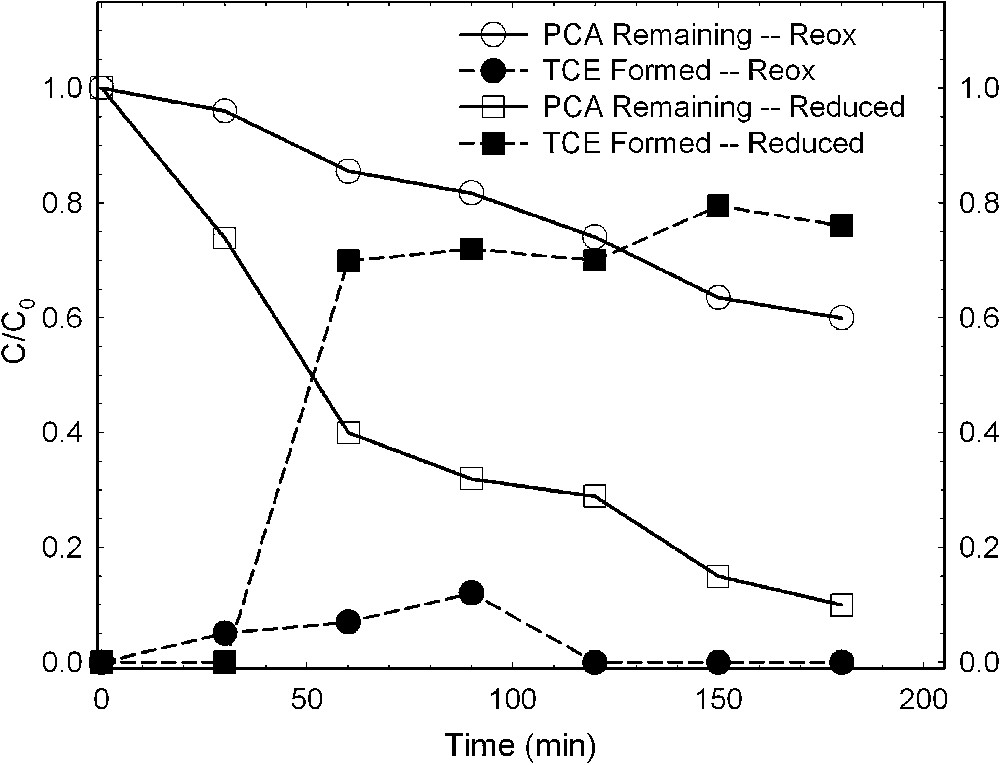
Dehydrochlorination of pentachloroethane (PCA) to tetrachloroethene (TCE) by ferruginous smectite (SWa-1) reduced by Shewanella oneidensis strain MR-1 (data from [7]).
Déshydrochlorination du pentachloroéthane (PCA) en tétrachloréthylène (TCE) par une smectite ferrifère (SWa-1) réduite par des bactéries Shewanella oneidensis (souche MR-1) [7].
3.7 Changes in clay structure and stability
Infusion of extra electrons into the clay crystal structure is a destabilizing event that creates a number of known ancillary reactions or processes, and likely causes some changes in the clay that have yet to be observed or characterized [36]. Known changes due to bacterial reduction are increased susceptibility to mineral dissolution; changes in short-range order within the clay crystal; and shifts in vibrational energies of structural OH, SiO, and M2OH [26]. Preliminary evidence also indicates a possible difference in the distribution of reduced Fe within the clay lattice compared to dithionite reduction [10].
A few recent studies have turned attention to the effect of bacterial reduction on clay dissolution. Dong et al. [8] observed vivianite (Fe(II) phosphate) in electron micrographs of nontronite NAu-1 reduced with S. oneidensis strain MR-1. The Mössbauer spectrum at 4 K of bacteria-reduced soil clay (data not shown) also reveals a magnetically ordered structure consistent with vivianite. These studies indicate, therefore, that at least some of the Fe is removed from the clay structure during bacterial reduction. The extent of clay dissolution and the fraction of total Fe dissolved are, however, still in question. Kostka et al. [26] previously found that the extent of dissolution depended on the presence of chelating agents in solution. In the presence of nitrilotriacetic acid (NTA), dissolution during bacterial reduction was extensive; but, in the absence of NTA, dissolution was minimal. Fig. 10 confirms that only small amounts of Fe are released to solution during bacterial reduction. This does not, however, preclude the possibility that other solid phases of Fe are forming outside the structure of the clay mineral lattice.

Effects of bacterial reduction on the dissolution of iron from smectites in the absence of chelating agents in solution.
Effets de la réduction bactérienne sur la solubilisation du fer contenu par différentes smectites, en l'absence d'agents chélatants en solution.
Lee et al. [28] undertook a study of changes in the vibrational energies of structural moieties in bacteria-reduced smectite. Dithionite reduction produces massive shifts in both the position and intensities of structural OH, SiO, and M2OH bands in smectites [13,14]. Bacterial reduction, on the other hand, yields only minor changes by comparison. Upon reduction of Garfield nontronite and Upton montmorillonite, the infrared OH stretching band shifted to slightly lower wavenumber in both of these clays and was largely restored to its original position upon re-oxidation. Similar minor changes were observed in the SiO stretching and M2OH bending bands, indicating that, even though some dissolution occurs, the changes to the clay structure are minor. Minor structural effects were also confirmed by polarized EXAFS [29].
4 Summary and conclusions
The studies described in this paper confirm that bacteria-mediated changes in the oxidation state of iron in soil clay minerals play an important role in determining the chemical and physical properties of soils and sediments. As Fe(III) is reduced to Fe(II) by bacteria, specific surface area decreases, cation exchange capacity (CEC) increases, swelling in water decreases, reactivity with organic chemicals and pesticides increases, and the potential for mineral dissolution and transformation increases. Changes in clay mineral structure due to bacterial reduction are, however, small. Structural Fe(III) can serve as the sole electron acceptor for respiratory bacteria, and Fe(III) respiration is stoichiometrically coupled with carbon oxidation when formate or lactate is the carbon source and electron donor.
Because of the large potential for the redox state of soil minerals to change with environmental conditions such as wetting, drying, warming, and cooling cycles, the chemical properties of the soil must be regarded as constantly changing. The resulting dynamic nature of soil behavior must be taken into account in management strategies to maximize soil fertility and structural performance. Studies of clay-bacteria interactions are continuing and further reviews will be forthcoming [39].
Acknowledgements
Financial support for research funding from the following is acknowledged: The International Arid Lands Consortium, Grant No. 03R-15; the National Science Foundation, Division of Petrology and Geochemistry, Grant No. EAR 01-26308; and the Natural and Accelerated Bioremediation Research (NABIR) Program, Biological and Environmental Research (BER), U.S. Department of Energy, Grant No. DE-FG02-00ER62986, including subcontract FSU F48792.