1 Introduction
The aridification of large areas since the Eocene is very often linked, at geologic time scales, to horizontal or vertical motions induced by tectonics. For instance, many papers have related the recent aridification of East Africa to the East-African Rift System (hereafter EARS) uplift (vertical motion) or to modifications of the Indonesian throughflow (horizontal tectonic drift) [4,24]. More interestingly, an increasing amount of evidence [12,13] and modelling studies [9,20,28] have recently pinpointed that the Chinese desert began to extend 22 million-years ago (Ma), mainly due to the Tibetan plateau uplift and to the shrinkage of the Paratethys. A striking feature in this case is that such a desert remains arid since 22 Ma, mostly because it is driven by tectonics, which is a long term forcing factor. This is completely different for the Sahara, whether the humid period rapidly collapsed [8], or ended more smoothly [16]. Indeed, this desert is closer to the ocean than its Chinese counterpart, and moisture advection within the western African monsoon system drives the climate with much higher frequency. Nevertheless, few data are available to record cyclic “green Sahara” episodes.
Schuster et al. [23] and Vignaud et al. [26] have shown that oscillations between lacustrine and desertic periods have existed in Chad since 7 Ma. Thus, we need to understand how climate evolved to set on such arid and humid conditions repeatedly. Also, the more recent mechanisms of climate changes during the Quaternary are still not very well-understood, and the scientific community, including paleontologists, archaelogists, and palynologists, need reliable numerical climate simulations to constrain the several scenarios of environmental evolution, as well as the main forcing factors. Last but not least, the earliest hominids known have been discovered in the Djurab desert [3], Chad, and constraining the environment-climate couple they evolved in is a decisive challenge for paleontologists.
During the Late Miocene, still active tectonics in the eastern part of the continent led to rapid changes in topography, eventually creating a physical barrier to moisture transported from the Indian Ocean. Details concerning the timing and the amplitude of the uplift can be found in [21,24]. At the timescale of millions of years, the onset of a topographic barrier such as the EARS can produce strong changes in atmospheric circulation. These changes are due to the physical obstacle produced by the mountain range which is oriented ∼90° from the zonal (westward and eastward) flows, but also to the strong thermodynamical differences between a low and an elevated area. Dynamical (i.e. atmospheric fluxes) and physical (rainfall patterns) changes linked to the uplift have been assessed for the eastern part of the continent [24]. However, in this study, the consequences of topographic changes on central and western Africa have not been analysed. To know if these events had remote climate consequences is far from being obvious, and the impact of such changes are assessed in the first part of this article.
In the second part, a first attempt is made to model with high resolution the impact of the Mega Lake Chad (MLC) on climate, with the background aim of understanding Chad basin hydrological processes at different timescales. Earlier, studies have focused on the Holocene MLC, as it is very well documented. Ghienne et al. [11] have proved the existence of this massive water surface between 11°N and 18°N by using digital elevation models to identify flat geomorphological structures that could only be produced by a giant lake. These results have been confirmed by other proxies (Schuster et al. [22] evidenced the presence of inselbergs in the southern Chad sub-basin) and satellite measurements [18,19]. Though the Holocene MLC water balance has been studied in earlier works [7,25], only one study has focused on the regional climate response to the presence of wetlands and lakes in northern Africa [6]. This study has suggested that surface water played an important role in forcing Holocene climate in northern Africa. With our new simulations, we aim at providing more detailed assessment of this impact, by increasing the spatial resolution compared to [6], and focusing on Chad basin hydrology.
Lastly, considering that climate over central Africa depends strongly on the West-African monsoon, which in turn is linked to the Sea Surface Temperatures (SSTs) of the Gulf of Guinea, we provide a study of the climatic consequences of changes in SSTs on this region.
2 Model and experimental design
In this article, our strategy is to use numerical modelling to assess climatic forcing factors one at a time (sensitivity experiments). The model we use is LMDz4 (Laboratoire de météorologie dynamique, Paris), an Atmospheric Global Circulation Model (AGCM). This AGCM solves the equations of the physics and dynamics of the atmosphere, forced by predefined physical conditions (boundary conditions) including monthly SSTs, greenhouse gas levels, icesheets extension, insolation, and topography [14]. By changing the values of these boundary conditions, one can assess their impact on climate atmospheric processes and ultimately on climate. LMDz4 is run with a stretched grid (i.e. zoomed), providing a ∼70 km resolution over a restrained region (here, the Chad basin). For each set of experiments, the model has been run between 15 and 20 years.
2.1 Impact of the East-African Rift System (EARS)
To assess the impact of the EARS uplift on atmospheric processes, we ran two numerical simulations with LMDz4. The first experiment is a control (CTL) run and was forced with every boundary conditions set at preindustrial values. As our goal here was to assess the atmospheric changes linked to the uplift we set up a second run (NORIFT) with a reduced topography over the eastern part of the African continent. These two experiments are identical to those found in [24], in which the zoom had already been set on the Chad basin.
2.2 Impact of the MLC
A lake model has been fully coupled to LMDz4 to account for feedbacks of a giant surface of water such as a MLC on atmospheric physics and dynamics. This lake model developed by Krinner [15] is made of 8 vertical levels. We can estimate the evolution of the lake volume by calculating the water balance between rainfall, evaporation and runoff over the basin, and deducing monthly the lake level by interpolating each point with a 1′ topography dataset. Where the lake is not present, the soil is represented by a bucket model, which means that when the water height exceeds 150 mm in a grid cell it is overflowed and considered as runoff. Runoff that occurs inside the hydrographic basin of Chad is added to the lake volume, in place of river inputs which are not simulated. Moreover, this model does not account for underground water reserves/inflows. To assess the impact of the MLC on climate, we used two experiments. The first one is the Control experiment depicted above, with no lake specified. The second experiment (LAKE0K) was run with the lake model initialized with a 350,000 km2 giant lake corresponding to the MLC [22]. Other boundary conditions are identical to CTL. In order to compare directly with results from Coe and Bonan [6], we present climatic outputs averaged over the basin, for the first 4 years of simulation.
2.3 Impact of the Guinean gulf SSTs
We ran a last experiment forced by different SSTs. As no reconstructed and gridded SSTs dataset is available for the Mid-Holocene, we used SSTs coming from a 6K experiment run with a coupled model for the Paleoclimate Modeling Intercomparison Project (PMIP) [2]. This strategy allows us to increase the resolution (our configuration of LMDz provides a high resolution whereas the coupled model's one is coarse) and makes comparison between forcing factors more meaningful, as a consistent tool is used for all experiments (Table 1).
Expériences LMDz4 et résumé bref des conditions aux limites utilisées dans cet article.
Experiment name | CTL | NORIFT | LAKE0K | SST6K |
Boundary conditions | AMIP SSTs, preindustrial greenhouse gases, modern tectonics | Identical to CTL, except reduced eastern African topography | Identical to CTL, but implemented with a lake model initialized with a Mega Lake Chad | Identical to CTL except for the monthly SSTs that are from an ocean model run at 6000 B.P. |
3 Results
3.1 Impact of the EARS uplift on the Saharan and sub-Saharan regions
Here we analyse climate outputs as an average of the whole run except the two first years, which are excluded for spin-up.
Moisture transports are significantly modified over the eastern part of the continent in both seasons, as depicted by specific humidity and winds at the surface in Fig. 1. North to the Equator the reduction of the topographic barrier in NORIFT leads moisture to be transported further from the Indian Ocean to the continent during austral winter. During austral summer, surface fluxes are inverted, and a clear northeastward moisture transport is simulated from southern Sudan to Somalia.
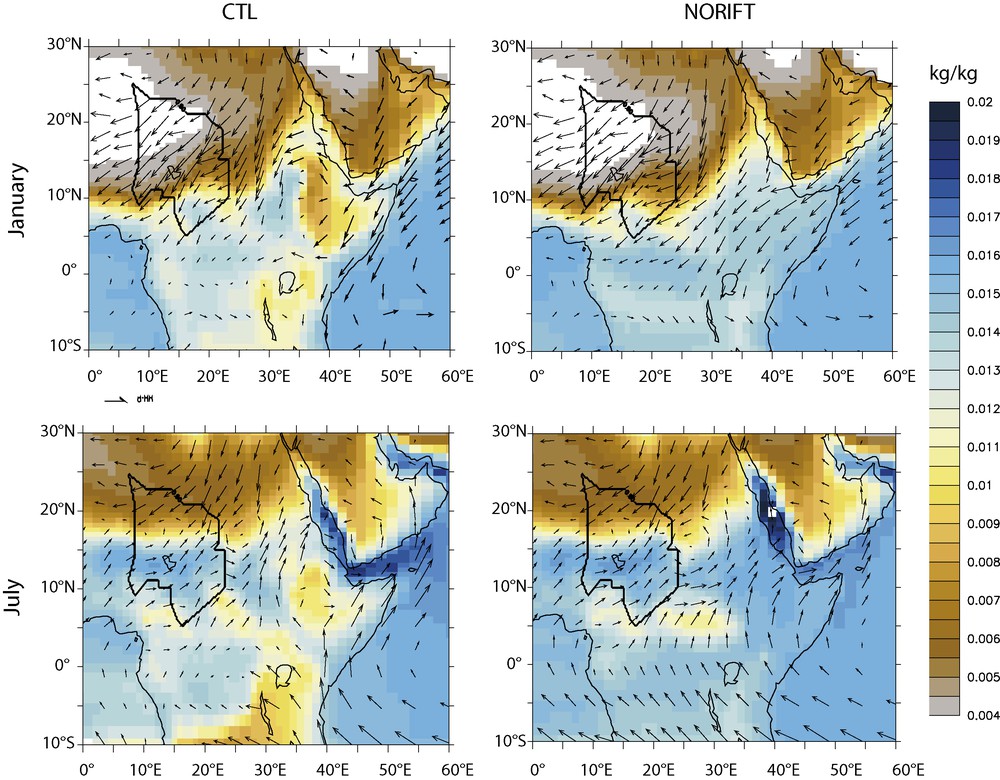
Simulated specific humidity and winds at 950 mb for CTL (left) and NORIFT (right), in January and in July. Polygon depicts Chad hydrologic basin limits.
Humidité spécifique et vents à 950 mb pour les simulations CTL (gauche) et NORIFT (droite), en janvier et juillet. Le polygone indique les contours du bassin hydrologique du Tchad.
No significant shift in moisture transport is simulated over the Sahara region, including the northern part of the Chad basin. January is characterized by the strong surface Thermal Low linked to the strong surface heating north to 15°N. Dry winds are oriented southwestward and are not influenced by the presence or absence of high topography over the eastern part of the continent. Over the southern part of the Chad basin, a slight change towards more westward surface winds is simulated in January when topography is lowered, inducing a weak increase of specific humidity. In July, the wind pattern is unchanged over this region.
Fig. 2 shows the rainfall changes between NORIFT and CTL. The increase in rainfall when topography is lowered (i.e. the aridification linked to the uplift of the EARS) is clearly simulated. Another interesting pattern in eastern Africa is the reduced rainfall amount in NORIFT over southern Sudan and Uganda during summer. This strong signal is clearly linked to water vapour transportation towards reduced-elevation areas of eastern Africa. Over the desertic areas of northern Chad and Saharan regions, no significant changes are observed.
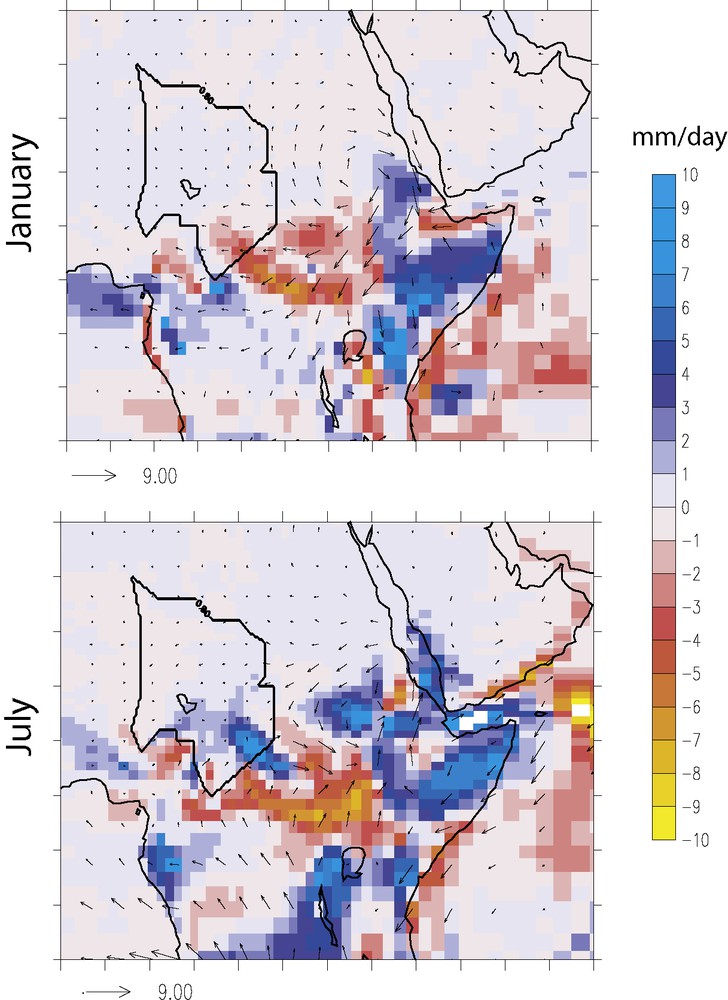
Rainfall anomaly (NORIFT-CTL) for January (top panel) and July (bottom panel), in mm/day.
Anomalie de pluie (NORIFT-CTL) en janvier (haut) et juillet (bas), en mm/jour.
3.2 Impact of a Mega Lake Chad on regional climate
The MLC covers 52 grid points in the LMDz surface scheme (Fig. 3). Although oversimplified, this representation allows estimating the climate response to the presence of this huge watershed. The presence of the MLC results in a cooling of the air at the surface over the lake by up to 5 °C (Fig. 4b and d), ultimately leading to a decrease of the sensible heat flux by 16% in JJA. Consistent with results from [6], the cooling induces a low-level increase of sea-level pressures over the lake. This feature occurs over the southern sub-basin in DJF and over the northern sub-basin in JJA, and drives changes in low-level winds. DJF southward low-level winds are reinforced and JJA northeastward monsoon flow is derivated westward. At the same time, annual summer latent heat flux is enhanced by 28% (Fig. 4g and h), with the MLC being a major source of evaporation. Evaporation (Fig. 5) over the megalake occurs all-year long, with highest values in the northern sub-basin in DJF (7 mm/day) and in the southern sub-basin in JJA (6 mm/day). However, water vapour coming from this source does not produce rain over the basin, as it is exported southward in winter and as convection is inhibited by the cold lake surface in summer.
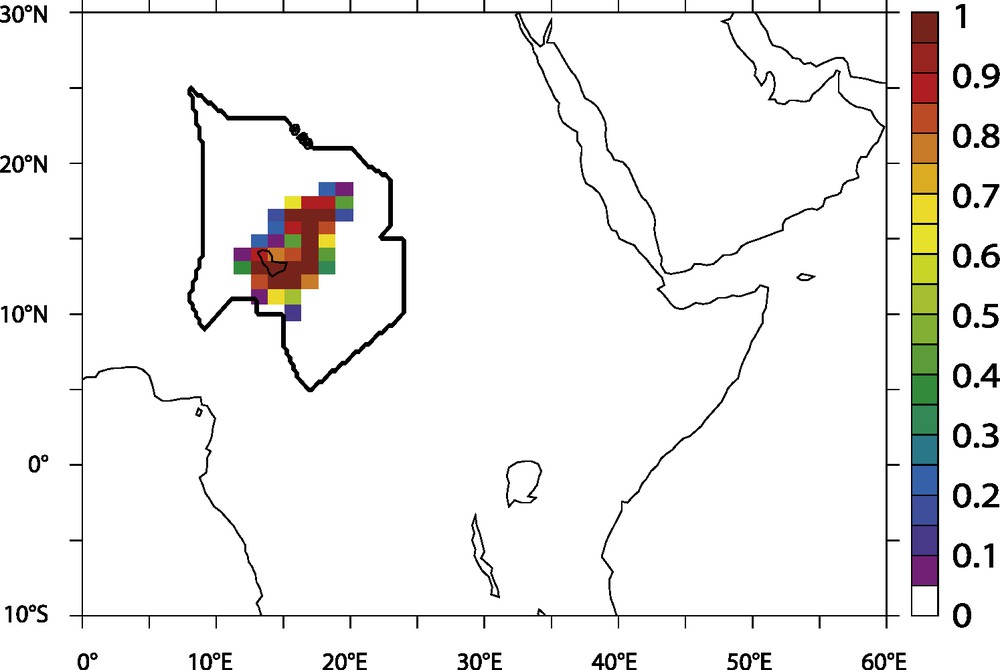
Fraction (varying between 0 for absence and 1 for full grid cell) of each grid cell occupied by lake in LAKE0K experiment.
Fraction (0 indique l’absence et 1 un point de grille plein) de chaque point de grille occupée par le lac dans la simulation LAKE0K.

Temperature and energetic changes when a giant lake is set over the Chad basin; a, c: air temperature at 2 m for CTL run, for DJF and JJA, respectively; b, d: LAKE0K-CTL anomaly for DJF and JJA, respectively; e: Annual sensible Heat flux anomaly between both runs; f: Seasonal cycle of sensible heat flux averaged over the basin, in W/m2; g, h, same as e, f, for latent heat flux (W/m2).
Variations de température et de balance énergétique quand un lac géant est prescrit dans le bassin du Tchad ; a,c : température de l’air à 2 m. pour la simulation CTL, pour DJF et JJA, respectivement ; b,d : différences LAKE0K-CTL pour DJF et JJA, respectivement ; e : différence annuelle de flux de chaleur sensible entre les deux simulations ; f : cycle saisonnier de chaleur sensible moyenné sur le bassin tchadien, en W/m2 ; g, h : identique à e, f, pour le flux de chaleur latente (W/m2).

Evaporation simulated for CTL (left panels) and LAKE0K (right panels). Top (bottom) panels are January (July). Units are mm/day. Vectors represent surface wind speed (m/s).
Évaporation telle que simulée dans les expériences CTL (gauche) et LAKE0K (droite). Les figures du haut (bas) indiquent les valeurs en janvier (juillet). Les valeurs sont exprimées en mm/jour. Les vecteurs représentent la vitesse du vent de surface (m/s).
Fig. 6 represents the Rainfall minus Evaporation (PE) balance averaged over the basin, for each month of the experiments. When no lake is included, the balance is obviously positive during the monsoon season and negative during the dry season. When a giant lake is included, the balance shifts to almost permanent negative values, reflecting the strong increase of evaporation due to the lake. By reducing the convection and stabilizing the lower layers of the troposphere, the lake prevents the water evaporated above its surface to be convected during the monsoon season, and recycled over the basin. The consequence is that in a modern-like climate context, a MLC would not produce local recycling of water, and ultimately vanish, if not fed by underground residual waters. An ongoing work aims at simulating the evolution and the impact of a MLC in a Mid-Holocene context, to check that its feedback in a more humid climatic background, with different surface conditions, is the same.
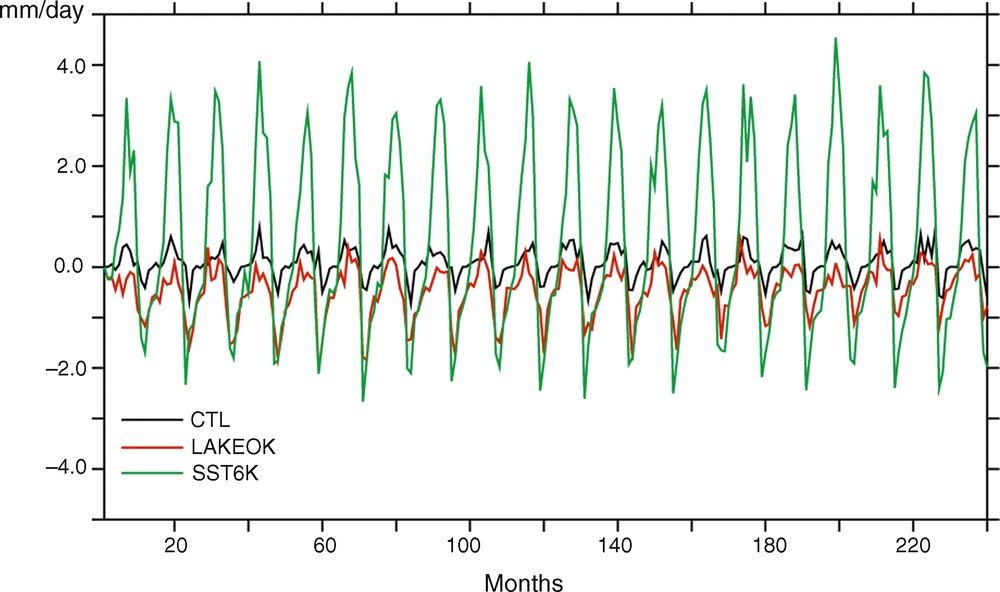
Precipitation minus Evaporation rate averaged over the basin for all the months of the three experiments. Units are mm/day.
Précipitation–Évaporation moyennée sur le basin pour tous les mois des trois simulations. Les valeurs sont exprimées en mm/jour.
3.3 Impact of sea surface temperatures of the eastern Atlantic
The thermal gradient between the tropical Atlantic and the adjacent continent is the “engine” of the West-African monsoon, and thus plays a very important role in rainfall patterns of sub-Saharan regions. Several modelling studies of the Mid-Holocene have focused on continental warming and suggested that 6K insolation, by enhancing the seasonal cycle at mid-latitudes and warming the continent during austral summer, favoured the northward shift of the monsoon. However, this parameter only could not induce a shift big enough to explain the data [1]. The second part of the engine is the ocean, which acts as a major water provider and where from the moisture advection towards the continent is initiated. In the late 1990s, Kutzbach and Liu [17] have suggested, through an asynchronous coupling between an AGCM and an ocean model, that SSTs warmer than present could enhance the African monsoon and produce wetter conditions over western and central Africa. However, recent works with a fully coupled model have demonstrated that warming event in the Guinean gulf tends to weaken the African monsoon [5]. This is explained by not considering the ocean as a water source only. Indeed, as the thermal contrast between the ocean and the continent drives moisture advection towards the continent, a slight decrease in SSTs over the Guinean gulf can enhance this gradient and then increase low-level moisture flow towards the continent and associated rainfall.
Our AGCM, forced by 6K SSTs coming from a PMIP coupled run [2] agrees with this hypothesis. Fig. 7a shows that the SSTs we use are colder than present. When forced by these SSTs, LMDz produces a very strong increase in rainfall all over western and central Africa, confirming that the monsoon dynamics are very sensitive to SSTs (Fig. 7b and c). This is also depicted in Fig. 6, in which the SST6K PE balance reaches +3 mm/day during the monsoon season. These results, which suggest a different mechanistic interpretation (“engine” instead of “source”) for the humid periods of the Sahara, will have to be tested via supplemental experiments, as present-day studies show complex relationships between SSTs and the West-African monsoon, involving an impact of northern tropical Atlantic [10,27]. Moreover, the fact that the monsoon is more sensitive to SSTs in our forced AGCM run than in the coupled PMIP run is still in question. The modelled SSTs from the PMIP experiment will also have to be compared to Mid-Holocene gridded dataset. Such comparisons should be achieved for other epochs with enhanced monsoon as well, in order to validate our hypotheses.

a: yearly averaged SSTs anomaly between CTL and SST6K experiments. Units are °C; b: Annual Rainfall as simulated in CTL experiment; c: SST6K-CTL anomaly. Units are mm/day.
a : différence de SST entre les simulations CTL et SST6K, moyennée annuellement. Les valeurs sont exprimées en degrés Celsius ; b : précipitation annuelle simulée par CTL : c : différence SST6K-CTL. Les valeurs sont exprimées en mm/jour.
4 Conclusion
This article has aimed at giving an assessment of major climate forcing factors that could potentially have influenced Saharan and sub-Saharan regions during the Late Cenozoic. Because evidences show the occurrence of a MLC several times during the last 7 Ma, one could speculate on the influence of the East-African Rift uplift role on moisture advection towards the Chad basin. Our results suggest that the Late Cenozoic uplift did not play a major role on climate evolution of central and western Africa, being too remote to induce any changes in moisture transport further than southern Sudan. However, its impact on eastern Africa is enormous. Another important point was to investigate whether a giant lake could be sustained just by acting as a major source of water and locally recycling evaporated water. Our preliminary experiments suggest that a huge watershed such as a MLC had a negative feedback on the water balance, by weakening convection with lower temperatures. This study will have to be followed by deeper analyses of moisture transportation in a humid climate context, maybe with water trackers included in the model, to assess if the water evaporated from the lake could be turned into precipitation on other parts of the basin, which is not the case in our runs (ongoing work). Also, further studies will have to account for runoff and ultimately to underground water sources.
Lastly, a simple experiment with SSTs colder than present suggests that they can “turn on” the monsoon engine and play a significant role in switching the central African climate from dry to wet. The change in the simulated hydrological cycle is so important that it could provide enough rain on the Saharan and sub-Saharan regions to maintain wetlands and lakes such as a MLC. Ongoing work will help testing this hypothesis and our results will have to be confronted to reconstructed and gridded SSTs records from the Guinean gulf.
Acknowledgments
We thank the ECLIPSE program from the CNRS. This work benefited from the great help from Yann Meurdesoif, at LSCE, and Gerhard Krinner (LGGE, Grenoble) to implement the lake model in LMDz code. Finally, we thank A.-M. Lezine for invitation to the Académie des sciences.