1 Introduction
Located at the western limit of the Upper Rhine graben, the Soultz-sous-Forêts area is a pilot zone selected by the European Hot Dry Rock (HDR) project whose purpose is to exploit geothermal low enthalpy energy (Gérard and Kappelmeyer, 1987; Sanjuan et al., 2006). Thermal anomalies are linked to the specific geotectonic context of the Rhine graben, an intracontinental rift with abnormal thermal gradients, and localized fluid flow in relation with faults.
The observation of brines in basement rocks have been the subject of several controversies in the past, for example, those concerning the Canadian shield brines (Frape and Fritz, 1987) or those found in the KTB deep drilling (Möller et al., 2005), especially when the sedimentary cover is now lacking above the basement. Thus, the significant chloride content of fluids, more or less equilibrated with their host-rocks, is either considered as the result of the penetration of sedimentary brines (Munz et al., 1995), or from water-rock interactions and Cl release during alteration (Edmunds et al., 1984). The basement below the Mesozoic and Cenozoic series from the Rhine graben offers the opportunity to study past to present day fluid movements within and between different kinds of aquifers (sedimentary formations, crystalline basement), and especially the deep downwards penetration of brines, issued from the overlaying sedimentary formations, into the basement.
At Soultz-sous-Forêts, past to recent fluid movements are recorded by series of healed or sealed micro- to macrostructures, which provide evidence of a rather continuous self-sealing of the open space. Previous studies of paleofluid circulation in Exploration Puits Soultz (EPS-1) drill hole have shown the circulation of moderate to highly saline fluids either in the sedimentary cover or in the basement down to 2200 m depth (Dubois et al., 2000). The Géothermie puits Kutzenhausen (GPK2) deep drilling has provided a few samples of the basement at 5057 m which were considered as particularly useful for the investigation of paleofluid events and their relation to present day reservoir at this depth. A detailed characterization of the paleofluids trapped in fluid inclusion planes (FIP) in the granite has been carried out in order to determine their composition and their trapping temperature. Fluid inclusion temperature estimates were compared with present day measured temperatures in the borehole.
2 Geological setting
The Soultz-sous-Forêts geothermal plant is located in the Rhine Graben which constitutes part of the continental rift extending from Frankfurt (Germany) to Basel (Switzerland). It formed during the Oligocene by large-scale extension related to mantle upwelling (Rousset et al., 1993). The north-western part of the Rhine graben is filled by an asymmetric sedimentary cover ranging from Triassic to Oligocene overlying a Hercynian fractured granitic basement.
Abundant literature is available on the geology and hydrogeology of the basement at Soultz-sous-Forêts area, in relation with the drilling of EPS1, and GPK1, 2, 3 holes, and is briefly summarized as follows:
- • the main granite body is an Hercynian monzogranite dated at 331 ± 9 Ma (U-Pb method, [Alexandrov et al., 2001]) with no equivalent at the surface. Its mineralogical composition comprises phenocrysts of pink alkali feldspars (1 to 5 cm in size) in a matrix of quartz, plagioclase, biotite and minor amphibole. Magnetite is abundant and sphene, apatite, allanite and zircon are frequent (Stussi et al., 2002). At depth, a two mica granite and a biotite – amphibole rich porphyry granite were found (Genter et al., 1999; Hooijkaas et al., 2006);
- • in drill holes, unaltered granite alternates with largely altered zones characterized by dense network of veins, and the alteration of the host rocks (Genter and Traineau, 1996; Ledésert et al., 1999; Sausse, 2002; Sausse et al., 2006; Traineau et al., 1991). Previous studies have documented large-scale modern fluid circulation in the sedimentary basement and within the fractured granitic bedrock. This circulation is believed to be at the origin of drastic changes in heat transfer in this area, explaining that the present-day temperature gradient decreases from 0.09–0.10 °C m−1 in the sedimentary cover to unexpected values of 0.04–0.01 °C m−1 in the upper part of the fractured granites (Clauser and Villinger, 1990; Person and Garven, 1992).
3 Petrography of the studied granite
Four samples of granite from the GPK2 drill hole (drilling depth: 5057 m corresponding to a true vertical depth of around 4858 m) have been studied: (i) the sample noted K1-1, 5057.05 m is a biotite–amphibole rich porphyric granite, with a rather high biotite content, partially chloritized, and which could correspond to a mafic-rich mineral cumulate and/or Schlieren; (ii) the sample K1-3, 5057.1 m which is a dark fined-grained xenolith, with planar orientation of mafic minerals, corresponds to the frequently observed xenolith in EPS1 cores (Genter et al., 1999), and (iii) the two other samples are composed of a two mica granite (K1-13, 5057.36 m and K1-40, 5057.98 m) which correspond to the main granite body found at depth. These granite samples display the same mineralogical features than those described by Genter et al. (Genter et al., 1999) and Hooijkaas et al. (Hooijkaas et al., 2006). Alteration consists in the partial to complete replacement of biotite by chlorite, and a discrete crystallization of phengite or illite in plagioclase, but is ambiguously attributable to inherited Hercynian retrograde alteration or to recent fluid rock interactions. The lack of veinlets and significant alteration indicates that samples were taken away from major sealed drains such as those described by Smith et al. (Smith et al., 1998).
3.1 Fluid inclusion characterization
Fluid inclusions (FI) were studied on wafers of about 150 μm thickness. Microthermometric measurements were carried out on a Linkam heating - freezing stage previously calibrated using synthetic fluid inclusions. The accuracy of measurements is considered to be ± 0.1 °C at low temperature and about ± 0.5 °C at the maximum temperatures achieved in this study (250 °C). The fluid inclusions observed in quartz grain from the granite are two-phase aqueous inclusions. Thus two measurements were systematically made in each inclusion: i) the melting temperature of ice (Tm ice) which can be converted to equivalent NaCl concentration using the equation of Bodnar (Bodnar, 1993); and ii) the bulk homogenization temperature (Th) which represents the minimum trapping temperature.
Quartz grains from the granite host early fluid inclusions along grain boundaries with heterogeneous features which can be attributed to the early history of the granite (not considered in this study), and sets of fluid inclusion planes (FIP). Traces of paleomicrofractures are only made visible by the fluid inclusion plane, the microfracture being just healed and not sealed. Most of FIPs display homogeneous features (L/V ratio), and small sizes (5–10 μm). Microthermometric measurements indicate that fluid inclusions from a single FIP represent a single fluid event characterized by homogeneous features (density, salinity) according to what is expected for the healing of quartz in presence of a homogeneous fluid (Cathelineau et al., 1994). The microthermometric data of the FIPs are presented in Table 1 and Fig. 1.
Résumé des données microthermométriques obtenues sur les inclusions fluides des quatre échantillons prélevés à 5057 m dans le puits GPK2.
Sample | Depth (m) | Mineral | Tm ice (°C) | n | Th (°C) | n |
K1-1 | 5057.05 | Quartz A | −1/−2 | 8 | 145.5/158.1 | 8 |
Quartz B | −17.9/−24.6 | 6 | 150.2/173.3 | 5 | ||
Quartz C | −13.5/−16.1 | 7 | 207.2/237.2 | 5 | ||
K1-3 | 5057.10 | Quartz A | −1.2/−4.5 | 14 | 168.4/184.2 | 13 |
Quartz D | −0.1/−3.5 | 21 | 177.0/184.9 | 20 | ||
K1 -13 | 5057.36 | Quartz C | −2.8/−4.6 | 13 | 171.9/189.1 | 11 |
Quartz A | −1.9/−17.8 | 23 | 161.4/189.4 | 22 | ||
K1-40 | 5097.98 | Quartz A | −4.8/−9.3 | 13 | 169.4/177.3 | 10 |
Quartz B | −2.4/−3.1 | 11 | 171.6/182.0 | 11 | ||
Quartz C | −1.0/−15.3 | 22 | 167.0/190.0 | 16 |
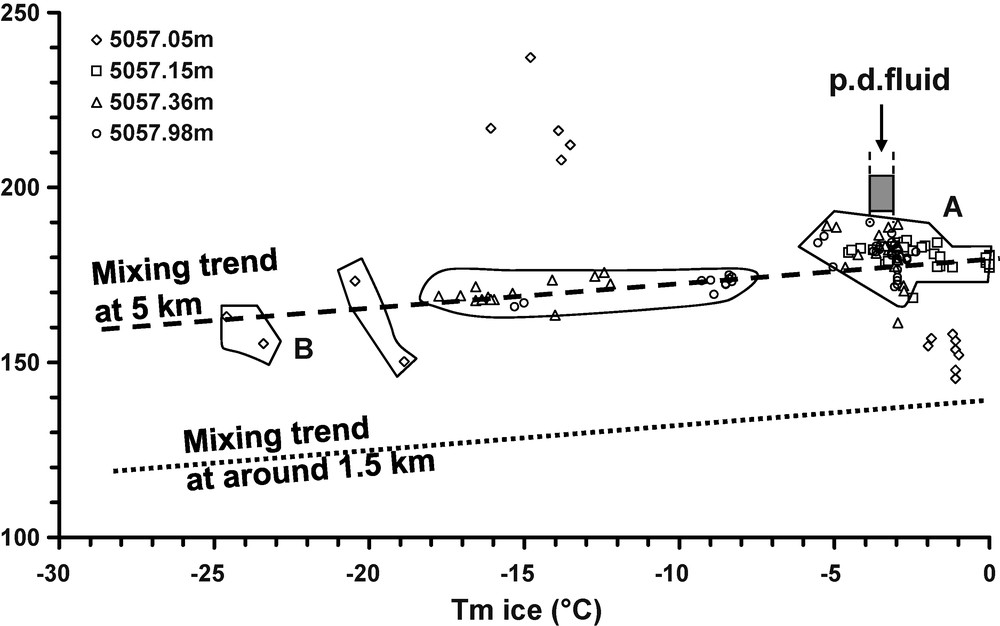
Tm ice–Th diagram applied to the four studied samples at 5057 m from the GPK2 borehole, with indication of the present-day fluid features (temperature and salinity from Sanjuan et al. (Sanjuan et al., 2006)). A mixing trend at 1.5 km is mentioned according to the data from Dubois et al. (Dubois et al., 1996; Dubois et al., 2000). p.d. fluid: present day fluid.
Diagramme Tfg (fusion de la glace)–Th appliqué aux quatre échantillons étudiés du puits GPK2 à 5057 m, avec indication des caractéristiques des fluides actuels (température et salinité d’après Sanjuan et al. (Sanjuan et al., 2006)). La tendance de mélange à 1,5 km de profondeur est reportée d’après les données de Dubois et al. (Dubois et al., 1996; Dubois et al., 2000). p.d. fluid : fluide actuel.
The fluid inclusion set is characterized by a large range of Tm ice from −24.6 to near −0.1 °C (Table 1), indicating a potential mixing of two end members: a brine and a rather diluted fluid. When observed, first melting occurs between −40 °C and −65 °C (± 5 °C), which indicates the presence of divalent cations, Ca2+ and Mg2+ in addition to Na+, as confirmed by semiquantitative investigation using LIBS.
All inclusions homogenized to the liquid phase between 145 °C and 237 °C, but most of the measured Th at the exception of two sets of FIPs, range between 150 °C and 185 °C. The Tm ice–Th diagram (Fig. 1) shows that the fluid inclusions with the highest salinity (i.e., the lowest Tm ice) have the lowest homogenization temperatures (around 150 °C), and the low salinities correspond to the highest Th around 180–190 °C. The overall distribution of the data is typical of a mixing trend between two main fluids: a brine with slightly lower Th and a hotter dilute fluid.
Only one sample (5057.05 m) displays three types of FIPs with contrasting features: one similar to those already described for the other samples, but also two FIP sets exhibiting distinct features: i) one is characterized by Tm ice values around −1 °C but lower Th than in the other samples, i.e., around 150 °C; and ii) another FIP set characterized by a higher Th around 210 °C.
At variance with observation of granites from EPS-1 (Dubois et al., 2000), no evidence of late retrograde fluids was found in the FIPs, such as CO2-rich fluids or high temperature hydrothermal fluids related to fluid events prior to the main events affecting the basement since the Oligocene. In addition, the fluid inclusion studied display a distribution of Tm–Th pairs rather similar to that found in the euhedral quartz crystals from the shallowest levels of the granite. Thus, the mixing trend found at 5057 m is subparallel to the mixing trend found for samples from a depth ranging from 1430 to 2207 m (Dubois et al., 2000). The two extreme salinities are similar in both cases, but a difference in Th of approximately 35–40 °C is found between the two sets of samples.
4 Discussion
Fluids found as inclusions in healed microfissures from the deepest samples at Soultz display the same features as those observed in recent quartz veins from the shallow levels of the granite. They attest of discrete fluid sampling resulting from mixing of two main fluids: i) a basinal brine, probably issued from the Trias formations, and characterized by a high salinity of 20 wt. % eq.NaCl; ii) a low salinity fluid which could correspond to a recharge water (deep meteoric fluid penetration from the Vosges mountains). The variety of salinity–temperature pairs indicates that at each stage of healing of the microfissures, a distinct rate of mixing between the two fluids is registered. These data show that the granite reservoir fluids were not fully homogenized at the time of healing of the microfissures. The predominant fluid inclusions have a salinity of 49 to 64 g/l, thus very similar to those recorded during the water sampling (around 55 g/l, bottom hole production) in GPK2 (Sanjuan et al., 2006), but the whole set of data (1 to 200 g/l) brackets the present day salinity.
Temperature recorded at depth by fluid inclusion planes is around 185–210 °C (Th around 185 °C plus a pressure correction of 25 °C at 5 km assuming an hydrostatic pressure). This temperature is remarkably similar to those recorded at present before the reinjection (around 200 °C, Pribnow et al., 1999) (Fig. 2). The presence of lower temperature brines indicates that downward penetration of sedimentary brines tends to cool the deep reservoir fluids. The 35–40 °C gap between the estimated fluid inclusion temperature recorded in between 1500 m and 2000 m and those recorded at 5000 m correspond to a mean thermal gradient of about 10–11 °C /km, a rather low value but coherent with the temperature–depth profile measured in the GPK drill holes. The 25 °C temperature difference between the brine and the hotter dilute fluid is found both at 2000 and 5000 m depth, indicating an anisothermal mixing in all parts of the granite reservoir.

Temperature depth profile (present day measured temperatures from Pribnow et al. (Pribnow et al., 1999) and fluid inclusion estimates (corrected for fluid pressure). Fluid inclusion data EPS1 from Dubois et al. (Dubois et al., 2000), fluid inclusion data GPK2: this study.
Profil thermique (températures actuelles mesurées d’après Pribnow et al. (Pribnow et al., 1999) et données des inclusions fluides (Th corrigées des effets de la pression). Données inclusions fluides d’après Dubois et al. (Dubois et al., 2000) : pour EPS1, et à partir de cette étude pour GPK2.
Fluid inclusion planes in deep samples contain fluids having striking similarities with those found as primary inclusions in euhedral quartz from veins at shallower levels. In the shallow levels, FIP are predominantly inherited from hotter fluid events, possibly from Hercynian stage. FIP from deep samples are dominated by recent reservoir fluids and FI salinities depict the full range of mixing between the sedimentary brine and the hot dilute fluids. Thus, either sets of microfissures were formed and healed at depth since the Oligocene or the inherited FIP from earlier stages were reopened and healed by the main reservoir fluids. The microfissuring and healing conditions at 5 km depth seem more favorable than at 1–2 km depth.
The pattern of palaeofluid circulations at Soultz two-fold with (Fig. 3):
- • a major system of laterally migrating brines at the granite–sedimentary cover interface where both sandstones and the upper part of the (granitic) basement had a high permeability (a property acquired for the basement by weathering during the emersion period);
- • local convective cells (rooted at least at more than 5 km depth) involving low salinity fluids (presumably surface derived, likely coming through the Vosges basement) and centered on horst fault systems;
- • deep downward penetration of brines which accounts for the introduction of large amounts of chlorine in the granitoid micropores and for the high chlorine concentrations of the present day fluids recovered in the granitoid aquifer.

Model of fluid circulation in the basement of the Rhine graben showing deep penetration of sedimentary brines down to 5 km depth and fluid mixing with recharge meteoric water.
Modèle de circulation des fluides dans le socle du graben du Rhin montrant la pénétration profonde des saumures d’origine sédimentaire jusqu’à 5 km en profondeur, et leur mélange avec des fluides de recharge météoriques.
The lack of quartz vein or veinlet in the deep samples from GPK2 did not allow the study of the degree of isotopic equilibration between fluids and host-rocks such as that done on EPS1 vein by Smith et al. (Smith et al., 1998). The latter authors concluded that entering fluids did not undergo the same degree of interaction with the granite, e.g., a further indication of contrasted speed of fluid migration and fluid inhomogeneity.
The healing of microfracture sets in the basement all along the Rhine rift hydrothermal activity is important to document as it has successively reduced the microfracture permeability. These processes are of particular interest to take into consideration during the monitoring of the fracture networks in the hot dry rock projects (as for instance the injection tests in the Soultz granite for an enhanced microfracture permeability). In addition, the determination of the origin of chloride in ground-waters as well as the evolution of its concentration are very important parameters for the modeling of the fluid hydrogeochemistry in fractured basement aquifers. In the particular case of Soultz, the relatively homogeneous chlorine content of the produced present day fluids is the result of successive mixings of fluids coming from parts of a reservoir not chemically homogenized.
Acknowledgements
This work has been supported by the ECODEV program. The authors thank A. Gérard to have stimulated and encouraged fluid inclusions studies, J.-J. Royer (ENSG- CRPG, Nancy-université) for providing the four samples and J. Sausse (G2R, Nancy Université) for her help and her knowledge of the Soultz-sous-Forêts geothermal exchanger. Two anonymous reviewers are warmly acknowledged for their constructive remarks.