1 Introduction
After a period of geoscientific quiescence in the last third of the 20th century (Villeneuve et al., 2015), the Adrar Souttouf Massif in the southern Moroccan Sahara became again a subject for geological studies. Most of them focussed on geochronology, but were almost exclusively limited to igneous rocks and their metamorphic equivalents (Bea et al., 2016, 2017; Gärtner et al., 2013a, 2016a, 2016b; Montero et al., 2014, 2016; Villeneuve et al., 2006). Data obtained from these studies clearly show differences in the magmatic (Bea et al., 2016, 2017; Gärtner et al., 2013a, 2016a; Montero et al., 2014, 2016) and crustal evolution (Gärtner et al., 2016a, 2016b) of the Adrar Souttouf Massif's four main units, as introduced by Villeneuve et al. (2006). Of them, the Oued Togba and Sebkha Gezmayet units form the western parts of the massif. Both of the latter contain some igneous rocks of Early Devonian age (Gärtner et al., 2013a), which partially show signs of almost coeval metamorphic overprint (Bea et al., 2017). Additionally, the igneous and ortho-metamorphic rocks of the Oued Togba unit contain a remarkable inheritance of Mesoproterozoic zircon grains. Neither Devonian nor Mesoproterozoic magmatism is known from the West African Craton (Ennih and Liégeois, 2008; Gärtner et al., 2017; Linnemann et al., 2011). However, the Avalonian and Meguma terranes that were accreted to Laurussia during the Mid-Palaeozoic comprise many Devonian igneous complexes (Pollock et al., 2012; van Staal et al., 2009). Furthermore, most of the Avalonian terranes have a prominent sub-peak of Mesoproterozoic zircon ages. Hence, the westernmost Oued Togba unit was thought to be of Avalonian affinity, while the adjacent Sebkha Gezmayet unit to the east could be somehow linked to the Meguma terrane (Gärtner et al., 2013a, 2016b). Despite the very limited access to the working area and very limited outcrops, this study aims to prove the latter by analysing the detrital zircon record of the sedimentary rocks of the Sebkha Gezmayet unit.
2 Geological setting
The Adrar Souttouf Massif comprises four SSW–NNE-trending tectonic units that form multiple thrust sheets, which were partially thrusted above each other with an eastward vergence during Ediacaran, but finally during Carboniferous contractional deformation events (e.g., Gärtner et al., 2013a, 2016a; Villeneuve et al., 2015; Fig. 1). These tectonic units are termed from west to east (Villeneuve et al., 2006, 2015): Oued Togba, Sebkha Gezmayet, Dayet Lawda, and Sebkha Matallah units (Fig. 1). A thin skin model is assumed for the entire massif (e.g., Michard et al., 2008, 2010; Sougy, 1962; Villeneuve et al., 2006) that is corroborated by geophysical data (Fateh, 2008; Labails et al., 2010), as well as potential basement windows of igneous rocks of the western Reguibat Shield in the Oued Togba and Sebkha Matallah units (Gärtner, 2017; Gärtner et al., 2013a).
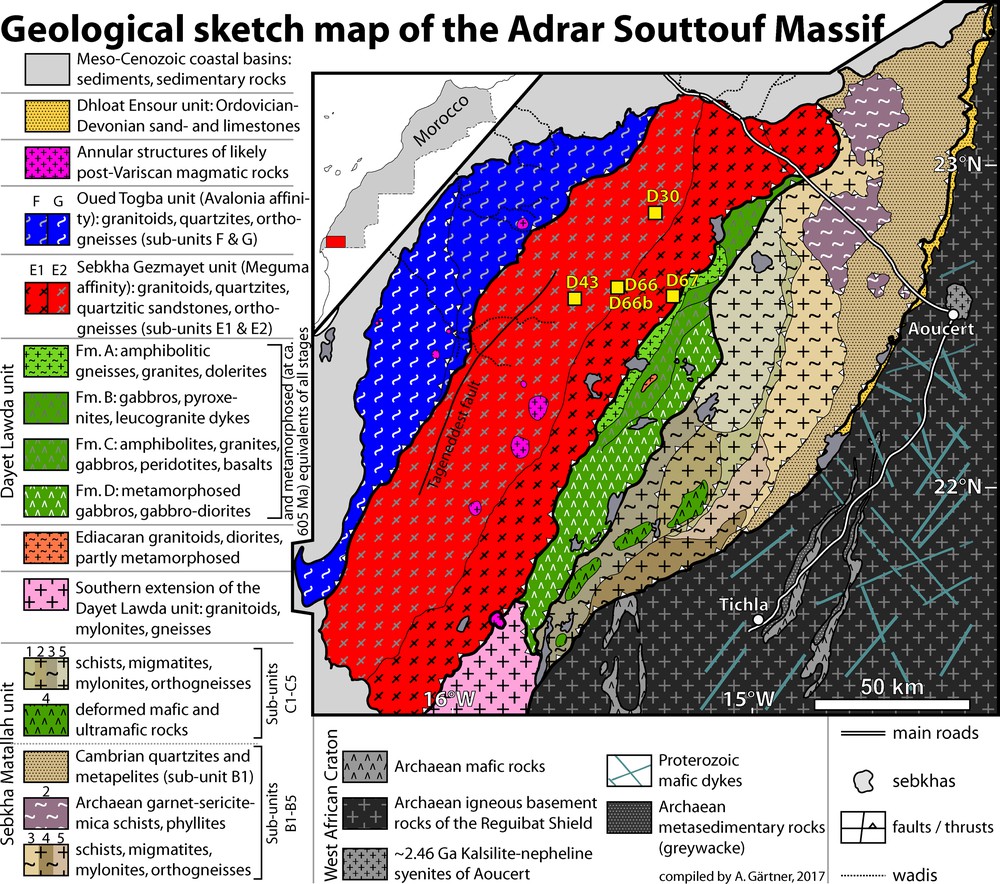
Geological sketch map of the Adrar Souttouf Massif and sample localities (compiled from Lécorché et al., 1989; Rjimati and Zemmouri, 2011; Rjimati et al., 2002a, 2002b, 2002c, 2002d, 2002e, 2002f, 2010, 2011; Villeneuve et al., 2006, 2015; and own studies).
The eastern margin of the Adrar Souttouf Massif is thrusted over a thin succession of Latest Ordovician to Devonian siliciclastic rocks and shallow marine limestone, termed the Dhloat Ensour unit (Gärtner et al., 2017; Villeneuve et al., 2006). A diamictite at the base of the succession likely results from the Hirnantian glaciation (Destombes et al., 1969; Lécorché et al., 1991; Michard et al., 2010; Rjimati et al., 2002a; Sougy, 1969), whereas the biocenosis in the uppermost limestones point to a Lochkovian age (Sougy, 1962). This links the thrusting of the Adrar Souttouf Massif over the Dhloat Ensour unit to the Variscan Orogeny (e.g., Bronner et al., 1983; Rjimati et al., 2002a; Sougy, 1962; Sougy and Bronner, 1969).
Recent studies on zircon age populations of igneous and metamorphic rocks revealed an Avalonian affinity of the Oued Togba unit, while the Sebkha Gezmayet unit resembles the known zircon age spectra of Meguma (Gärtner et al., 2013a, 2016b). Both units are mainly made of felsic igneous rocks of almost exclusively Neoproterozoic to Permian age and their metamorphic equivalents (Bea et al., 2017; Gärtner et al., 2013a; Montero et al., 2016, 2017), but also contain siliciclastic (meta-) sedimentary rocks of yet unknown age (see below). The Dayet Lawda unit in the central part of the massif comprises mafic igneous rocks in all stages of metamorphic overprint up to granulite facies (Bea et al., 2017; Gärtner et al., 2016a). In some parts, this unit hosts occurrences of non- to weakly deformed granitoid rocks, diorite and felsic tuff, while any kind of sedimentary rock has not yet been found (Gärtner et al. 2016a; Villeneuve et al., 2015). The Dayet Lawda unit is interpreted as an oceanic island arc that was active at ca. 635 Ma, and which underwent high-grade metamorphic conditions during a collisional event at about 605 Ma (Gärtner et al., 2016a). As a result, parts of this unit were obducted onto the proto-Sebkha Matallah unit to the east that rests unconformably on the West African Cratons’ igneous basement.
The Sebkha Matallah unit forms the easternmost part of the Adrar Souttouf Massif and comprises a variety of metamorphic rocks (e.g., amphibolite, migmatite, phyllite) cut by mafic dykes. Felsic igneous rocks occur scattered in this unit (e.g., Bea et al., 2016). The northern and eastern parts of this unit are covered by Neoproterozoic to Earliest Cambrian, weakly deformed siliciclastic rocks (e.g., Rjimati et al., 2002a, 2002b). Zircon data from the Sebkha Matallah unit suggest a strong relation to the adjacent Reguibat Shield (Bea et al., 2013, 2016; Gärtner et al., 2016a, 2017; Montero et al., 2014, 2016). However, most of these data were obtained from magmatic or metamorphic rocks, which allow only limited palaeogeographic reconstructions due to often scarce inheritance of older zircon age groups. As this study is dedicated to the sedimentary rocks of the Adrar Souttouf Massif, the information referring to the igneous and metamorphic rocks can be consulted in other works (e.g., Bea et al., 2016; Gärtner et al., 2013a, 2016a, 2016b; Montero et al., 2014, 2016, 2017; Villeneuve et al., 2006, 2015).
2.1 The sedimentary rocks of the Adrar Souttouf Massif–a brief description
The northern and western parts of the Adrar Souttouf Massif are covered by undeformed Early Cretaceous to modern siliciclastic sedimentary rocks and sediments that are partially calcified and that lie unconformably above the pre-Mesozoic rocks (Bronner et al., 1985; Leprêtre, 2015; Marchand et al., 1983; Quiroga, 1886, 1889; Rjimati et al., 2011). Pre-Cenozoic siliciclastic (meta-) sedimentary rocks, i.e. silt- and sandstone, quartzite, phyllite, conglomerate, etc., are widespread, but are often scattered all over the working area (Arribas, 1968). Carbonate rocks are very scarce. Lacking fossils and age determinations of intrusions into sedimentary successions hamper any exact timing for most of the sediments. In contrast to the igneous and ortho-metamorphic rocks, the sedimentary rocks have not been studied in detail since the pioneering works until the end of the 1960s. To date, a general stratigraphic scheme does not exist. Nevertheless, several authors briefly described the sedimentary rocks of the different units, except for the Dayet Lawda. First geochronological analyses including sediments from the Sebkha Matallah unit of the Adrar Souttouf Massif were published by Bea et al. (2016, Mesoarchaean schist) and Gärtner et al. (2017, Early Cambrian metapelite). The Dhloat Ensour unit sensu Villeneuve et al. (2006) is located at the Adrar Souttouf Massif's eastern margin, but is not part of the massif sensu stricto. The unit includes Ordovician to Devonian siliciclastics and carbonates.
2.2 Sedimentary rocks of the Sebkha Gezmayet unit
According to Arribas (1968), larger occurrences of (meta-) sedimentary rocks seem to be limited to few bands in the central and northern parts of the Sebkha Gezmayet unit, although local outcrops can be found in many of its other parts. They contain very dense quartzite, phyllite, and mylonite of possibly sedimentary origin (Villeneuve et al., 2006). Additionally, Arribas (1968) reports paragneiss occurrences of similar composition as in the Leglat Complex of the Sebkha Matallah unit to the east. Thin bands of highly metamorphosed limestone between amphibolite can be found at the border of the Sebkha Gezmayet and the northern tip of the Dayet Lawda unit. They could be analogues to the Devonian limestone layers of the Dhloat Ensour unit (Gärtner et al., 2017). In most cases, the metasedimentary rocks of the Sebkha Gezmayet unit lie (unconformably?) above a highly metamorphosed igneous basement. Almost all major contacts are of tectonic origin, which, together with the lack of fossils and the very small-scaled and patchy outcrop situation, complicates stratigraphic correlations.
3 Methods and sample selection
Here we analyse five samples of three times quartzite, one quartzitic sandstone, and one amphibolite mylonite that we collected from the northern Sebkha Gezmayet unit of the Adrar Souttouf Massif. The amphibolite is likely derived from a mafic intrusion. The four other samples can be regarded as an initial data set of the metasedimentary rocks from this area. A detailed description of the sample preparation, evaluation of the zircon grain morphologies, and U–Pb age determination using LA–ICP–MS techniques is given in the supplementary data (supplementary table S1).
4 Results
The five analysed samples can be regarded as an initial data set of the metasedimentary rocks from this area. Of them, 517 zircon grains were analysed with respect to their morphological characteristics according to Pupin (1980) and Gärtner et al. (2013b). Age determinations were derived from the U–Th–Pb isotope data of 447 analyses, of which 236 were concordant in the interval of 90–110%. Full details of all results of the isotopic measurements as well as morphological characteristics are given in supplementary table S2. The general legend for the use of the morphotype diagram of Pupin (1980) is given in supplementary figure 1, which also includes sketches of the most abundant morphotypes of this study. An overview of length, width, and roundness of all samples is provided in supplementary Figure 2. As the samples are of sedimentary origin except for sample D43, they contain a broad variety of internal textures with either magmatic or metamorphic origin (Corfu et al., 2003; Vavra, 1990, 1994; Vavra et al., 1996, 1999; supplementary figure 3). Hereafter, they will only be explicitly mentioned if they are of importance for interpretative purposes linked to the sedimentation age or a possible metamorphic overprint. Further results are shown in Fig. 2, and are briefly described below.
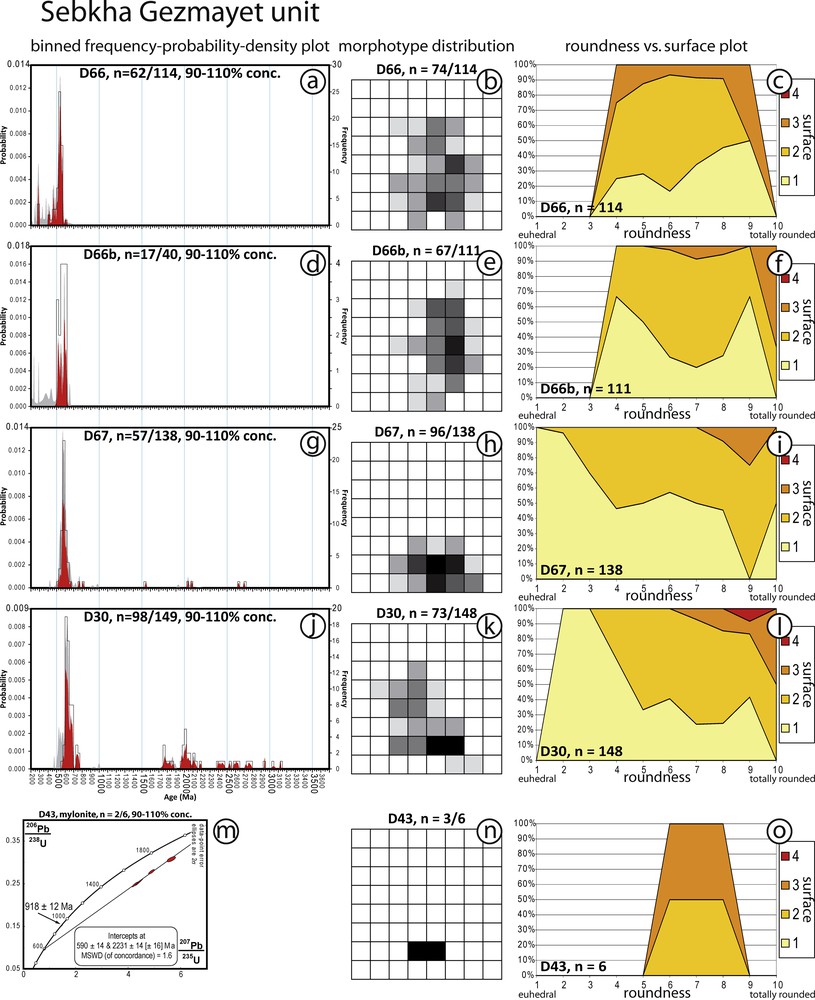
Zircon age distribution pattern [a, d, g, j] applying binned frequency probability–density plots (bin width = 25 Ma, the grey curve represents discordant analyses), except for sample D43 [m] which shows a concordia plot, zircon morphology distribution patterns in accordance to supplementary Figure 1 (Pupin, 1980) [b, e, h, k, n], and a roundness (classes 1–10, euhedral to completely rounded, x-axis) vs. surface (percentage of classes 1–4, entirely smooth to totally pitted, y-axis) plot using the classification of Gärtner et al. (2013b) [c, f, i, l, o] of samples D30, D43, D66, D66b, and D67 from the Sebkha Gezmayet unit. The samples are arranged from the likely oldest rock at the bottom to the possible youngest on top (see discussion in the text).
4.1 Sample D30, quartzite, N22°52′30.60″, W15°18′04.20″
This red-brown, fine-grained, massive quartzite is crossed by secondary quartz veinlets and lies unconformably above a leucocratic granite. In total, 148 zircon grains of this rock were analysed with respect to their morphology. Thus, their mean length, width, and roundness are 122 and 64 μm, and 7.02, respectively. More than 90% of all grains show no or only few surficial collision marks, while 8% exhibit moderate pits, and only one grain is totally covered by them. The morphotypes of 73 grains could be identified, whereas S24 and S25 are most abundant and represent almost 50% of the population. Age determinations were done at 149 spots on 148 zircon grains, of which 98 yielded concordant ages between 574 ± 14 and 3129 ± 12 Ma. The five youngest grains overlap in error and were used to calculate a concordia age at 592 ± 9 Ma, the maximum age of sedimentation. Most of the grains have ages between ca. 600 and 700 Ma, while subpeaks occur from ca. 1750–1800 Ma, and ca. 2000–2075 Ma. Th–U values for all analyses and the concordant ones are between 1.55 and 0.09.
4.2 Sample D43, amphibolite mylonite, N22°36′27.66″, W15°34′06.60″
This strongly sheared, very fine-grained, black amphibolite mylonite sample contained six zircon grains. They have a mean length, width, and roundness of 87 and 54 μm, and 7.00, respectively. The grains surfaces show few to moderate collision marks. Three grains had definable morphotypes, which are S23 and S24. Two of the six zircon grains yielded concordant ages at 590 ± 8 and 918 ± 12 Ma. The remaining four analyses and the younger concordant age form a discordia with a lower intercept at 590 ± 14 and an upper intercept at 2231 ± 14 Ma. The obtained Th–U values range from 1.09 to 0.38 for all, and from 0.67 to 0.42 for the concordant grains. According to the data and with respect to the well-defined discordia, the precursor of this amphibolite mylonite intruded at 590 ± 14 Ma.
4.3 Sample D66, quartzitic sandstone, N22°38′30.72″, W15°25′46.92″
This red quartzitic sandstone is very fine-grained and shows strong petrographic similarities to sample D30. The rock forms thin plates of approximately 5 cm in thickness and lies unconformably above strongly deformed mica schist, brown quartz phyllite, and locally mylonitic orthogneiss of granitic composition. The 114 zircon grains that were extracted from this sample have mean values of length, width, and roundness of 84 and 52 μm, and 6.20, respectively. Almost 90% of all grains show no or only few characteristics of collision resulting from transport processes, whereas the remaining grains show a medium occurrence of such features. Due to the relatively low roundness, a comparatively high number of 74 zircon grains were definable with respect to their morphotype. Characterised by high variability, the S15, S24, and S25 types are most abundant, making up about 31% of all definable grains. U–Th–Pb isotopic data were gained from 114 grains, of which 62 gave concordant ages between 284 ± 6 and 609 ± 15 Ma. The maximum age of sedimentation at 287 ± 3 Ma was calculated from the four youngest grains, which are almost entirely homogenised pointing to intense metamorphic overprint of the source rock (e.g., Corfu et al., 2003). The main peak is composed of zircon ages between 520 and 570 Ma. The obtained Th–U values vary from 7.14 to 0.07 for all, and from 1.39 to 0.08 for the concordant grains.
4.4 Sample D66b, quartzite, N22°38′30.60″, W15°25′48.72″
The white-to-brown, fine-grained, laminated quartzite D66b lies unconformably above a strongly deformed amphibolite, whose deformed feldspars indicate a sinistral NE strike-slip movement. This sediment shows slaty cleavage and contains a comparably diverse spectrum of accessory minerals (apatite, rutile, scarce pyroxene and amphibole). Of this rock, 111 zircon grains were extracted. Their mean length and width, is 86 and 51 μm, while the mean roundness is 6.70. A number of 104 grains exhibits no or only few surficial collision marks, while another seven grains have a medium amount of them. Morphotypes could be defined on 67 grains and spread over most of the Pupin diagram. Thereof, the most abundant types are S4, S5, S10, S15, and S20, making up more than 50% of the entire definable population. Isotopic age determinations were done on 40 grains, of which 17 gave concordant ages in the range of 516 ± 31 to 613 ± 11 Ma. All of them form just one main peak, of which the youngest five grains overlap in error and allow the calculation of a concordia age at 526 ± 5 Ma. The latter one is regarded as the maximum age of sedimentation. The Th–U values are between 2.32 and 0.06 for all, and between 0.51 and 0.09 for the concordant analyses.
4.5 Sample D67, quartzite N22°36’27.60”, W15°15’21.00”
This dark grey, very fine-grained, layered, strongly deformed quartzite unconformably overlies a strongly deformed, partly mylonitic reddish granitoid, and contained 138 zircon grains. Their mean length, width, as well as the roundness is 91 and 52 μm, and 4.30, respectively. Only a minor population of two grains shows a medium amount of surficial collision marks, while the rest exhibits few or none. Remarkably, this sample has two distinct groups of roundness, of which the first is between 2 and 4, whereas the second ranges from 6 to 9. Ninety-six grains could be analysed with respect to their morphotype, with highest abundances of the S23, S24, S25, and J4 types, which represent about 67% of the overall definable zircon grains. Concordant ages were derived from 57 of 138 zircon grains. These ages range from 521 ± 9 to 2703 ± 14 Ma. Although it is a single analysis, the youngest detrital zircon age is interpreted as the maximum age of sedimentation. Such youngest single grain ages (YSG) are mostly very close to the youngest detrital zircon age derived from several analyses and can be regarded as good approximation of the maximum age of deposition (Dickinson and Gehrels, 2009). A major peak is between ca. 521 and 629 Ma, while minor zircon occurrences could be found at ca. 766, 811, 1550, 2043, 2090, 2638, and 2703 Ma. The obtained Th–U elemental ratios vary from 1.89 to 0.04 for all, and 1.18 to 0.27 for the concordant analyses.
5 Discussion
The application of multiple morphological parameters also facilitates the use of zircon grains with discordant data for some interpretative purposes, which otherwise would have been discarded and is used as additional tool to narrow down potential source rocks (e.g., Markwitz and Kirkland, 2018). The distribution of roundness gives a general idea about the distance of transport, the morphotypes may be representative of the temperature of the host magma (Pupin, 1980), but likely not of its chemical composition (Markwitz and Kirkland, 2018), and the surficial characteristics could bear a hint to the medium of transport (Gärtner et al., 2013b). Lacking fossils and volcanic ash layers in the sedimentary rocks do not allow an exact timing of the deposition or a biostratigraphic correlation. Thus, the maximum age of deposition that is derived from the youngest zircon population may give a first hint to the possible stratigraphic position.
Depending on the tectonic setting, the maximum ages of deposition can show large deviations to the real time of sediment accumulation, which is assumed to be largest within intraplate, e.g., passive margin, conditions (Cawood et al., 2012). The individual zircon age distribution pattern of each sample can additionally be used to characterise the hinterland of a certain sample in time applying a provenance study (e.g., Andersen, 2005; Cawood et al., 2003; Fedo et al., 2003; Thomas, 2011, and references therein). Variations in this pattern are a good indicator for potential changes in a basins catchment area through space and time (e.g., Clift et al., 2010; Kimbrough et al., 2015; Sláma and Košler, 2012). Combining the U–Th–Pb maximum ages of sedimentation, detrital zircon age spectra, Th–U elemental ratios, and morphological characteristics allow extracting an individual zircon pattern for the Sebkha Gezmayet unit. Accordingly, the geologic history of the unit, as well as the hypothetical relationship to the Meguma terrane can be reconstructed based on zircon, which is possibly the best approximation until detailed field work is possible.
5.1 Sediment deposition in the Sebkha Gezmayet unit since the Ediacaran
The Sebkha Gezmayet unit hosts at least two generations of Ediacaran granitoid intrusions. Thereof, the older ones were emplaced between ca. 605 and 588 Ma, whereas the younger ones intruded between ca. 583 and 571 Ma (Bea et al., 2017; Gärtner et al., 2013a). Plutons of the latter seem to occur only east of the Tageneddest Fault (Bea et al., 2017; Gärtner et al., 2013a). Amphibolite mylonite D43 likely intruded at about 590 Ma coeval with the older granitoid generation, although lying in an area which is dominated by the younger granitoid intrusions (Bea et al., 2017) and is discordantly overlain by sedimentary rocks. The comparatively high roundness of zircon grains could be a result of magmatic corrosion in a mafic magma, which was depleted in Zr and occurs also in other mafic rocks of the Adrar Souttouf Massif (cf. Gärtner et al., 2016a, and references therein). However, this remains an open question due to the low amount of zircon grains. Additionally, the grain size is relatively small (supplementary figure 2). In general, the new geochronological results resemble the already published data for the igneous rocks of this unit (Bea et al., 2017; Gärtner et al., 2013a).
The possibly oldest sediment of this unit (D30) has a maximum age of sedimentation at 592 ± 9 Ma, calculated from the five youngest zircon grains overlapping within errors. This could link the youngest zircon population to the older Ediacaran granitoid group, but implies a deposition of the sedimentary rock after their exhumation followed by erosion. The youngest concordant grain at 574 ± 14 Ma (99% concordance), either belonging to the older granitoid generation (within errors), or to potential volcanic rocks corresponding to the younger magmatic pulse, limits the maximum sedimentation age at about 574 Ma. This is thought to be more realistic than the conservative maximum age estimation at 592 ± 9 Ma. A younger age, similar to that of samples D66 and D66b (see below) remains a possible option. D30 is the only sample with appreciable older zircon age populations (Fig. 2). Thus, either igneous basement rocks or precursory sediments containing Palaeoproterozoic zircon grains were likely exposed and eroded during the time of initial sediment accumulation on the Sebkha Gezmayet unit. Palaeoproterozoic zircon ages and minor proportions of Archaean grains point to a West African Craton provenance (see compilation in Gärtner et al., 2017), which would imply a sedimentary transport from easterly directions prior to the mixing with Late Neoproterozoic zircon grains from the pan-African belts at the craton's western margin. This hypothesis is corroborated by the highest mean value of roundness obtained from all samples of this unit, which indicates long distance transport and/or recycling (e.g., Belov, 1993; Gärtner et al., 2017; Meinhold et al., 2008). A mixture of cratonal zircon grains that are mostly characterised by high-temperature morphologies, and zircon crystals formed during orogenic episodes, which show a broad variety of morphotypes (Gärtner, 2017) is suggested. Remarkably, some grains of this sample completely covered by surficial collision marks, and a mean grain size that is significantly larger than in all other samples, which is a unique feature within the Sebkha Gezmayet unit and may hint to higher energy of the transport medium (Cui et al., 2016; Fig. 2).
The next level of sedimentary rocks with respect to the maximum age of deposition comprises samples D67 and D66b, which have, within errors, the same Mid Cambrian maximum age of sedimentation at 521 ± 9 and 526 ± 5 Ma, respectively (Fig. 2). Such ages can be associated with the coeval magmatism that was found in the Sebkha Gezmayet and Sebkha Matallah units (Bea et al., 2016; Gärtner et al., 2013a), and which are likely related to a rifting event (Bea et al., 2016; Gärtner et al., 2017; Haissen et al., 2017). Although local effects cannot be ruled out, sample D67 is interpreted to be slightly older than D66b for the following reasons: some few Palaeoproterozoic grains found in D67 suggest some input caused by the recycling of sediments comparable to D30. Furthermore, the curve of single grain roundness shows two distinct peaks (supplementary figure 2). This leads to the assumption that the less rounded Ediacaran zircon grains result from significant input of freshly weathered igneous first-cycle material from the immediate surroundings, while the more rounded grains suffered several cycles of sediment recycling and/or long distant transport. This is well in line with the uniform grain size for all samples of the Sebkha Gezmayet unit, except D30, and the homogeneous distribution of zircon morphotypes that exclusively indicate high crystallisation temperatures (Pupin, 1980). Such a strong concentration of morphotypes is unique amongst the studied sedimentary samples and points to a very local group of host rocks (van Hattum et al., 2003; Konsa and Puura, 1999). If the catchment area was larger and its host rocks more diverse, a larger spread of morphotypes would have been expectable, particularly in areas affected by orogenic events (e.g., Gärtner, 2017). Finally, the generally smooth surfaces of the zircon grains are a hint to a short-distance transport with only few collisions (Gärtner et al., 2013b). In contrast, zircon grains of sample D66b are more rounded, expose a higher number of surficial pits, and show a broad variety of morphotypes. Additionally, they have absolutely no indication of older than Mid Ediacaran zircon detritus (Fig. 2). However, the value of mean roundness and surficial pitting is significantly smaller than for most of the other sediments of this study, which emphasises the shorter distance of transport on the whole (Belov, 1993; Gärtner et al., 2013b; Zoleikhaei et al., 2016). Because of their likely very local provenance, and with respect to the signs of recycling, sediments D67 and D66b, and eventually even D30, are interpreted to have been deposited in a sedimentary basin of very limited areal extent that was fed by regional sources. Such basin development is commonly linked with an extensional tectonic regimen, which often takes place during the late stages of orogenic evolution or during the onset of rifting (Pedrera et al., 2010). This would be in accordance to the assumed rifting of the Oued Togba and Sebkha Gezmayet units in Mid-Cambrian times (Gärtner et al., 2013a, 2016a, 2017) and the coeval rift related magmatism in the Sebkha Matallah unit (Bea et al., 2016).
The youngest sediment of the Sebkha Gezmayet unit could be D66, whose maximum age of sedimentation at 287 ± 3 Ma suggests an Early to Mid-Permian or even later deposition. However, the slight metamorphic overprint of the rock limits the absolute age to the context of the terminating Variscan–Alleghanian orogeny, which was the last major tectonic event in the studied region. The existence of small zircon grains with intact oscillatory magmatic zoning led to the assumption that the low-grade metamorphic overprint did not affect the detrital zircon population of this rock. Consequently, the youngest, completely homogenised zircon population likely originates from a metamorphosed source. The zircon spectrum resembles that of sample D66b almost completely, and the only differences to the other metasedimentary rocks are the relative abundance of zircon grains with ages below ca. 500 Ma (Fig. 2). They can be grouped as follows: ca. 284–291, ca. 405–430, ca. 460–470, and ca. 480–500 Ma, of which the latter have both yet only been recognised as scarce inheritance in the magmatic rocks of the Sebkha Gezmayet unit (Gärtner et al., 2013a). The ca. 400 Ma zircon age is well known as inherited component from the Sebkha Gezmayet unit (Gärtner et al., 2013a), while the Early Permian zircon age group is thought to be derived from the already described quartzitic diorite that likely intruded the unit in the course of the Variscan Orogeny (Gärtner et al., 2013a). Remarkably, the youngest zircon grains that define the maximum age of deposition are very homogeneous in all investigated characteristics (supplementary table S2). Therefore, a common, nearby source of these grains is suggested, although the high value of roundness indicates some recycling (e.g., Belov, 1993; Gärtner et al., 2017; Meinhold et al., 2008). As the sedimentary rocks are coeval or a bit younger, they likely were deposited during a late syn-orogenic stage (Martínez Catálan et al., 2016).
5.2 Palaeogeographic conditions for sedimentation in the Sebkha Gezmayet unit
All sedimentary rocks exhibit a very homogeneous spectrum of zircon ages that varies just in the youngest population, except for sample D30, which is the only sample with a significant amount of pre-pan-African zircon ages. Remarkably, Mesoproterozoic zircon could not be found in any of the samples, which excludes the Oued Togba unit as potential source for the studied sedimentary rocks, although both units were likely juxtaposed since the pan-African orogeny (Gärtner et al., 2013a, 2015). The potential Ediacaran connection of the Oued Togba and Sebkha Gezmayet units is reconstructed from coeval Ediacaran magmatism, parallel metamorphism in the Oued Togba unit, and similar Hf-isotope composition of shared detrital zircon populations of Late Ediacaran sedimentary rocks on both units (Gärtner, 2017; Gärtner et al., 2013a, 2016b). However, a later, possible Ordovician to Silurian juxtaposition of the Oued Togba and Sebkha Gezmayet units cannot be fully excluded. The obtained U–Pb data point out a strong relation of the Sebkha Gezmayet unit with a West African Craton-type source in general (Ennih and Liégeois, 2008; Gärtner et al., 2017; Linnemann et al., 2011). This does not apply for the Late Cambrian to Devonian zircon grains, for which no corresponding tectonic event is reported at the West African Craton. The existence of these zircon populations was linked to a collision of the Oued Togba and Sebkha Gezmayet units to the periphery of another craton, likely Laurussia, during which several intrusions were emplaced (Gärtner et al., 2013a). Additionally, widespread metamorphic overprint occurred in the Oued Togba unit during the Pragian (Bea et al., 2017). These are arguments for a separate geologic evolution of the Oued Togba and Sebkha Gezmayet units after a proposed Cambrian rifting of both units away from the West African Craton (Bea et al., 2016; Gärtner et al., 2013a, 2016a).
A mixture of zircon grains derived from the local post-pan-African intrusions, the surrounding, steadily eroding Ediacaran magmatic rocks, and reworked Palaeoproterozoic grains from pre-Cryogenian-type sediments of the Reguibat Shield or the Taoudeni basin, which are characterised by a lack of Mesoproterozoic zircon (Bradley et al., 2015; Gärtner et al., 2017; Straathof et al., 2010; etc.), is very likely, as indicated by the comparatively high roundness of the Palaeoproterozoic and the often less rounded Ediacaran grains (supplementary figure 4). On the other hand, the post-Cryogenian sediments to the southwest and the west of the studied area can be excluded as sources due to their significant amount of Mesoproterozoic detrital zircon grains (Bradley et al., 2015; Gärtner, 2017; Gärtner et al., 2017; Straathof et al., 2010). It has to be noted that up to now no Mesoproterozoic igneous rock is known from the West African Craton. The input of Mesoproterozoic detrital zircon with a main peak between ca. 1.2 and 1.3 Ga likely starts in the Cryogenian (Bradley et al., 2015; Gärtner, 2017; Gärtner et al., 2013a; Straathof, 2011; Straathof et al., 2010). A possible model that tries to explain the sudden input of these grains requires an outboard source and will be discussed elsewhere. The potential magmatic sources of Palaeoproterozoic zircon grains occur widespread on the West African Craton, but the Sfariat Belt of the Reguibat Shield (Schofield et al., 2006) is the closest yet known region. Other potential primary sources are the Palaeoproterozoic basement inliers of the Anti-Atlas, the Kédougou-Kéniéba Inlier, or the Leo-Man Shield (see compilations of Gärtner et al., 2017 for age spectra and supplementary Figure 5 for locations).
The assumption that the maximum ages of sedimentation derived from the detrital zircon ages are close to the real age of sedimentation results in very steep cumulative probability curves (Cawood et al., 2012; Fig. 3). Consequently, the patterns obtained for samples D66b and D67 point to a convergent setting during deposition, while sample D66 was likely deposited in a collisional regimen. Finally, the data for sample D30 is interpreted to represent an extensional setting (Cawood et al., 2012). This is well in line with earlier assumptions that contain a Cambrian rifting (e.g., Bea et al., 2016) with subsequent drifting and collisional phases during the Appalachian and Variscan-Alleghanian orogenies (Gärtner et al., 2013a, 2016a, 2016b).
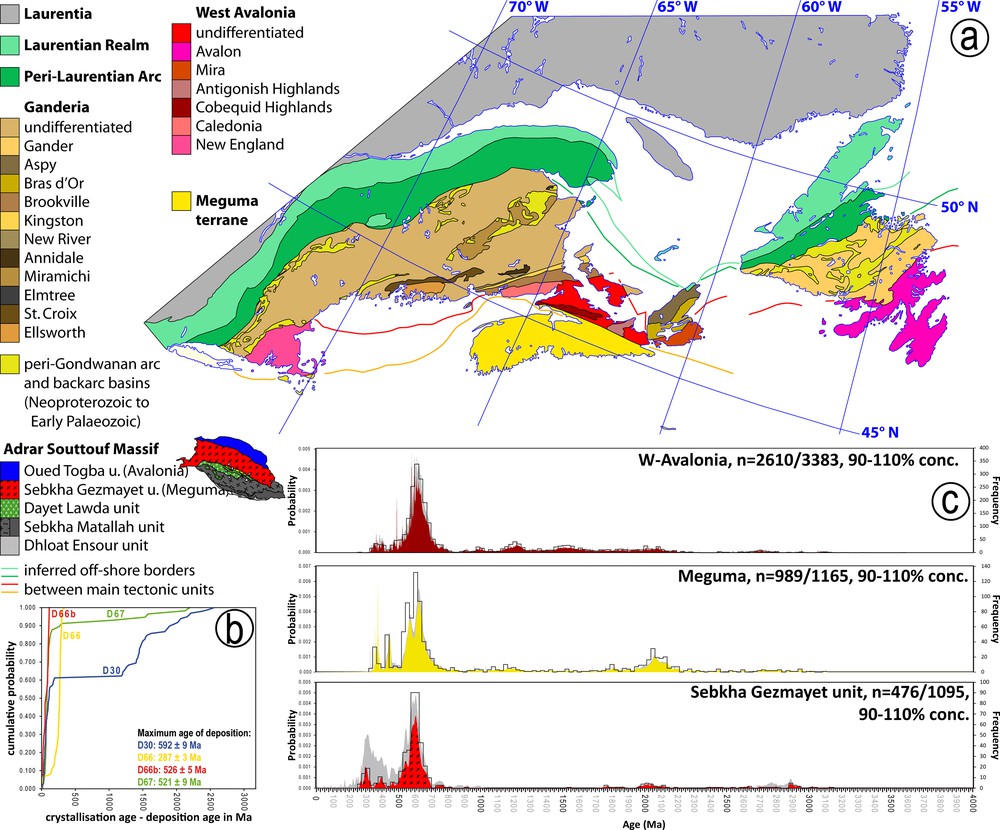
The possible positions of the Adrar Souttouf Massif during the Late Palaeozoic Pangaea configuration (Torsvik and Cocks, 2017) (a), the cumulative probability plots for the four sedimentary samples according to Cawood et al. (2012) (b), and comparison of published U–Pb zircon ages of the West Avalonian terranes (c) (literature cited in Gärtner et al., 2016b; added with data from Doig et al., 1993; Grammatikopolus et al., 1995; Henderson et al., 2016; Murphy et al., 2004; Pu et al., 2016; Thompson et al., 1996; Zartman and Naylor, 1984) and the Meguma terrane (literature cited in Gärtner et al., 2016b; added with data from Keppie et al., 1993; Kontak et al., 2004; Tate et al., 1997; White et al., 2018) in comparison to the zircon record of the Sebkha Gezmayet unit of the Adrar Souttouf Massif (Gärtner et al., 2013a, this study; bin width for all plots = 25 Ma, the grey curve represents discordant analyses).
The assumptions of Pollock et al. (2012), Schenk (1997), and Waldron et al. (2009, 2011) include a West African affinity of the Meguma terrane, while a Meguma affinity is proposed for the Sebkha Gezmayet unit (Gärtner et al., 2013a). The newly obtained overall zircon age spectrum of the Sebkha Gezmayet unit is very close to that of the Meguma terrane (Fig. 3) and corroborates the already assumed relation between both crustal blocks. Furthermore, the Latest Cambrian to Devonian zircon grains have no known igneous source in the West African Craton, but are abundant in the Meguma terrane (e.g., MacLean et al., 2003; White et al., 2018; Fig. 3). This would be in line with the general set-up of the Adrar Souttouf Massif with the Avalonia-related Oued Togba unit in the west and a trailing Meguma-like Sebkha Gezmayet unit to the east, and the succession of the peri-Gondwanan terranes along the eastern coast of North America (Hibbard et al., 2006). The global palaeogeography during the assembly of Pangaea (e.g., Torsvik and Cocks, 2017; Torsvik et al., 2008; supplementary figure 5) also allows a location of the Adrar Souttouf Massif south of New England (Fig. 3, supplementary figure 5). Finally, the high grade of local material incorporation and sedimentary reworking, particularly of the post-Mid Cambrian samples D66b and D67, could be a hint to an isolated position off cratonal margins due to the opening of the Rheic Ocean (Landing, 2005; Murphy et al., 2010; Nance et al., 2012; Satkoski et al., 2010).
6 Conclusions
The Sebkha Gezmayet unit is thought to have previously been linked with the Meguma terrane (Gärtner et al., 2013a). This interpretation remains valid, as the newly obtained zircon spectrum corresponds well to the already gained data and the published zircon data of the Meguma terrane (Fig. 3). Accordingly, the position of the Adrar Souttouf in the pre-Atlantic Pangaean configuration was likely (modern) south of New England. Furthermore, different age groups of sediments in the Sebkha Gezmayet unit could be described for the first time. Combined with zircon morphology and the detrital zircon ages, a possible scenario of the sedimentary environment is postulated until detailed fieldwork is possible.
Acknowledgements
This study was supported by partial funding provided by the Academy Hassan II for Science and Technology of the Kingdom of Morocco through Project No. SDU 02/2012-2015 awarded to N.Y. We thank the civil and military authorities in Dakhla, Aoucert, and Tichla (southern Morocco) who facilitated our stay and our movement in these difficult areas. Thanks also to A. El Archi (Chouaïb Doukkali University), E.-C. Rjimati, and A. Zemmouri (Geological Survey of Morocco) for advices and guidance in the field. The authors are also grateful for the comments and suggestions of Yvette Kuiper and of an anonymous reviewer, as well as of André Michard, which helped improve the quality of the manuscript.