1 Introduction
Nanotechnology is no more an emerging technology. More than 2000 nanoproducts are already on the market (Nanowerk, 2012). The variety of nanoproducts is large, going from cosmetics to electronics, paints to food and health. The complexity of these nanoproducts increases in terms of structural and dynamic complexity: (1) passive nanostructures, (2) active nanostructures, (3) nanosystems, (4) molecular nanosystems (Roco, 2011).
To date, new nanomaterials, graphene and core-shell nanoparticles used as vectors for biological applications, are the object of intense research.
Nevertheless, nanotechnologies are at the heart of societal interest and also instill fear since more than 15 years (e.g., ETC, and other citizen groups). One of the first reasons for this fear is the size of the nanoparticles that is seen as the parameter that could induce health and environmental problems. In this regard, governments and politic organizations have decided to fund national and European scientific projects about the risk assessment of nanotechnology in order to decide regulation politics through OECD countries. For instance, regulation is presently evolving to take into account nanoscale in products (for instance REACH).
Compared to nano-toxicity, nano-ecotoxicity is seen as a new discipline. While many biologists considered that nanomaterials have the same environmental behavior as “classic” pollutants, as organic molecules or metals, physicists, chemists, geologists, physical chemists considered the structure–size relationship as being at the heart of their scientific and technological interests. The challenge in nano-ecotoxicity is to work with an interdisciplinary approach with the weak information available concerning the quantities of nanomaterial that could be released all along the life cycle, taking into account the complexity of the environmental matrices. This requires to evaluate the exposure, (bio)transformations, bio(distribution) and biological effects of the nanomaterials at environmentally relevant doses and on the long term. To go further in this discussion, an example coming from three engineered nanomaterials will be presented in this paper. This choice is based considering their importance in terms of production quantity and applications (TiO2- and CeO2-based nanomaterials) or due to the large number of products present on the market (Ag-based nanomaterials) (Gaffet et al., 2014). Moreover, these three nanomaterials are characterized by different kinetics and mechanisms of transformation. While TiO2 is chemically stable, CeO2 and Ag can be transformed through reduction and oxidation, respectively leading to dissolution and change of reactivity. This could imply large differences of effects on the biota and on environmental risks.
2 An interdisciplinary approach to forecast the risk
The nanotechnology development and the societal demand in terms of risk forecasting are certainly, for the first time in the OECD countries, the mean to develop interdisciplinary researches between science and society in order to build answers and to develop safer-by-design nanoproduct approaches implying the control all along the life cycle from the occupational activity to the waste production and behavior (Fig. 1).
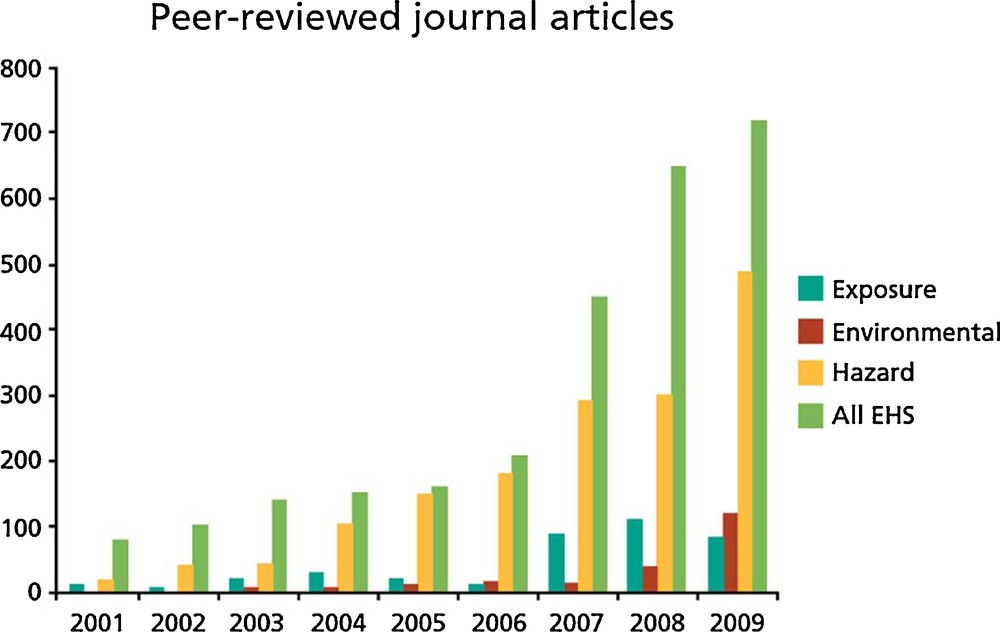
(Colour online). Number of peer-reviewed publications.
From: EHS issues of nanomaterials hazard, NanoAlert Service, 2011.
The unknowns concerning nanomaterials include not only the nature of nanomaterials that may find their way into commerce, the properties of these materials, and even the uses and handling practices for nanomaterials, but also environmental transport, persistence vs transformation, and bioactivities, including toxicity.
Risk assessment, which is a result of an evaluation of the exposure and hazard, has been essentially studied through the hazard. Exposure and transformation have not been the object of many studies. Nevertheless, exposure has been the primary criterion for prioritizing EHS (Environment, Health, and Safety) (Wiesner and Bottero, 2011). Ecotoxicology represents the majority of the peer-reviewed publications between 2001 and 2009 compared to exposure or environmental topics.
3 How are developed the environmental risk studies
The complexity of assessing the environmental risk of nanomaterials is due to the fact that the exposure is the result of:
- • the transport and transfer of nanoparticles, for instance from soil to plants or from water to the food chain;
- • the interactions of the nanomaterials between them or with the environment (e.g., interactions with other particles, organic molecules, cellular membranes) leading to the formation or not of aggregates (homo- or hetero-aggregates) that can impact the initial reactivity of the primary nanoparticles (Hotze et al., 2010a,b);
- • the transformation of the nanoparticles due to reduction, oxidation, dissolution, leading to the formation of new mineral phases that could have different reactivity compared to primary nanoparticles;
- • the behavior of the coating associated with the synthesis or the interactions with environmental organic molecules, which can affect aggregation states and reactivity, and consequently the exposure and impacts of nanomaterials.
Fig. 2 shows the sources of nanoparticles and the risks for organisms and ecosystems in relation with exposure and transformation during and after technology used for water treatment and land application. The unknowns concerning nanomaterials include not only the nature of nanomaterials that may find their way into commerce, the properties of these materials, and even the uses and handling practices for nanomaterials, but also the environmental transport, persistence vs transformation, and bioactivities, including toxicity.
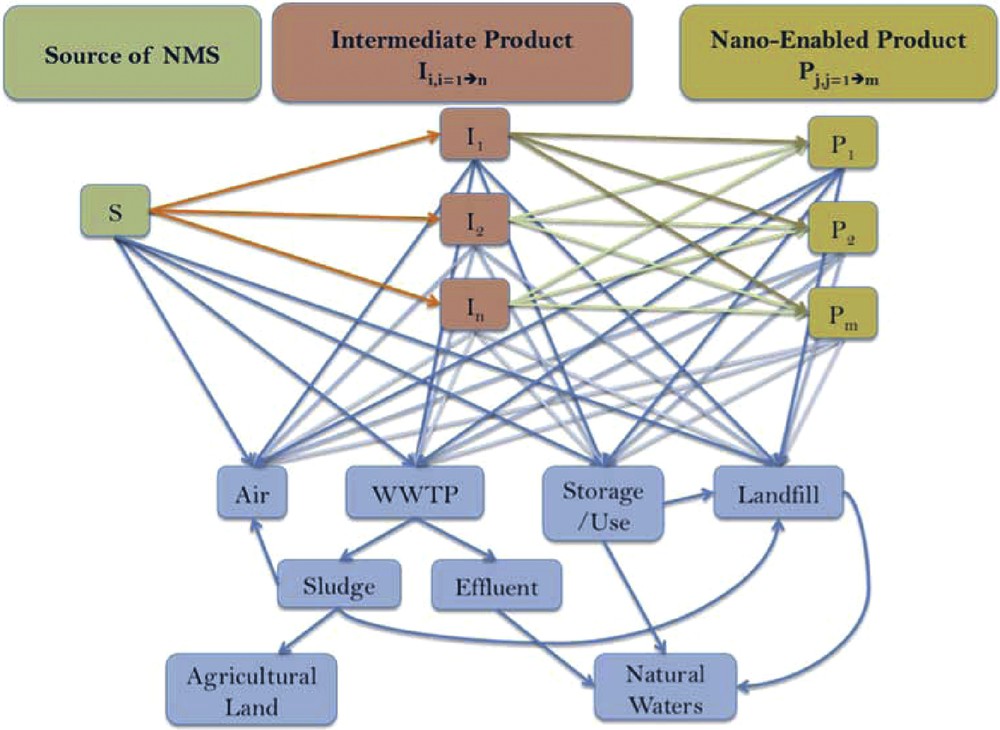
(Colour online). Simplified flow of nanomaterials through the production process and the environment; WWTP: Waste Water Treatment Plant.
Fig. 3 synthesizes the different steps of the environmental behavior of the nanomaterials from their interactions with abiotic media to the biological effects following various steps…, but also their transformation depending on Eh and pH, on the medium, the biota activity. Such complex reactivity needs to be approached associating various disciplines, as chemistry, physical chemistry – particularly surface chemistry –, geosciences (pedology and mineralogy), hydrology and microbiology, biology, ecotoxicology, etc.
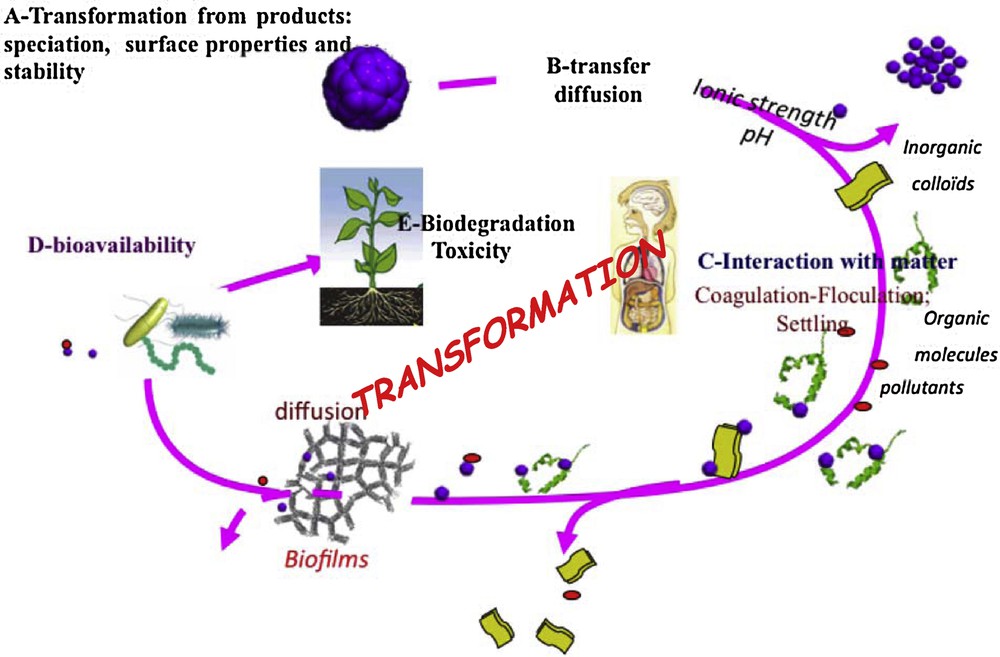
(Colour online). Transfer, transformation and flux of Nms within the environment and links between the different disciplines that need to be developed.
To try to reveal these complex behaviors, three engineered nanomaterials were chosen: TiO2, which is chemically stable, and CeO2 and Ag0, which present transformations through reduction and oxidation, respectively leading to dissolution and followed by a change in reactivity due to the affinity of the ions for molecules present in the media…
The transformations could imply large differences between their effects on the biota. This choice is also led by considering their importance in terms of production quantity and applications (TiO2 and CeO2) or the large number of products present on the market (Ag0) (Gaffet et al., 2014).
4 What are the fluxes of nanomaterials in the environmental compartments?
Recent works by Keller et al. (2013); Gambogi (2006); Gottschalk et al. (2009), (2010a,b), (2013) tried to evaluate the production and fluxes of nanomaterials in the environment in terms of mass. The production of TiO2, CeO2 and Ag0 are evaluated to 88,000 ton/year, 10,000 ton/year and 452 ton/year, respectively (Keller et al., 2013). Kaegi et al. (2008) have also experimentally measured the release of TiO2 from a true wall and a model wall. The release of TiO2 was ∼600 μg/L for a model and around 10 μg/L for a true façade.
The calculated environmental and WWTP concentrations are displayed in Table 1.
Concentrations of TiO2, Ag0 and CeO2 in the environmental medium.
TiO2 | Ag0 | CeO2 | |
Surface water (μg/L) | 10−2–10 | 10−5–10−1 | 10−2 |
WWTP effluents(μg/L) | 10 | 10−2–10−1 | < 10−4 |
Biosolids (μg/kg) | 102–103 | 10−2–1 | 10−6 |
Sediments (μg/kg) | 103–104 | ∼10 | NA |
Soils (μg/kg) | 10−4–103 | 10−4–10 | ∼103 |
Atmosphere (μg/L) | 10−3–10−2 | 10−5 | 10−4–10−3 |
The WWTP is, in the OECD countries, an important pathway for treating urban and industrial effluents. From Keller et al. (2013), approximately 40% of TiO2 and Ag0 and 11% for CeO2 are treated by WWTP. The question now is what is the efficacy of WWTP processes for blocking Nms.
From experimental and modelling approaches using data from Keller et al. (2013) and Hendren et al. (2011), Barton (2014) and Barton et al. (2014a,b) evaluated the WWTP effluent and biosolids concentrations for TiO2, coated Ag0 and CeO2 by considering also the transformation (reduction and oxidation of CeO2, Ag0, respectively) evaluated from experiments and data obtained by Kaegi et al. (2011, 2013). Table 2 shows the values depending on the transformation and nature of the coating of Ag0 and CeO2. The transformations of CeO2 by reduction and formation of citrate–Ce(III) and cerium(III)–phosphate phases have been experimentally evaluated by using synchrotron beamlines (XANES) (Barton, 2014, and Barton et al., 2014a). The same approaches concerning Ag0 have been made in order to evaluate Ag+/Ag0 ratios and the transformation in Ag2S within a WWTP pilot (Kaegi et al., 2011, 2013). The results have shown that Ag0 is totally transformed into Ag2S, whereas ∼80% of CeO2 is reduced.
Influent, effluent and biosolids concentrations of different Ag0, CeO2 and TiO2 treated in a WWTP.
Np | Influent (mg/L) | Effluent (mg/L) | Biosolids concentration (mg/g) |
PVP–Ag 40 nm | 1.2·10−3 ± 10% | 1.17·10−6 | 1.49·10−6 |
PVP–Ag 8 nm | 1.2·10−3 ± 10% | 1.17·10−6 | 1.49·10−6 |
GA–Ag 25 nm | 1.2·10−3 ± 10% | 1.16·10−6 | 1.49·10−6 |
GA–Ag 6 nm | 1.2·10−3 ± 10% | 1.16·10−6 | 1.49·10−6 |
TiO2 | 0.29 ± 10% | 1.9·10−3 | 0.244 |
Pristine CeO2 | 6.72·10−3 ± 10% | 2.229·10−4 | 1.45·10−4 |
Citrate-coated CeO2 | 6.72·10−3 ± 10% | 2.448·10−4 | 1.44·10−3 |
The affinity of nanomaterials for biosolids was calculated by using sorption isotherms of Nms by bio-flocs from industrial WWTP samples at the steady state and versus the contact time (Table 3). Different equations were used in order to calculate the attachment efficacy α and particularly Smoluchowski's equation:
(1) |
(2) |
Calculation of the attachment efficacy α of various nanomaterials for WWTP bioflocs.
Nm | Final removal | Affinity α |
GA Ag | 71.1 ± 0.4 | 0.0017 |
PVP Ag | 94.8 ± 2.2 | 0.0120 |
Pristine CeO2 | 90.8 ± 1.7 | 0.0064 |
Citrate CeO2 | 86.4 ± 1.3 | 0.0023 |
TiO2 | 95.1 ± 1.7 | 0.0080 |
These results show that WWTP are efficient to remove a very large mass (number) of nanomaterials from urban water. The mass of nanomaterials rejected in the effluent is very low and some of them are transformed in nanomaterials that are very different from the initial ones (for instance, Ag0 is transformed into Ag2S, CeO2 into CePO4). Nevertheless, one of the problems is the use of bio-sludge for agricultural applications.
5 How could nanomaterials affect water resources?
Both groundwater and surface water need to be considered when studying the interactions between water resources and nanomaterials. This requires to thoroughly characterize (i) the transfer of nanomaterials in porous media and (ii) the colloidal behavior of nanomaterials in surface waters through homo- and hetero-aggregation.
Lecoanet et al. (2004) were the first to evaluate the transfer of nanomaterials in well-defined porous medium in a packed column constituted of glass beads. The transfer of TiO2 appeared to be very limited in this study. In a later study, Solovitch et al. (2010) highlighted the homo-aggregation versus deposition regarding the transfers of TiO2 nanomaterials in porous media. They showed that the interaction energy between TiO2 and sand particles explain the transfer mechanisms and homo-aggregation. The size analysis of the eluted particles show an aggregation of the initial nanomaterials at the environmental pH.
More recently, transfer experiments of coated and uncoated Ag nanomaterials in porous media in the presence of a biofilm (Xiao and Wiesner, 2013) allowed one to calculate the affinity α values Eq. (3), where rC is the geometric radius of the collector (here glass beads), ɛ and L the porosity and length of the porous medium, and C/C0 the ratio of nanomaterial concentration in the effluent vs the influent, η0 being the single-collector efficiency (Tufenkji and Elimelech, 2004):
(3) |
The values of α are between ∼0.01 and ∼0.03, depending on the coating and the presence of Ca++ cations. The values of α when glass beads are coated with bacteria proteins are lower than that calculated for clean glass beads. This is confirmed by a work of Kaegi et al. (2013), in which the authors evaluate the fate and transformation of PVP-coated Ag0 in an urban wastewater system. They observe a transfer of Ag0 in the sewer system without loss, proving that its affinity for biofilms is very low. Similarly, the coating of glass beads particle collectors by iron oxide increases the attachment efficiency a values from 0.06 to 0.2 when the partial coverage of the glass beads surface increases from 0 to 25% (Lin et al., 2011).
The evaluation of the interactions efficiency of Nms with colloids present in surface waters has been evaluated through the calculation of the α values corresponding to homo-aggregation of Nms comparing to the heteroaggregation of Nms and colloids (Therezien et al., 2014). They compared the calculated data to the persistence of Ag0 in aquatic mesocosms built in the frame of the CEINT program (Lowry et al., 2012a). Heterogeneous aggregation should dominate the transport and fate of nanomaterials in aquatic media. The incorporation of transformation of Nms could be added to the model in order to predict the behavior of Nms. Similarly, Praetorius et al. (2012), using a database for the Rhine river, have calculated the transfer of uncoated TiO2 Nms through hetero-aggregation modeling using calculated values for the affinity, size, and fractal geometry of aggregates, settling velocity, etc. The moving of free TiO2 depends on the attachment efficiency and on different parameters as the number of colloids, their density, etc. If α is high, the longitudinal transfer in the river is short, and the concentration of TiO2 in the sediment increases quickly. It is the opposite if α is low.
An experimental and modeling approach has been recently developed (Praetorius et al., 2014) using colloids as SiO2 and natural organic matter and natural surface water (Rhône river) in contact with TiO2 nanomaterials. The aggregation of 100 mg/L of SiO2 in the presence of 0.8 mg/L of TiO2 is fast, due to a value of 0.9. The presence of NOM decreases the aggregation kinetic and α values. In the Rhône river, hetero-aggregation is fast, even for a TiO2 concentration of 0.1 mg/L, and characterized by an α value not far from 1.
From these results, it is obvious that the ecotoxicity of nanomaterials must be taken into account for predicting nanomaterial concentrations in surface waters and sediments in order to prioritize the organisms that can be exposed. The calculation of α in complex aquatic media constituted by various colloids (e.g., organics, clays, micro-biota, silica…) is a necessity to model the fate of the nanomaterials.
6 Evaluation of hazards taking into account the transformation of nanomaterials
6.1 Frame of hazard studies
To date, the majority of data concerns the effects of pristine nanomaterials on one type of organism. The large majority of these data are relative to bacteria and invertebrates. Generally the concentrations used for the toxicity tests are very large (generally > mg/L) as compared to the estimates (Gottschalk et al., 2009, 2010a,b), and the evaluation of the exposure in various contexts in complex environmental media is not really fulfilled.
The biomarkers used to evaluate the effects are associated with the defense mechanisms and damage of these organisms and allow evaluating the detoxification process (GST: anti-glutathione S-transferase (GST) antibody), oxidative defense (SOD: super oxide dismutase, CAT: catalase), hypoxia (lactase deshydrogenase), oxidation (TBARS: thiobarbituric acid reactive substances), neurotoxicity (AChE: acetylcholinesterase), apoptosis (CSP-3-like: caspase homologue) and genotoxicity (DNA damage). More recently, other parameters that relate the effects to the transformations of nanomaterials have been considered. They are the primary determinants of nanomaterials fate, transport, and toxicity (Auffan et al., 2008, 2009a,b). Transformations can be mediated by membrane cells that can alter the core nanomaterials as well as the surface functionality, the latter leading to possible changes in surface composition, aggregation state, reactivity, and potential toxicity (Auffan et al., 2010; Bottero and Wiesner, 2009; Lowry et al., 2012b).
The studies concerning the transformations of nanomaterials as CeO2 or Ag in relation with the effects are relatively recent. The properties that are exploited for various applications are also analyzed when CeO2 nanomaterials are in contact with bacteria or human fibroblasts (Auffan et al., 2009a; Thill et al., 2006; Zeyons et al., 2009). For CeO2 the transformation in contact with biota corresponds to a reduction of CeIV in CeIII as analyzed through XANES and is at the origin of a Fenton reaction (Benameur et al., 2014). Other metal oxide nanoparticles as TiO2, Fe0 or Fe3O4 have been shown to alter the antioxidant system (mainly by increasing CAT activity) of mollusks (Buffet et al., 2011), earthworms (Lapied et al., 2011) or daphnids (Wiench et al., 2009). In some cases, there is clear evidence of the production of ROSs (reactive oxygen species) directly by nanoparticles (e.g., photocatalytic properties of TiO2, or Fenton reactions due to the dissolution of Fe2+).
The mechanisms of transformation of Ag0 nanomaterials in the presence of micro-organisms, invertebrates and vertebrates were also analyzed through XAS in several studies (Levard et al., 2011, 2013a,b; Meyer et al., 2010; Reinsch et al., 2012; Yang et al., 2012; Yi et al., 2011). Most of these works have shown that the sulfidation of Ag0 through oxidation and dissolution in Ag+ reduces the toxicity against micro- and macro-organisms.
These studies constitute the basis of our knowledge on the toxic effect of nanomaterials, giving a first look on the mechanisms that can impact the oxidative status of organisms (micro, macro). The investigations of oxidative stress markers induced, directly of not, by exposed invertebrates seem to be a relevant proxy to evaluate the nanomaterials contamination level of organisms as well as their physiological status. For that, a thorough analysis of the speciation of nanomaterials in contact with biota is necessary at relevant environmental concentrations.
6.2 Necessity of studying the hazard of nanomaterials taking into account the exposure
One of the actual challenges in nano-ecotoxicology is the potential accumulation of nanomaterials by sediments that could impact organisms and transfer throughout food chains. This transfer can be ensured also through the life cycle of the organisms (e.g., molting of arthropods or maternal transfers), as shown with Daphnia pulex and Caenorbitis elegans (Auffan et al., 2013; Meyer et al., 2010). In order to evaluate more relevantly the relationship between transfer, transformation and biological effects, working in mesocosms with low concentrations of nanomaterials (from μg/L to 1 mg/L after about one month of chronic addition) and with an interdisciplinary approach (physical chemistry, (micro-) biology and ecology) is required. This has been achieved through the association between French and American researchers (through the CEINT and I-CEINT programs).
Mesocosms are the unique way to simulate ecosystems and to follow the evolution in terms of exposure and effects at various time and spatial scales. If some data exist using mesocosms (Buffet et al., 2012; Cleveland et al., 2012; Ferry et al., 2009), only few studies have taken into account all the parameters impacting biological effects. Recent data (Auffan et al., 2014; Lowry et al., 2012a; Tella et al., 2014) have shown the relationship between exposure (aggregation and sedimentation rates, nanomaterials concentration in sediments) and impacts. In the mesocosms, it is possible to inject nanomaterials through a single pulse or multiple pulses and to work with low concentrations of nanomaterials, even after one month (Auffan et al., 2014). All the biological assays necessary to evaluate the effects from genotoxicity to ecotoxicity and from the bacteria to macro-organisms can be used. According to the nanomaterials tested and the contamination scenarios, these experimental systems can operate with different physical and physicochemical features as water quality and depth, sediment mineralogy and depth, current velocity, tidal reservoirs, and biota, owing to a high flexibility.
Studying the transformation of nanomaterials in such complex systems and at low doses is also possible because nanomaterials are concentrated by surficial sediments, biofilms or specific organs of organisms. As an example, Tella et al. (2014) used aquatic indoor mesocosms to study the exposure and impacts of CeO2 nanomaterials. After injecting 1 mg/L of CeO2 nanomaterials, the concentration at the surface of the sediment reaches 100 mg/kg of Ce after one week and 500 mg/kg after one month. The concentration of Ce in the organisms also reaches 200 mg/kg (after one week) to 2000 mg/kg (dry weight) after one month in the digestive gland of adult snails Planorbarius corneus (Tella et al., 2014). Similarly, the reduction of CeIVO2 in CeIII in the organisms is important, reaching ∼80% of CeIII after 4 weeks in the adult's snails and respectively ∼40% and ∼20% in their juveniles and in their eggs. With the same approach and using outdoor mesocosms mimicking freshwater emergent wetlands, Lowry et al. (2012a) have evaluated the transfers, transformations and effects of Ag nanomaterials. The distribution of Ag has been evaluated from plants and organisms during 18 months. Silver sulfidation was demonstrated in the terrestrial soils and subaquatic sediments and a high body burden of Ag was measured in mosquito fish and chironomids. By simultaneously creating representative conditions for environmental transformation and ecosystem exposure, these studies in outdoor or indoor mesocosms allow the integration of the complementary biological and physical-chemical approaches into an environmental risk assessment model related to nanotechnologies based on reliable exposure and impact data.
Risk forecasting researches need the development of an harmonized terminology that supports accurate description of the properties of the nanomaterials and not only based on a size (M. Auffan et al., 2009a). It is also necessary to develop and standardize technics and protocols for their characterization in complex media (air, soils, sediments, wastes, surface water, biota, organisms…) and for evaluating the hazard correlating the exposure, transformation at low concentrations. This is the heart of the eco-conception of safer-by-design nanoproducts that is developed within the project Labex SERENADE (2012–2020), which gathers scientific organisms, universities and companies to promote sustainable and responsible development and competitiveness of nanotechnology, a new approach to entrepreneurship through the integration of findings on marketing, communication or ethics including key enabling technologies and nanosafety.
Acknowledgements
This manuscript is based partly on Works supported by the French ANR (Agence Nationale de la Recherche) through projects: Aging Nano (ANR-0CESA-001), Mesonnet (ANR-10-NANO-0006), European program NEPHH FP7 -228536, Labex SERENADE (ANR-11-LABX-0064 funded by the Government through “Investissement d’avenir” A*Midex project (No. ANR-11-IDEX-0001-02) and GDRI I-CEINT (CNRS-DUKE Univ).