The temporal and spatial evolution of dispersed media is a fundamental problem in a wide range of physicochemical systems, such as emulsions, suspensions and aerosols. These systems are multiphasic and involve compounds of different densities. They are therefore subject to the influence of gravity which determines the sedimentation rate of their dispersed phase. This effect can be dominant and prevent a detailed study of the phenomena occurring between the constituents themselves, such as the coalescence of drops in emulsions, the evaporation of droplets or the flocculation in suspensions. In this context, the Centre National d’Etudes Spatiales (CNES) has recently supported the development of a new instrument to produce populations of droplets, a few micrometers in radius, under controlled conditions with the objective of allowing a detailed study of their properties in microgravity conditions. The principle of this instrument is to generate, by a fast compression/expansion of air, populations of water droplets and to track their evolution by optical scanning tomography in transmission mode within a volume of approximately 2 mm
Révisé le :
Accepté le :
Première publication :
Publié le :
Charles Graziani 1 ; Mathieu Nespoulous 1 ; Renaud Denoyel 1 ; Stephan Fauve 2 ; Christian Chauveau 3 ; Luc Deike 4 ; Mickaël Antoni 1
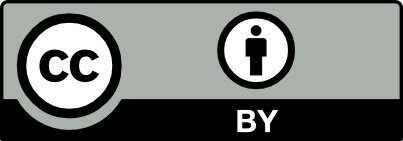
@article{CRMECA_2023__351_S2_183_0, author = {Charles Graziani and Mathieu Nespoulous and Renaud Denoyel and Stephan Fauve and Christian Chauveau and Luc Deike and Micka\"el Antoni}, title = {A new experimental set-up for aerosol stability investigations in microgravity conditions}, journal = {Comptes Rendus. M\'ecanique}, pages = {183--197}, publisher = {Acad\'emie des sciences, Paris}, volume = {351}, number = {S2}, year = {2023}, doi = {10.5802/crmeca.159}, language = {en}, }
TY - JOUR AU - Charles Graziani AU - Mathieu Nespoulous AU - Renaud Denoyel AU - Stephan Fauve AU - Christian Chauveau AU - Luc Deike AU - Mickaël Antoni TI - A new experimental set-up for aerosol stability investigations in microgravity conditions JO - Comptes Rendus. Mécanique PY - 2023 SP - 183 EP - 197 VL - 351 IS - S2 PB - Académie des sciences, Paris DO - 10.5802/crmeca.159 LA - en ID - CRMECA_2023__351_S2_183_0 ER -
%0 Journal Article %A Charles Graziani %A Mathieu Nespoulous %A Renaud Denoyel %A Stephan Fauve %A Christian Chauveau %A Luc Deike %A Mickaël Antoni %T A new experimental set-up for aerosol stability investigations in microgravity conditions %J Comptes Rendus. Mécanique %D 2023 %P 183-197 %V 351 %N S2 %I Académie des sciences, Paris %R 10.5802/crmeca.159 %G en %F CRMECA_2023__351_S2_183_0
Charles Graziani; Mathieu Nespoulous; Renaud Denoyel; Stephan Fauve; Christian Chauveau; Luc Deike; Mickaël Antoni. A new experimental set-up for aerosol stability investigations in microgravity conditions. Comptes Rendus. Mécanique, Physical Science in Microgravity within the Thematic Group Fundamental and Applied Microgravity, Volume 351 (2023) no. S2, pp. 183-197. doi : 10.5802/crmeca.159. https://comptes-rendus.academie-sciences.fr/mecanique/articles/10.5802/crmeca.159/
[1] The terminal velocity of fall for water droplets in stagnant air, J. Meteorol., Volume 6 (1949) no. 4, pp. 243-248 | DOI
[2] Empirical formulae for the terminal velocity of water drops falling through the atmosphere, Q. J. R. Meteorol. Soc., Volume 76 (1950) no. 329, pp. 302-311 | DOI
[3] et al. Measurement of the Raman spectra and hygroscopicity of four pharmaceutical aerosols as they travel from pressurised metered dose inhalers (pMDI) to a model lung, Int. J. Pharm., Volume 520 (2017) no. 1-2, pp. 59-69 | DOI
[4] Determination of parabens in cosmetic products using multi-walled carbon nanotubes as solid phase extraction sorbent and corona-charged aerosol detection system, J. Chromatogr. A., Volume 1217 (2010) no. 1, pp. 1-6 | DOI
[5] Enhancing the thermal and upper voltage performance of Ni-rich cathode material by a homogeneous and facile coating method: spray-drying coating with Nano-Al
[6] A paper-based electrochemical device for the detection of pesticides in aerosol phase inspired by nature: A flower-like origami biosensor for precision agriculture, Biosens. Bioelectron., Volume 205 (2022), 114119 | DOI
[7] Total aerosol effect: radiative forcing or radiative flux perturbation?, Atmos. Chem. Phys., Volume 10 (2010) no. 7, pp. 3235-3246 | DOI
[8] An emerging ground-based aerosol climatology: aerosol optical depth from AERONET, J. Geophys. Res., Volume 106 (2001) no. D11, pp. 12067-12097 | DOI
[9] Global aerosol climatology from the MODIS satellite sensors, J. Geophys. Res., Volume 113 (2008) no. D14, D14S07 | DOI
[10] The Physics of Blown Sand and Dust and Desert Dunes, William Morrow, New-York, 1942
[11] The terminal velocity of fall for water droplets in stagnant air, J. Meteorol., Volume 6 (1949), pp. 243-248 | DOI
[12] Homogeneous nucleation rates for water, J. Chem. Phys., Volume 99 (1993), pp. 4680-4692 | DOI
[13] A refined droplet approach to the problem of homogeneous nucleation from the vapor phase, J. Chem. Phys., Volume 94 (1991), pp. 3872-3884 | DOI
[14] Homogeneous nucleation rate measurements for water over a wide range of temperature and nucleation rate, J. Chem. Phys., Volume 78 (1983), pp. 3204-3211 | DOI
[15] Optical tomography in medical imaging, Inverse Probl., Volume 15 (1999) no. 2, p. R41-R93 | DOI | MR | Zbl
[16] Optical tomography: forward and inverse problems, Inverse Probl., Volume 25 (2009) no. 12, 123010 | DOI | MR | Zbl
[17] Learning approach to optical tomography, Optica, Volume 2 (2015) no. 6, pp. 517-522 | DOI
[18] Transition from spherical to irregular dispersed phase in water/oil emulsions, Langmuir, Volume 30 (2014) no. 16, pp. 4599-4604 | DOI
[19] Effect of SPAN80 on the structure of emulsified aqueous suspensions, Colloids Surf. A, Volume 521 (2017), pp. 121-132 | DOI
[20] Von Karman swirling flows, Annu. Rev. Fluid Mech., Volume 19 (1987) no. 1, pp. 465-491 | DOI | Zbl
[21] Direct observation of the intermittency of intense vorticity filaments in turbulence, Phys. Rev. Lett., Volume 67 (2007) no. 8, pp. 983-986 | DOI
[22] Study of the von Karman flow between coaxial corotating disks, Phys. Fluids, Volume 8 (1996), pp. 914-922 | DOI
[23] Thermodynamics of clouds, Bull. Am. Meteorol. Soc., Volume 46 (1965) no. 10, pp. 674-676
[24] The evaporation kinetics of pure water droplets at varying drying rates and the use of evaporation rates to infer the gas phase relative humidity, Phys. Chem. Chem. Phys., Volume 20 (2018), pp. 23453-23466 | DOI
[25] The importance of gravity in droplet evaporation: A comparison of pendant and sessile drop evaporation with particles, AIChE J., Volume 62 (2016), pp. 947-955 | DOI
[26] Sessile drop in microgravity: creation, contact angle and interface, Microgravity Sci. Technol., Volume 21 (2009), pp. 67-76 | DOI
[27] Experimental investigation of pendant and sessile drops in microgravity, Microgravity Sci. Technol., Volume 22 (2010), pp. 339-345 | DOI
[28] Microgravity Sci. Technol., 29 (2017), pp. 229-239 | DOI
Cité par Sources :
Commentaires - Politique