The impedance control of an pneumatic artificial muscle for isokinetic rehabilitation applications was investigated. Due to the direct contact of rehabilitation robots with the human body, the safety and reliability of these robots are of significant importance. For such applications, position and force must be introduced simultaneously. In this regard, there are two scenarios in which the controller should compromise between the position commands and the resultant force or vice versa. To achieve these goals and considering the safety requirements, a novel control algorithm was proposed, which was identified as a reliable strategy that can be utilized for rehabilitation purposes. The fuzzy sliding mode controller was implemented to control the actuator’s velocity (time-varying position signal) to eliminate/reduce chattering and improve the settling time. The performance of the controller using the position-based impedance algorithm was investigated through lab experiments. For experiments, a mechanism consisting of a pneumatic muscle and a pneumatic proportional valve was used, and the control of vertical reciprocating motion of a mass attached to the end of the muscle was investigated. Three different reference signals were used in tests, and the maximum tracking error was measured to be less than 6%. Using the measured error criteria, a parametric study was performed to identify the effects of three impedance parameters on the outputs. The results of the parametric study were reported through response surfaces and sensitivity charts. The presented methods have not been implemented in prior research for controlling a pneumatic muscle, and the results of the experiments were satisfactory.
Révisé le :
Accepté le :
Publié le :
Mahdi Chavoshian 1 ; Mostafa Taghizadeh 1 ; Nima Zamani Meymian 2
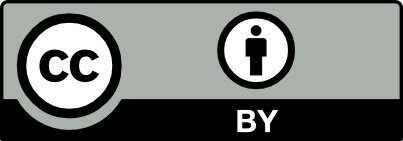
@article{CRMECA_2020__348_3_211_0, author = {Mahdi Chavoshian and Mostafa Taghizadeh and Nima Zamani Meymian}, title = {Implementation and experimental tests of an impedance control of pneumatic artificial muscles for isokinetic rehabilitation}, journal = {Comptes Rendus. M\'ecanique}, pages = {211--233}, publisher = {Acad\'emie des sciences, Paris}, volume = {348}, number = {3}, year = {2020}, doi = {10.5802/crmeca.16}, language = {en}, }
TY - JOUR AU - Mahdi Chavoshian AU - Mostafa Taghizadeh AU - Nima Zamani Meymian TI - Implementation and experimental tests of an impedance control of pneumatic artificial muscles for isokinetic rehabilitation JO - Comptes Rendus. Mécanique PY - 2020 SP - 211 EP - 233 VL - 348 IS - 3 PB - Académie des sciences, Paris DO - 10.5802/crmeca.16 LA - en ID - CRMECA_2020__348_3_211_0 ER -
%0 Journal Article %A Mahdi Chavoshian %A Mostafa Taghizadeh %A Nima Zamani Meymian %T Implementation and experimental tests of an impedance control of pneumatic artificial muscles for isokinetic rehabilitation %J Comptes Rendus. Mécanique %D 2020 %P 211-233 %V 348 %N 3 %I Académie des sciences, Paris %R 10.5802/crmeca.16 %G en %F CRMECA_2020__348_3_211_0
Mahdi Chavoshian; Mostafa Taghizadeh; Nima Zamani Meymian. Implementation and experimental tests of an impedance control of pneumatic artificial muscles for isokinetic rehabilitation. Comptes Rendus. Mécanique, Volume 348 (2020) no. 3, pp. 211-233. doi : 10.5802/crmeca.16. https://comptes-rendus.academie-sciences.fr/mecanique/articles/10.5802/crmeca.16/
[1]
(US Patent 5466213, November 1995)[2] Comparison of two techniques of robot-aided upper limb exercise training after stroke, Am. J. Phys. Med. Rehabil., Volume 83 (2004) no. 9, pp. 720-728 | DOI
[3] A high-performance redundantly actuated parallel mechanism for ankle rehabilitation, Int. J. Robot. Res., Volume 28 (2009) no. 9, pp. 1216-1227 | DOI
[4] Impedance control for a pneumatic robot-based around pole-placement joint space controllers, Control Eng. Pract., Volume 13 (2005), pp. 291-303 | DOI
[5] Pneumatic control of robots for rehabilitation, Int. J. Robot. Res., Volume 29 (2010) no. 1, pp. 23-38 | DOI
[6] Design of upper limb assistive device using a pneumatic cylinder, J. Rehabil. Robot., Volume 1 (2013) no. 1, pp. 9-18
[7] Pneumatically powered robotic exercise device to induce a specific force profile in target lower extremity muscles, Robotica, Volume 32 (2014) no. 8, pp. 1281-1299 | DOI
[8] Determining the static characteristics of pneumatic muscles, Meas. Control, Volume 49 (2016) no. 2, pp. 62-71 (Park YL) | DOI
[9] Bio-inspired active soft orthotic device for ankle foot pathologies, Intelligent Robots and Systems (IROS), 2011 IEEE/RSJ International Conference, IEEE, 2011, pp. 4488-4495
[10] A pneumatically powered knee-ankle-foot orthosis (KAFO) with myoelectric activation and inhibition, J. Neuroeng. Rehabil., Volume 6 (2009) no. 1, 23 | DOI
[11] Robust nonlinear control of an intrinsically compliant robotic gait training orthosis, IEEE Trans. Syst. Man Cybern. Syst., Volume 43 (2013) no. 3, pp. 655-665 | DOI
[12] Optimizing control of passive gait training exoskeleton driven by pneumatic muscles using switch-mode firefly algorithm, Robotica, Volume 37 (2009) no. 12, pp. 2087-2103 | DOI
[13] Design, analysis, and control of a cable-driven parallel platform with a pneumatic muscle active support, Robotica, Volume 35 (2017) no. 4, pp. 744-765 | DOI
[14] Control methods for exoskeleton rehabilitation robot driven with pneumatic muscles, Ind. Robot Int. J., Volume 36 (2009) no. 3, pp. 210-220 | DOI
[15] A system approach for control development of lower-limbs training machines, Control Eng. Pract., Volume 10 (2002) no. 3, pp. 287-299 | DOI
[16] Leg-robot for demonstration of spastic movements of brain-injured patients with compact magnetorheological fluid clutch, Adv. Robot., Volume 24 (2010) no. 5–6, pp. 671-686 | DOI
[17] Pneumatic muscle actuated isokinetic equipment for the rehabilitation of patients with disabilities of the bearing joints, Proceedings of the International MultiConference of Engineers and Computer Scientists (Lecture Notes in Engineering and Computer Science), Volume 2, The International Association of Engineers (IAENG), 2009 (ISSN: 2078-0966)
[18] Active knee rehabilitation orthotic device with variable damping characteristics implemented via an electrorheological fluid, IEEE/ASME Trans. Mechatronics, Volume 15 (2010) no. 6, pp. 952-960
[19] Pneumatic muscle actuator (PMA) task-specific resistance for potential use in microgravity exercise, Aviat. Space Environ. Med., Volume 83 (2012) no. 7, pp. 696-701 | DOI
[20] Pneumatic control of robots for rehabilitation, Int. J. Robot. Res., Volume 29 (2010) no. 1, pp. 23-38 | DOI
[21] Static and dynamic characteristics of McKibben pneumatic artificial muscles, Proceedings of the 1994 IEEE International Conference on Robotics and Automation, San Diego, CA, IEEE, 1994 | DOI
[22] The McKibben muscle and its use in actuating robot-arms showing similarities with human arm behavior, Ind. Robot Int. J., Volume 24 (1997) no. 6, pp. 432-439 | DOI
[23] The characteristics of the McKibben artificial muscle, The Application of External Power in Prosthetics and Orthotics, Nat. Acad. Sci.-Nat. Res. Council., Washington, DC, 1961
[24] Hill’s muscle model-based modeling of pneumatic artificial muscle, Ann. DAAAM Proc. (2011), pp. 1005-1007
[25] Piecewise affine modeling and constrained optimal control for a pneumatic artificial muscle, IEEE Trans. Ind. Electron., Volume 61 (2014) no. 2, pp. 904-916 | DOI
[26] Adaptive tracking control of an air powered robot actuator, Trans. ASME, J. Dyn. Syst. Meas. Control, Volume 115 (1993) no. 3, pp. 427-433 | DOI | Zbl
[27] Control of pneumatic muscle actuators, IEEE Control Syst., Volume 15 (1995) no. 1, pp. 40-48
[28] Tracking control of a pneumatic muscle actuator using one servo valve, American Control Conference (ACC), 2010, IEEE, 2010, pp. 4385-4390 | DOI
[29] Nonlinear model-based control of pneumatic artificial muscle servo systems, Control Eng. Pract., Volume 18 (2010) no. 3, pp. 311-317 | DOI
[30] Sliding mode tracking for pneumatic muscle actuators in opposing pair configuration, IEEE Trans. Control Syst. Technol., Volume 13 (2005) no. 4, pp. 550-558 | DOI
[31] Sliding-mode control of a high-speed linear axis driven by pneumatic muscle actuators, IEEE Trans. Ind. Electron., Volume 55 (2008) no. 11, pp. 3855-3864 | DOI
[32] Tracking control of pneumatic artificial muscle actuators based on sliding mode and non-linear disturbance observer, IET Control Theory Appl., Volume 4 (2010) no. 10, pp. 2058-2070 | DOI | MR
[33] MIMO sliding mode controller for gait exoskeleton driven by pneumatic muscles, IEEE Trans. Control Syst. Technol., Volume 26 (2017) no. 1, pp. 274-281 | DOI
[34] Trajectory tracking control of a pneumatic muscle system using fuzzy logic, Fuzzy Information Processing Society, 2005. NAFIPS 2005. Annual Meeting of the North American, IEEE, 2005, pp. 472-477 | DOI
[35] Fuzzy PD+I learning control for a pneumatic muscle, IEEE International Conference on Fuzzy Systems, IEEE, 2003
[36] Fuzzy neural network control of the rehabilitation robotic arm driven by pneumatic muscles, Ind. Robot Int. J., Volume 42 (2015) no. 1, pp. 36-43 | DOI
[37] An integrated intelligent nonlinear control method for a pneumatic artificial muscle, IEEE/ASME Trans. Mechatronics, Volume 21 (2016) no. 4, pp. 1835-1845
[38] Pneumatic artificial muscles: a switching model predictive control approach, Control Eng. Pract., Volume 21 (2013) no. 12, pp. 1653-1664 | DOI
[39] An echo state Gaussian process-based nonlinear model predictive control for pneumatic muscle actuators, IEEE Trans. Autom. Sci. Eng., Volume 16 (2018) no. 3, pp. 1071-1084 | DOI
[40] Actuation and control for robotic physiotherapy, Doctoral dissertation, University of Leeds (2001)
[41] The parallel approach to force/position control of robotic manipulators, IEEE Trans. Robot. Autom., Volume 9 (1993) no. 4, pp. 361-373 | DOI
[42] Hybrid position/force control of manipulators, Trans. ASME, J. Dyn. Syst. Meas. Control, Volume 103 (1981), pp. 126-133 | DOI
[43] Impedance control: an approach to manipulation: Part II—Implementation, Trans. ASME, J. Dyn. Syst. Meas. Control, Volume 107 (1985) no. 1, pp. 8-16 | DOI | Zbl
[44] Position-based impedance control of an industrial hydraulic manipulator, IEEE Control Syst., Volume 17 (1997) no. 1, pp. 46-52
[45] Advanced Sliding Mode Control for Mechanical Systems: Design, Analysis and MATLAB Simulation, Springer Science & Business Media, 2012 | Zbl
[46] Novel considerations on static force modeling of pneumatic muscle actuators, IEEE/ASME Trans. Mechatronics, Volume 21 (2016) no. 6, pp. 2647-2659 | DOI
[47] An optimization method for flexural bearing design for high-stroke high-frequency applications, Cryogenics, Volume 95 (2018), pp. 82-94 | DOI
Cité par Sources :
Commentaires - Politique