This article describes key issues which have to be addressed to apply Computational Fluid Dynamics to Naval Hydrodynamics. The specific aspects of Naval Hydrodynamics are discussed and illustrated by recent simulations and comparisons with available experiments. Free-surface flows with or without waves and even violent phenomena such as ventilation or cavitation can be modelled with mixture-fluid surface capturing. Turbulence modelling of thick boundary layers and vortical flows requires anisotropic RANS models or hybrid RANS/LES in case of strongly separated flows. Moreover, fluid–structure interaction in the form of rigid or flexible body motion and multi-body systems is crucial to represent ship manoeuvring and propulsion. Finally, the paper underlines the central role played by anisotropic adaptive grid refinement in the accurate simulation of marine flows.
Révisé le :
Accepté le :
Première publication :
Michel Visonneau 1 ; Ganbo Deng 1 ; Emmanuel Guilmineau 1 ; Alban Leroyer 1 ; Patrick Queutey 1 ; Jeroen Wackers 1
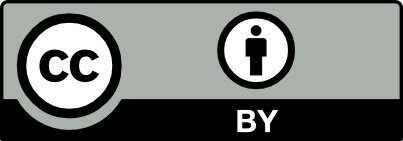
@article{CRMECA_2022__350_S1_A13_0, author = {Michel Visonneau and Ganbo Deng and Emmanuel Guilmineau and Alban Leroyer and Patrick Queutey and Jeroen Wackers}, title = {Computational fluid dynamics for naval hydrodynamics}, journal = {Comptes Rendus. M\'ecanique}, publisher = {Acad\'emie des sciences, Paris}, year = {2022}, doi = {10.5802/crmeca.162}, language = {en}, note = {Online first}, }
TY - JOUR AU - Michel Visonneau AU - Ganbo Deng AU - Emmanuel Guilmineau AU - Alban Leroyer AU - Patrick Queutey AU - Jeroen Wackers TI - Computational fluid dynamics for naval hydrodynamics JO - Comptes Rendus. Mécanique PY - 2022 PB - Académie des sciences, Paris N1 - Online first DO - 10.5802/crmeca.162 LA - en ID - CRMECA_2022__350_S1_A13_0 ER -
%0 Journal Article %A Michel Visonneau %A Ganbo Deng %A Emmanuel Guilmineau %A Alban Leroyer %A Patrick Queutey %A Jeroen Wackers %T Computational fluid dynamics for naval hydrodynamics %J Comptes Rendus. Mécanique %D 2022 %I Académie des sciences, Paris %Z Online first %R 10.5802/crmeca.162 %G en %F CRMECA_2022__350_S1_A13_0
Michel Visonneau; Ganbo Deng; Emmanuel Guilmineau; Alban Leroyer; Patrick Queutey; Jeroen Wackers. Computational fluid dynamics for naval hydrodynamics. Comptes Rendus. Mécanique, Online first (2022), pp. 1-19. doi : 10.5802/crmeca.162.
[1] Gothenburg 2010, A Workshop on Numerical Ship Hydrodynamics (L. Larsson; F. Stern; M. Visonneau, eds.), Chalmers University of Technology, Gothenburg, Sweden, 2010 | DOI
[2] Numerical Ship Hydrodynamics, An Assessment of the Tokyo 2015 Workshop, Springer International Publishing, 2020 | DOI
[3] Development of a structured overset Navier–Stokes solver with a moving grid and full multigrid method, J. Mar. Sci. Technol., Volume 24 (2019) no. 3, pp. 884-901 | DOI
[4] Accurate prediction of complex free surface flow around a high speed craft using a single-phase level set method, Comput. Mech., Volume 62 (2018) no. 3, pp. 421-437 | DOI | Zbl
[5] On the application of the one-phase level set method for naval hydrodynamic flows, Comput. Fluids, Volume 36 (2007) no. 5, pp. 868-886 | DOI | Zbl
[6] Ship hydrodynamics knowhow derived from computational tools—some examples, 9th Conference on Computational Methods in Marine Engineering (Marine 2021) (2022) | DOI
[7] Turn and zig-zag maneuvers of a surface combatant using URANS approach and dynamic overset grids, J. Mar. Sci. Technol., Volume 18 (2013) no. 2, pp. 166-181 | DOI
[8] Experimental and computational study of hull–propeller–rudder interaction for steady turning circles, Phys. Fluids, Volume 33 (2021), 127117 | DOI
[9] Viscous-flow calculations for KVLCC2 in maneuvering motion, J. Mar. Sci. Technol., Volume 18 (2013) no. 4, pp. 460-470
[10] Numerical simulation of the free-running of a ship using the propeller model and dynamic overset grid method, Ship Technol. Res., Volume 65 (2018) no. 3, pp. 153-162 | DOI
[11] False bottoms revisited: computational study for KCS under pure yaw motion in shallow water (H/T = 1.2), J. Mar. Sci. Technol. (2022), pp. 1-19 | DOI
[12] RANS investigation of influence of wave steepness on ship motions and added resistance in regular waves, J. Mar. Sci. Technol., Volume 23 (2018) no. 4, pp. 991-1003 | DOI
[13] Parametric study of added resistance and ship motion in head waves through RANS: calculation guideline, Appl. Ocean Res., Volume 110 (2021), 102573 | DOI
[14] Assessment of EFD and CFD capability for KRISO container ship added power in head and oblique waves, Ocean Eng., Volume 243 (2022), 110224 | DOI
[15] Propeller–hull interaction beyond the propulsive factors—a case study on the performance of different propeller designs, Ocean Eng., Volume 256 (2022), 111424 | DOI
[16] Analysis of the thrust deduction in waterjet propulsion—the Froude number dependence, Ocean Eng., Volume 152 (2018), pp. 100-112 | DOI
[17] Implicit LES predictions of the cavitating flow on a propeller, J. Fluids Eng., Volume 132 (2010) no. 4, 041302
[18] Large eddy simulations of cavitating tip vortex flows, Ocean Eng., Volume 195 (2020), 106703 | DOI
[19] Modification of k- turbulence model for ship resistance flow predictions, J. Ocean Eng. Mar. Energy, Volume 8 (2022) no. 4, pp. 527-538 | DOI
[20] DES for a tanker at drift angles with analogy to delta wings, Ocean Eng., Volume 55 (2012), pp. 23-43
[21] An overset RaNS prediction and validation of full scale stern wake for 1600 TEU container ship and 63,000 DWT bulk carrier with an energy saving device, Appl. Ocean Res., Volume 105 (2020), 102417 | DOI
[22] Submarine hydrodynamics for off-design conditions, J. Ocean Eng. Mar. Energy, Volume 8 (2022) no. 4, pp. 499-511 | DOI
[23] Assessment of CFD for surface combatant 5415 at straight ahead and static drift = 20, ASME J. Fluids Eng., Volume 141 (2019), pp. 1-26 | DOI
[24] Detached eddy simulations and tomographic PIV measurements of flows over surface combatant 5415 at straight-ahead and static drift conditions, Ocean Eng., Volume 238 (2021), 109658 | DOI
[25] Current capabilities of DES and LES for submarines at straight course, J. Ship Res., Volume 54 (2010) no. 03, pp. 184-196 | DOI
[26] Review and comparison of methods to model ship hull roughness, Appl. Ocean Res., Volume 99 (2020), 102119 | DOI
[27] Numerical study of roughness model effect including low-Reynolds number model and wall function method at actual ship scale, J. Mar. Sci. Technol., Volume 26 (2021) no. 1, pp. 24-36 | DOI
[28] Towing tank PIV measurement system, data and uncertainty assessment for DTMB model 5512, Exp. Fluids, Volume 31 (2001) no. 3, pp. 336-346 | DOI
[29] Phase-averaged PIV for the nominal wake of a surface ship in regular head waves, Int. J. Numer. Meth. Fluids, Volume 53 (2007) no. 2, pp. 229-256
[30] Experimental data for JBC resistance, sinkage, trim, self-propulsion factors, longitudinal wave cut and detailed flow with and without an energy saving circular duct, Numerical Ship Hydrodynamics: An Assessment of the Tokyo 2015 Workshop (T. Hino; F. Stern; L. Larsson; M. Visonneau; N. Hirata; J. Kim, eds.), Springer, Cham, 2021, pp. 23-51 | DOI
[31] Experimental and numerical studies of the flow around the JBC hull form at straight ahead condition and 8 drift angle, The 33rd Symposium on Naval Hydrodynamics (33rd SNH) (2020)
[32] Experimental investigation on the wake of the Japan bulk carrier model with stereoscopic and tomographic particle image velocimetry, The 34th Symposium on Naval Hydrodynamics (34th SNH) (2022)
[33] Numerical predictions of two fluid systems with sharp interfaces, Ph. D. Thesis, Imperial College of Science, Technology & Medicine, University of London (1997)
[34] An interface capturing method for free-surface hydrodynamic flows, Comput. Fluids, Volume 36 (2007) no. 9, pp. 1481-1510 | DOI | Zbl
[35] The ULTIMATE conservative difference scheme applied to unsteady one-dimensional advection, Comput. Meth. Appl. Mech. Eng., Volume 88 (1991) no. 1, pp. 17-74 | DOI | Zbl
[36] Free-surface viscous flow solution methods for naval hydrodynamic, Arch. Comput. Meth. Eng., Volume 18 (2011) no. 1, pp. 1-41 | DOI | Zbl
[37] Curvature-compensated convective transport: SMART, a new boundedness-preserving transport algorithm, Int. J. Numer. Meth. Fluids, Volume 8 (1988), pp. 617-641 | DOI | Zbl
[38] Large area propellers, Second International Symposium on Marine Propulsors, smp’11, Hamburg, Germany (2011)
[39] Assessing bi-stable ventilation for surface-piercing hydrofoils through numerical simulation, Innov’Sail 2020, Goteborg, Sweden (2020) (online)
[40] Experimental and numerical investigation of ventilation inception and washout mechanisms of a surface-piercing hydrofoil, 30th ONR Symposium on Naval Hydrodynamics, Hobart, Tasmania (2014)
[41] Numerical simulations using momentum source wave-maker applied to RANS equation model, Coast. Eng., Volume 56 (2009), pp. 1043-1060 | DOI
[42] Comparison of wave modeling methods in CFD solvers for ocean engineering applications, Ocean Eng., Volume 188 (2019), 106237
[43] The numerical solution of steady water wave problems, Comput. Geosci., Volume 14 (1988) no. 3, pp. 357-368 | DOI
[44] Numerical methods for nonlinear waves, Coast. Ocean Eng., Volume 14 (1999), pp. 357-368
[45] Analytical prediction of reflection coefficients for wave absorbing layers in flow simulations of regular free-surface waves, Ocean Eng., Volume 147 (2018), pp. 132-147 | DOI
[46] Analytical estimate of the reflection coefficient for forcing zones in 3D-flow simulations with nonlinear free-surface waves, 33rd Symposium on Naval Hydrodynamics, Osaka, Japan (2020)
[47] A preconditioned Navier–Stokes method for two-phase flows with application to cavitation prediction, Comput. Fluids, Volume 29 (2000) no. 8, pp. 849-875 | DOI | Zbl
[48] A two-phase flow model for predicting cavitation dynamics, ICMF 2004 International Conference on Multiphase Flow, Yokohama, Japan (2004)
[49] Development of a new cavitation model based on bubble dynamics, ZAMM—J. Appl. Math. Mech., Volume 81 (2001) no. S3, pp. 561-562 | DOI | Zbl
[50] Comparison of mass transfer models for the numerical prediction of sheet cavitation around a hydrofoil, Int. J. Multiphase Flow, Volume 37 (2011) no. 6, pp. 620-626 | DOI
[51] The influence of nucleation on cavitation inception in tip-leakage flows, 34th Symposium on Naval Hydrodynamics (2022)
[52] Two phase flow structure of cavitation: experiment and modeling of unsteady effects, 3rd International Symposium on Cavitation CAV1998, Grenoble, France, Volume 26 (1998), pp. 1-8
[53] Flow measurement around a 300 K VLCC model, Proceedings of the Annual Spring Meeting, SNAK, Ulsan (1998), pp. 185-188
[54] Comparison of explicit algebraic stress models and second-order turbulence closures for steady flows around ships, 7th International Conference on Numerical Ship Hydrodynamics (1999)
[55] Differential Reynolds-stress modeling for aeronautics, AIAA J., Volume 53 (2015) no. 3, pp. 1-17 (Published online: 10 September 2014, March 2015)
[56] Comments on the feasibility of LES for wings and on a hybrid RANS/LES approach, 1st AFOSR International Conference on DNS/LES (C. Z. Liu, ed.) (Advances in DNS/LES), Greyden Press, 1997, pp. 137-147
[57] Chapter four - Hybrid RANS-LES methods, Advanced Approaches in Turbulence (P. Durbin, ed.), Elsevier, 2021, pp. 133-159 | DOI
[58] Development of DDES and IDDES formulations for the k- shear stress transport model, Flow, Turbul. Combust., Volume 88 (2012), pp. 431-449 | DOI | Zbl
[59] Adaptive grid refinement for hydrodynamic flows, Comput. Fluids, Volume 55 (2012), pp. 85-100 | DOI | Zbl
[60] Creation of internal points in Voronoi’s type method. Control adaptation, Adv. Eng. Software, Volume 13 (1991) no. 5/6, pp. 303-312 | Zbl
[61] High-order sonic boom modeling based on adaptive methods, J. Comput. Phys., Volume 229 (2010) no. 3, pp. 561-593 | DOI | Zbl
[62] Average-based mesh adaptation for hybrid RANS/LES simulation of complex flows, Comput. Fluids, Volume 232 (2022), 105202 | DOI | Zbl
[63] Added mass evaluation with a finite-volume solver for applications in fluid–structure interaction problems solved with co-simulation, J. Fluids Struct., Volume 81 (2018), pp. 528-546 | DOI
[64] FSI investigation on stability of downwind sails with an automatic dynamic trimming, Ocean Eng., Volume 90 (2014), pp. 129-139 | DOI
[65] Acceleration of a fixed point algorithm for fluid–structure interaction using transpiration conditions, ESAIM: Math. Model. Numer. Anal., Volume 37 (2003) no. 04, pp. 601-616 (transpiration condition) | DOI | Numdam | Zbl
[66] ONR tumblehome course keeping and maneuvering in calm water and waves, J. Mar. Sci. Technol., Volume 24 (2019), pp. 948-967 | DOI
[67] Dynamic behaviour of the loads of podded propellers in waves: experimental and numerical simulations, International Conference on Offshore Mechanics and Arctic Engineering, Volume 8A: Ocean Engineering (2014)
[68] Numerical methods for RANSE simulations of a self-propelled fish-like body, J. Fluids Struct., Volume 20 (2005) no. 7, pp. 975-991 | DOI
[69] Validation of CFD simulations of the flow around a full-scale rowing blade with realistic kinematics, J. Mar. Sci. Technol., Volume 24 (2018) no. 4, pp. 1105-1118 | DOI
Cité par Sources :
Commentaires - Politique