[Systèmes implantables sans contact. Analyse de l’efficacité du transfert par le formalisme de la matrice de diffusion]
En considérant un système implantable sans contact comme une paire de composants Tx–Rx (émetteur-récepteur), l’article montre que l’efficacité de transfert peut être calculée en vue d’obtenir un résultat optimal pouvant être évalué au moyen de mesures ou de résultats issus de la littérature. À cette fin, le formalisme des paramètres S (matrice de diffusion) est introduit à la place des quantités généralement utilisées (gain, pertes, diagramme de rayonnement). En considérant le système comme une boîte noire, des remarques sur le calcul de la liaison optimale sont formulées selon la fréquence, la taille de l’antenne, l’utilisation d’un fantôme, l’intégration de circuits d’adaptation et d’amplificateurs d’efficacité. Les normes internationales relatives à la puissance autorisée et aux niveaux de sécurité à respecter sont inclues dans la discussion. Différents scénarios incluant la télémétrie, la récupération d’énergie sans fil et la transmission d’informations par des capteurs sont présentés à titre d’exemples.
Looking at wireless implantable systems as a pair of or more Tx–Rx components, we discuss how their efficiency can be calculated in order to achieve an optimum result that can be evaluated over measurements and literature. To that end, scattering parameters’ notation is introduced instead of considerations of the gain, the propagation losses and the radiation patterns. Looking at the system as a black box, remarks on optimum link calculation in relation to frequency, antenna size, phantom use, matching circuit integration and efficiency intensifiers are being carried out. International standards for allowed power and safety levels are added to the discussion. Different scenarios including telemetry, wireless harvesting and sensor transmission information are included as examples.
Révisé le :
Accepté le :
Première publication :
Publié le :
Mots-clés : Paramètres S, Equation de Friis, Bilan de liaison, DAS (débit d’absorption spécifique), Récupération d’énergie sans fil
Stavros Koulouridis 1
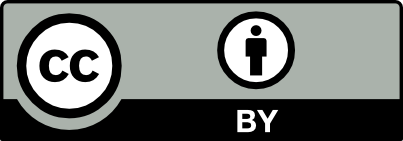
@article{CRPHYS_2024__25_S1_5_0, author = {Stavros Koulouridis}, title = {Implantable {T\protect\textsubscript{x}{\textendash}R\protect\textsubscript{x}} wireless systems. {An} overview of their efficiency analysis through scattering matrix formalism}, journal = {Comptes Rendus. Physique}, pages = {5--22}, publisher = {Acad\'emie des sciences, Paris}, volume = {25}, number = {S1}, year = {2024}, doi = {10.5802/crphys.208}, language = {en}, }
TY - JOUR AU - Stavros Koulouridis TI - Implantable Tx–Rx wireless systems. An overview of their efficiency analysis through scattering matrix formalism JO - Comptes Rendus. Physique PY - 2024 SP - 5 EP - 22 VL - 25 IS - S1 PB - Académie des sciences, Paris DO - 10.5802/crphys.208 LA - en ID - CRPHYS_2024__25_S1_5_0 ER -
Stavros Koulouridis. Implantable Tx–Rx wireless systems. An overview of their efficiency analysis through scattering matrix formalism. Comptes Rendus. Physique, Energy in the heart of EM waves: modelling, measurements and management, Volume 25 (2024) no. S1, pp. 5-22. doi : 10.5802/crphys.208. https://comptes-rendus.academie-sciences.fr/physique/articles/10.5802/crphys.208/
[1] A novel dual-band implantable antenna for pancreas telemetry sensor applications, Telecom, Volume 3 (2022) no. 1, pp. 1-16 | DOI | Zbl
[2] Radiowave propagation from a tissue-implanted source at 418 MHz and 916.5 MHz, IEEE Trans. Biomed. Eng., Volume 47 (2000) no. 4, pp. 527-534 | DOI
[3] Design of a dual-band implantable antenna and development of skin mimicking gels for continuous glucose monitoring, IEEE Trans. Microw. Theory Tech., Volume 56 (2008), pp. 1001-1008 | DOI
[4] A dual-band implantable rectenna for wireless data and power support at sub-GHz region, IEEE Trans. Antennas Propag., Volume 67 (2019) no. 11, pp. 6800-6810 | DOI
[5] Implantable wireless transmission rectenna system for biomedical wireless applications, IEEE Access, Volume 8 (2020), pp. 195551-195558 | DOI
[6] Optimal radiation of body-implanted capsules, Phys. Rev. Lett., Volume 122 (2019) no. 10, 108101 | DOI
[7] Microwave Engineering, Wiley, Hoboken, NJ, 2011
[8] et al. Experimental ultra wideband path loss models for implant communications, 2016 IEEE 27th Annual International Symposium on Personal, Indoor, and Mobile Radio Communications (PIMRC), Valencia, Spain, 2016, pp. 1-6
[9] Characterization of implantable antennas for intracranial pressure monitoring: reflection by and transmission through a scalp phantom, IEEE Trans. Microw. Theory Tech., Volume 56 (2008) no. 10, pp. 2366-2376 | DOI
[10] Antenna characterization for wireless power-transmission system using near-field coupling, IEEE Antennas Propag. Mag., Volume 54 (2012) no. 4, pp. 108-116 | DOI
[11] Capsule antenna design based on transmission factor through the human body, IEICE Trans. Commun., Volume 101 (2018) no. 2, pp. 357-363 | DOI
[12] Performances of an implanted cavity slot antenna embedded in the human arm, IEEE Trans. Antennas Propag., Volume 57 (2009) no. 4, pp. 894-899 | DOI
[13] et al. A compact dual-band implantable antenna for wireless biotelemetry in arteriovenous grafts, IEEE Trans. Antennas Propag., Volume 71 (2023) no. 6, pp. 4759-4771 | DOI
[14] Medical Device Radiocommunications Service (MedRadio) (Federal Communications Commission. [Available in: https://www.fcc.gov/medical-device-radiocommunications-service-medradio])
[15] Ultra Low Power Medical Data Service (MEDS) Systems operating in the frequency range 401 MHz to 402 MHz and 405 MHz to 406 MHz; Harmonised Standard covering the essential requirements of article 3.2 of the Directive 2014/53/EU, 2016 (European Telecommunications Standards Institute)
[16] Ultra Low Power Active Medical Implants (ULP-AMI) and associated Peripherals (ULP-AMI-P) operating in the frequency range 402 MHz to 405 MHz; Harmonised Standard covering the essential requirements of article 3.2 of the Directive 2014/53/EU, 2016 (European Telecommunications Standards Institute)
[17] Wireless Medical Telemetry Service, 47 CFR Parts 1, 2, 15, 90 and 95, Federal Communications Commission, Federal Register (The Daily Journal of United States Government), Volume 65 (2000) no. 137, pp. 43995-44010
[18] Short Range Devices (SRD) operating in the frequency range 25 MHz to 1,000 MHz with power levels ranging up to 500 mW e.r.p ([Available in https://www.etsi.org/committee/1398-erm])
[19] Part 15. RadioFrequency Devices ([Available in https://www.ecfr.gov/current/title-47/chapter-I/subchapter-A/part-15])
[20] Guidelines for limiting exposure to, electromagnetic fields (100 KHz to 300 GHz), 2020 (ICNIRP Guidelines)
[21] IEEE standard for safety levels with respect to human exposure to electric, magnetic, and electromagnetic fields, 0 Hz to 300 GHz - Corrigenda 2, 2019–2020
[22] Characterization of an intra-body wireless link in the UHF band, Prog. Electromagn. Res. M, Volume 111 (2022), pp. 247-259 | DOI
[23] Intra-body propagation channel investigation using electrically coupled loop antenna, Prog. Electromagn. Res. M, Volume 40 (2014), pp. 57-67 | DOI
[24] Effect of tissue boundaries on the intra-body communication channel at 2.38 GHz, 2017 International Workshop on Antenna Technology: Small Antennas, Innovative Structures, and Applications (iWAT), 2017, pp. 285-288
[25] Design of implantable microstrip antennas for communication with medical implants, IEEE Trans. Microw. Theory Tech., Volume 52 (2004) no. 8, pp. 1944-1951 | DOI
[26] Compact broadband stacked implantable antenna for biotelemetry with medical devices, Electron Lett., Volume 43 (2007), pp. 660-662 | DOI
[27] Performance of a novel miniature antenna implanted in the human head for wireless biotelemetry, IEEE AP-S International Symposium on Antennas and Propagation and USNC/URSI, Spokane, Washington, USA, 2011
[28] Metasurface patch for wireless power transfer in implantable devices, Adv. Funct. Mater., Volume 33 (2023) no. 38, 2300027
[29] et al. The use of metasurfaces to enhance microwave imaging: experimental validation for tomographic and radar-based algorithms, IEEE Open J. Antennas Propag., Volume 3 (2022), pp. 89-100 | DOI
Cité par Sources :
Commentaires - Politique
Vous devez vous connecter pour continuer.
S'authentifier