[Quantifier l’érosion de surface des sols]
Cet article de synthèse introduit tout d’abord le contexte général de l’érosion des sols, un processus naturel omniprésent impliquant des échelles spatio-temporelles extrèmement variées. Si cette perte de matière solide induite par toutes sortes d’écoulements fluides à la surface des sols est souvent source de beauté des paysages qui nous entourent, elle n’en constitue pas moins un risque majeur et fait peser des menaces croissantes dans le contexte du changement global comme la perte de fertilité des sols agricoles, le déclin des écosystèmes littoraux ou le besoin accru de sureté des ouvrages de protection contre les inondations. Par conséquent, il est essentiel de pouvoir prédire aux différentes échelles les niveaux futurs de retrait des sols afin de mettre en oeuvre de possibles mesures d’anticipation ou d’atténuation. Il convient pour cela de connaître la sensibilité à l’érosion d’un sol via une quantification expérimentale. Pour décrire plus en détail ce type de mesures, le choix est fait ici de restreindre le cadre considéré aux situations d’érosion de surface concentrée de sols homogènes, sur des échelles spatiales et temporelles restant modérées, et telle que typiquement étudiée dans les domaines de l’ingénierie civile ou environnementale. La mesure de l’érosion en elle-même est délicate et complexe, aussi bien pour déterminer le taux de matière érodée à une surface que pour sélectionner et quantifier une grandeur hydrodynamique pertinente, capable de rendre compte de la force exercée par l’écoulement. Un cadre conceptuel est ensuite nécessaire pour définir correctement une érodabilité du sol, condidérée comme une propriété intrinsèque du matériau à travers une loi empirique d’érosion. Dans l’approche la plus communément acceptée, cette érodibilité regroupe deux paramètres distincts : un seuil d’initiation de l’érosion, généralement choisi comme une contrainte de cisaillement critique, et un coefficient cinétique. Les différents types d’érodimètres issus de la litérature sont ensuite présentés et comparés, avec un descriptif spécifique et plus complet sur les trois principaux dispositifs utilisés en géomécanique (EFA, HET et JET). Enfin, avant de conclure et de suggérer quelques perspectives futures sur la thématique, nous présentons les nombreux avantages et utilisations possibles des valeurs d’érodabilité issues de ces essais, tout en montrant les limites de l’approche et les questions que celles-ci soulèvent.
This review first introduces the general context of soil erosion, an omnipresent natural process involving a wide variety of spatial and temporal scales. While this loss of solid matter induced by all kinds of fluid flows on the surface of soils is often a source of beauty in the landscapes that surround us, it also constitutes a major risk and poses growing threats in the context of global change as loss of agricultural soil fertility, decline in coastal ecosystems or increase in safety standards for flood protection structures. It is therefore essential to be able to predict future soil removal rates at multiple scales, in order to anticipate or mitigate their impacts. This requires experimental quantification of soil’s vulnerability to erosion. In order to describe this type of measurement in deeper detail, we restricted the scope of our review to situations of concentrated surface erosion of homogeneous soils, on moderate spatial and temporal scales, as typically studied in the fields of civil or environmental engineering. Measuring erosion itself is challenging, both in terms of determining the rate of mass removal from a surface, and in terms of selecting and quantifying a relevant hydrodynamic quantity to reflect the strength exerted by the flow. A conceptual framework is then required to correctly define soil’s erodibility, which is defined as an intrinsic property of the material through an empirical erosion law. In the most commonly accepted approach, this erodibility combines two distinct parameters: an erosion initiation threshold, generally chosen as a critical shear stress, and an erosion kinetic coefficient. The various types of erodimeters found in literature are then presented and compared, with a specific and more complete description of the three main devices used in geomechanics (EFA, HET and JET). Finally, before concluding and suggesting some perspectives on the topic, we outline the various advantages and applications of the erodibility values derived from experimental tests, while showing the limitations of the approach and the questions raised by them.
Révisé le :
Accepté le :
Première publication :
Mots-clés : Erosion, Mesure, Erodabilité des sols
Pierre Philippe 1 ; Nadia Benahmed 1
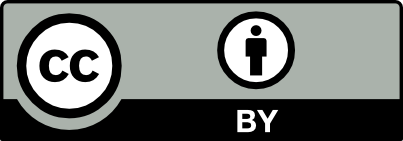
@article{CRPHYS_2024__25_S3_A20_0, author = {Pierre Philippe and Nadia Benahmed}, title = {Quantifying soil surface erosion}, journal = {Comptes Rendus. Physique}, publisher = {Acad\'emie des sciences, Paris}, year = {2024}, doi = {10.5802/crphys.225}, language = {en}, note = {Online first}, }
Pierre Philippe; Nadia Benahmed. Quantifying soil surface erosion. Comptes Rendus. Physique, Online first (2024), pp. 1-36. doi : 10.5802/crphys.225.
[1] Global silicate weathering and CO2 consumption rates deduced from the chemistry of large rivers, Chem. Geol., Volume 159 (1999) no. 1, pp. 3-30 | DOI
[2] Weathering indices as climate proxies. A step forward based on Congo and SW African river muds, Earth-Sci. Rev., Volume 201 (2020), 103039 | DOI
[3] Biomorphodynamics: Physical-biological feedbacks that shape landscapes, Water Resour. Res., Volume 44 (2008), W11301 | DOI
[4] A review of erosion and sediment transport models, Environ. Model. Softw., Volume 18 (2003), pp. 761-799 | DOI
[5] A review of hillslope and watershed scale erosion and sediment transport models, CATENA, Volume 64 (2005) no. 2, pp. 247-271 (25 Years of Assessment of Erosion) | DOI
[6] Soil erosion in the Anthropocene: research needs, Earth Surf. Process. Landf., Volume 43 (2018) no. 1, pp. 64-84 | DOI
[7] Principles of Sediment Transport in Rivers, Estuaries and Coastal Seas, 1006, Aqua Publications, Amsterdam, 1993
[8] Dynamics of Marine Sands, Thomas Telford Publishing, 1997
[9] The physics of sediment transport initiation, cessation, and entrainment across aeolian and fluvial environments, Rev. Geophys., Volume 58 (2020) no. 1, e2019RG000679 | DOI
[10] Physics and Modelling of Wind Erosion, Atmospheric and Oceanographic Sciences Library, 37, Springer, 2009, pp. 1-452 | DOI
[11] Global long-term observations of coastal erosion and accretion, Sci. Rep., Volume 8 (2018) no. 1, 12876 | DOI
[12] The management of coastal erosion, Ocean Coast. Manag., Volume 156 (2018), pp. 4-20 | DOI
[13] Soil erodibility and processes of water erosion on hillslope, Geomorphology, Volume 32 (2000) no. 3, pp. 385-415 | DOI
[14] A century of gully erosion research: urgency, complexity and study approaches, Earth-Sci. Rev., Volume 160 (2016), pp. 300-319 | DOI
[15] Impact of plant roots on the resistance of soils to erosion by water: a review, Prog. Phys. Geo.: Earth Environ., Volume 29 (2005) no. 2, pp. 189-217 | DOI
[16] Soil-erosion and runoff prevention by plant covers: a review, Sustainable Agriculture (E. Lichtfouse; M. Navarrete; P. Debaeke; S. Véronique; C. Alberola, eds.), Volume 2009, Springer, Netherlands, pp. 785-811 | DOI
[17] Subsurface erosion by soil piping: significance and research needs, Earth-Sci. Rev., Volume 185 (2018), pp. 1107-1128 | DOI
[18] Sediment detachment and transport processes associated with internal erosion of soil pipes, Earth Surf. Process. Landf., Volume 43 (2018) no. 1, pp. 45-63 | DOI
[19] The measurement of river bank erosion and lateral channel change: A review, Earth Surf. Process. Landf., Volume 18 (1993) no. 9, pp. 777-821 | DOI
[20] Hydraulic erosion of cohesive riverbanks, Geomorphology, Volume 76 (2006) no. 1, pp. 193-206 | DOI
[21] A review of bridge scour: mechanism, estimation, monitoring and countermeasures, Nat. Hazards, Volume 87 (2017), pp. 1881-1906 | DOI
[22] Erosion mitigation of bridge abutment backfill during flood events, J. Perform. Constr. Facil., Volume 37 (2023) no. 6, 04023054 | DOI
[23] The statistics of embankment dam failures and accidents, Can. Geotech. J., Volume 37 (2000) no. 5, pp. 1000-1024 | DOI
[24] Critical appraisal of piping phenomena in earth dams, Bull. Eng. Geol. Environ., Volume 66 (2007), pp. 381-402 | DOI
[25] Assessing the potential of internal instability and suffusion in embankment dams and their foundations, J. Geotech. Geoenviron. Eng., Volume 134 (2008) no. 3, pp. 401-407 | DOI
[26] Erosion in Geomechanics Applied to Dams and Levees, ISTE Ltd and John Wiley & Sons Inc, London, Hoboken, 2013 | DOI
[27] Introduction to Geomorphology, Hodder Arnold Publication, Arnold, 1998
[28] Countries and the global rate of soil erosion, Nat. Sustain., Volume 3 (2020), pp. 51-55 | DOI
[29] Soil erosion and agricultural sustainability, Proc. Natl. Acad. Sci. USA, Volume 104 (2007) no. 33, pp. 13268-13272 | DOI
[30] Socio-economic factors in soil erosion and conservation, Environ. Sci. Policy, Volume 6 (2003) no. 1, pp. 1-6 | DOI
[31] Impacts of climate change on water erosion: a review, Earth-Sci. Rev., Volume 163 (2016), pp. 94-117 | DOI
[32] et al. An assessment of the global impact of 21st century land use change on soil erosion, Nat. Commun., Volume 8 (2017) no. 1, 2013 | DOI
[33] Land use and climate change impacts on global soil erosion by water (2015–2070), Proc. Natl. Acad. Sci. USA, Volume 117 (2020) no. 36, pp. 21994-22001 | DOI
[34] Does soil thinning change soil erodibility? An exploration of long-term erosion feedback systems, SOIL, Volume 9 (2023) no. 1, pp. 71-88 | DOI
[35] An overview of soil loss tolerance, Catena, Volume 78 (2009) no. 2, pp. 93-99 | DOI
[36] et al. The impact of agricultural soil erosion on the global carbon cycle, Science, Volume 318 (2007) no. 5850, pp. 626-629 | DOI
[37] Agricultural soil erosion and global carbon cycle: controversy over?, Earth Surf. Process. Landf., Volume 34 (2009) no. 7, pp. 1033-1038 | DOI
[38] Erosion, deposition and soil carbon: A review of process-level controls, experimental tools and models to address C cycling in dynamic landscapes, Earth-Sci. Rev., Volume 154 (2016), pp. 102-122 | DOI
[39] Radiocesium transfer from hillslopes to the Pacific Ocean after the Fukushima Nuclear Power Plant accident: a review, J. Environ. Radioact., Volume 148 (2015), pp. 92-110 | DOI
[40] Development of a hybrid pollution index for heavy metals in marine and estuarine sediments, Environ. Monit. Assess., Volume 187 (2015), 306 | DOI
[41] Pollution indices as useful tools for the comprehensive evaluation of the degree of soil contamination—A review, Environ. Geochem. Health, Volume 40 (2018), pp. 2395-2420 | DOI
[42] River plastic emissions to the world’s oceans, Nat. Commun., Volume 8 (2017), 15611 | DOI
[43] Transport of microplastics in coastal seas, Estuar. Coast. Shelf Sci., Volume 199 (2017), pp. 74-86 | DOI
[44] Anthropogenic climate change has driven over 5 million km of drylands towards desertification, Nat. Commun., Volume 11 (2020), 3853 | DOI
[45] Global warming and coastal erosion, Clim. Change, Volume 64 (2004), pp. 41-58 | DOI
[46] Coastal erosion and climate change: A review on coastal-change process and modeling, Ambio, Volume 52 (2023) no. 12, pp. 2034-2052 | DOI
[47] Bridge pier scour: A review of processes, measurements and estimates, Environ. Eng. Manag. J., Volume 11 (2012), pp. 975-989 | DOI
[48] Long-term landscape evolution: linking tectonics and surface processes, Earth Surf. Process. Landf., Volume 32 (2007) no. 3, pp. 329-365 | DOI
[49] Physically based soil erosion and sediment yield models revisited, CATENA, Volume 147 (2016), pp. 595-620 | DOI
[50] et al. Soil erosion modelling: A global review and statistical analysis, Sci. Total Environ., Volume 780 (2021), 146494 | DOI
[51] et al. The European Soil Erosion Model (EUROSEM): a dynamic approach for predicting sediment transport from fields and small catchments, Earth Surf. Process. Landf., Volume 23 (1998) no. 6, pp. 527-544 | DOI
[52] The Water Erosion Prediction Project (WEPP) model, Landscape Erosion and Evolution Modeling (R. S. Harmon; W. W. Doe, eds.), Springer US, Boston, MA, 2001, pp. 145-199 | DOI
[53] The PESERA coarse scale erosion model for Europe. I. – Model rationale and implementation, Eur. J. Soil Sci., Volume 59 (2008) no. 6, pp. 1293-1306 | DOI
[54] The new assessment of soil loss by water erosion in Europe, Environ. Sci. Policy, Volume 54 (2015), pp. 438-447 | DOI
[55] LISEM: A single-event physically based hydrological and soil erosion model for drainage basins. I: Theory, input and outpout, Hydrol. Process., Volume 10 (1996) no. 8, pp. 1107-1117 | DOI
[56] Aggregate stability and assessment of soil crustability and erodibility: I. Theory and methodology, Eur. J. Soil Sci., Volume 47 (1996) no. 4, pp. 425-437 | DOI
[57] Using the USLE: chances, challenges and limitations of soil erosion modelling, Int. Soil Water Conserv. Res., Volume 7 (2019) no. 3, pp. 203-225 | DOI
[58] Representative elementary volume analysis of porous media using X-ray computed tomography, Powder Technol., Volume 200 (2010) no. 1, pp. 69-77 | DOI
[59] The limits of erosion modeling, Landscape Erosion and Evolution Modeling (R. S. Harmon; W. W. Doe, eds.), Springer US, Boston, MA, 2001, pp. 477-516 | DOI
[60] The legend of A. F. Shields, J. Hydraul. Eng., Volume 125 (1999) no. 4, 17211 | DOI
[61] Bed sediment entrainment by streamflow: State of the science, Sedimentology, Volume 66 (2019) no. 5, pp. 1449-1485 | DOI
[62] May the Shields theory be extended to cohesive and adhesive benthic sediments?, J. Geophys. Res. Oceans, Volume 112 (2007), C10020 | DOI
[63] Erosion threshold of sand-mud mixtures, Cont. Shelf Res., Volume 31 (2011) no. 10, supplement, p. S14-S25 (Proceedings of the 9th International Conference on Nearshore and Estuarine Cohesive Sediment Transport Processes) | DOI
[64] Introduction to the Physics of Cohesive Sediment in the Marine Environment, Developments in Sedimentology, 56, Elsevier, Amsterdam, 2004, pp. 121-159
[65] State-of-the-art review of continuum mechanics-based modelling of soil surface erosion, Archives Comput. Meth. Eng. (2024) | DOI
[66] Resistance of soils to concentrated flow erosion: a review, Earth-Sci. Rev., Volume 80 (2007) no. 1, pp. 75-109 | DOI
[67] Sediment transport and soil detachment on steep slopes: II. Sediment feedback relationship, Soil Sci. Soc. Am. J., Volume 73 (2009) no. 4, pp. 1298-1304 | DOI
[68] Transporting capacity of overland flow on plane and on irregular beds, Earth Surf. Process. Landf., Volume 11 (1986) no. 5, pp. 515-524 | DOI
[69] A conceptual framework for shear flow–induced erosion of soft cohesive sediment beds, J. Geophys. Res. Oceans, Volume 117 (2012) no. C10, 10020 | DOI
[70] A unified erosion formulation for fine sediments, Mar. Geol., Volume 179 (2001) no. 1, pp. 9-23 | DOI
[71] Dimensionless erosion laws for cohesive sediment, J. Hydraul. Eng., Volume 142 (2016) no. 2, 04015047 | DOI
[72] Stochastic components in the gully erosion modelling, Catena, Volume 63 (2005) no. 2, pp. 299-317 (Gully Erosion: A Global Issue) | DOI
[73] A stochastic formulation for erosion of cohesive sediments, J. Geophys. Res. Oceans, Volume 115 (2010), C01005 | DOI
[74] Erosion of Geomaterials, ISTE Ltd and John Wiley & Sons Inc, London, Hoboken, 2012 | DOI
[75] Apparatus, test procedures, and analytical methods to measure soil erodibility in situ, Appl. Eng. Agric., Volume 20 (2004) no. 4, pp. 455-462 | DOI
[76] Using an improved jet-erosion test to study the influence of soil parameters on the erosion of a silty soil, J. Hydraul. Eng., Volume 143 (2017) no. 8, 04017018 | DOI
[77] Quantification of erosion rates for undisturbed contaminated cohesive sediment cores by image analysis, Hydrobiologia, Volume 494 (2003) no. 1–3, pp. 271-276 (9th International Symposium on the Interactions between Sediments and Water, Banff, Canada, May 05–10, 2002) | DOI
[78] Automated sediment erosion testing system using digital imaging, J. Hydraul. Eng., Volume 130 (2004) no. 8, pp. 771-782 | DOI
[79] Experimental investigations of the bed evolution in wave flumes: performance of 2D and 3D optical systems, Coast. Engng, Volume 58 (2011) no. 7, pp. 606-622 | DOI
[80] Digital close range photogrammetry for measurement of soil erosion, Photogramm. Rec., Volume 20 (2005) no. 109, pp. 69-87 | DOI
[81] Bedload transport by a vertical jet impinging upon sediments, Phys. Fluids, Volume 26 (2014) no. 3, 035103 | DOI
[82] Concentrated leak erosion, Erosion in Geomechanics Applied to Dams and Levees, John Wiley & Sons, Ltd, London, Hoboken, 2013, pp. 271-341 | DOI
[83] Fluctuations of turbulent bed shear stress, J. Eng. Mech., Volume 129 (2003) no. 1, pp. 126-130 | DOI
[84] Statistical properties of wall shear stress fluctuations in turbulent channel flows, Intl J. Heat Fluid Flow, Volume 37 (2012), pp. 1-8 | DOI
[85] Pore-scale flow measurements at the interface between a sandy layer and a model porous medium: application to statistical modeling of contact erosion, J. Hydraul. Eng.-ASCE, Volume 139 (2013) no. 1, pp. 1-11 | DOI
[86] Muddy sediment erosion: insights from field studies, J. Hydraul. Eng., Volume 135 (2009) no. 2, pp. 73-87 | DOI
[87] Near-wall hot-wire measurements. Part II: turbulence time scale, convective velocity and spectra in the viscous sublayer, Exp. Fluids, Volume 31 (2001) no. 5, pp. 494-505 | DOI
[88] PIV measurements in the bottom boundary layer of the coastal ocean, Exp. Fluids, Volume 33 (2002) no. 6, pp. 962-971 | DOI
[89] A ferrofluid-based sensor to measure bottom shear stresses under currents and waves, J. Hydraul. Res., Volume 56 (2018) no. 5, pp. 630-647 | DOI
[90] Boundary-Layer Theory, Springer-Verlag, Berlin, Heidelberg, 2000 | DOI
[91] Wall-bounded flows, Springer Handbook of Experimental Fluid Mechanics (C. Tropea; A. L. Yarin; J. F. Foss, eds.), Springer, Berlin, Heidelberg, 2007, pp. 871-907 | DOI
[92] Calibration of the Preston tube and limitations on its use in pressure gradients, J. Fluid Mech., Volume 23 (1965) no. 1, pp. 185-208 | DOI
[93] Measurement of local bed shear stress in streams using a Preston-static tube, Limnol. Oceanogr., Volume 46 (2001) no. 8, pp. 2080-2087 | DOI
[94] The surface hot wire as a means of measuring mean and fluctuating wall shear stress, Exp. Fluids, Volume 31 (2002) no. 3, pp. 294-301 | DOI
[95] Modern developments in shear-stress measurement, Prog. Aerosp. Sci., Volume 38 (2002) no. 6, pp. 515-570 | DOI
[96] Wall-drag measurements of smooth- and rough-wall turbulent boundary layers using a floating element, Exp. Fluids, Volume 57 (2016), 90 | DOI
[97] On the use of bioluminescence for estimating shear stresses over a rippled seabed, Meccanica, Volume 45 (2010), pp. 881-895 | DOI
[98] Erosion and deposition of cohesive soils, J. Hydraul. Div.-ASCE, Volume 91 (1965) no. 1, pp. 105-139 | DOI
[99] Erosion rates of cohesive soils, J. Hydraul. Div.-ASCE, Volume 104 (1978) no. 2, pp. 279-283 | DOI
[100] Erosion of soft cohesive sediment deposits, J. Hydraul. Eng.-ASCE, Volume 111 (1985) no. 10, pp. 1308-1326 | DOI
[101] Effects of bed structure on erosion of cohesive sediments, J. Hydraul. Eng., Volume 125 (1999) no. 12, pp. 1297-1301 | DOI
[102] Energy-based method for providing soil surface erodibility rankings, J. Geotech. Geoenviron. Eng., Volume 137 (2011) no. 12, pp. 1290-1293 | DOI
[103] Model for surface erosion of cohesive soils, J. Hydraul. Eng., Volume 138 (2012) no. 7, pp. 581-590 | DOI
[104] Development of a simplified theoretical model to determine erodibility of compacted soil in hole erosion test based on fluid energy transformation, Acta Geotech., Volume 18 (2023) no. 10, pp. 5441-5455 | DOI
[105] Do critical stresses for incipient motion and erosion really exist?, J. Hydraul. Eng.-ASCE, Volume 113 (1987) no. 3, pp. 370-385 | DOI
[106] Le Rhône et les rivières à lit affouillable, Annales des Ponts et Chaussées, Volume 18 (1879), pp. 141-195
[107] Fluvial erosion/transport equation of landscape evolution models revisited, J. Geophys. Res. Earth Surf., Volume 114 (2009), F03007 | DOI
[108] Working with natural cohesive sediments, J. Hydraul. Eng., Volume 128 (2002) no. 1, pp. 2-8 | DOI
[109] The scaling law in the hole erosion test with a constant pressure drop, Int. J. Numer. Anal. Meth. Geomech., Volume 32 (2008) no. 13, pp. 1573-1595 | DOI
[110] WEPP - soil erodibility experiments for rangeland and cropland soils, J. Soil Water Conserv., Volume 46 (1991) no. 1, pp. 39-44
[111] Erosion function apparatus for scour rate predictions, J. Geotech. Geoenviron. Eng., Volume 127 (2001) no. 2, pp. 105-113 | DOI
[112] Soil detachment by shallow flow, Trans. ASAE, Volume 45 (2004) no. 2, pp. 351-357 | DOI
[113] Improvement of hole erosion test and results on reference soils, J. Geotech. Geoenviron. Eng., Volume 139 (2013) no. 2, pp. 330-339 | DOI
[114] Erosion of mud/sand mixtures, Coast. Engng, Volume 29 (1996) no. 1, pp. 1-25 | DOI
[115] Erodibility of cohesive sediment: the importance of sediment properties, Earth-Sci. Rev., Volume 105 (2011) no. 3, pp. 101-120 | DOI
[116] Probabilistic models for the erosion rate in embankments and reliability analysis of earth dams, Reliab. Eng. Syst. Saf., Volume 181 (2019), pp. 142-155 | DOI
[117] Predicting soil erodibility using electrical resistivity tomography, J. Geotech. Geoenviron. Eng., Volume 144 (2018) no. 4, 04018012 | DOI
[118] Erosion rate of compacted NA-Montmorillonite soils, J. Geotech. Eng., Volume 114 (1988) no. 3, pp. 296-305 | DOI
[119] Sea Carousel—A benthic, annular flume, Estuar. Coast. Shelf Sci., Volume 34 (1992) no. 6, pp. 557-577 | DOI
[120] Vims Sea Carousel: a field instrument for studying sediment transport, Mar. Geol., Volume 115 (1993) no. 3, pp. 271-287 | DOI
[121] Measurements of erosion of undisturbed bottom sediments with depth, J. Hydraul. Eng., Volume 122 (1996) no. 6, pp. 316-324 | DOI
[122] In Situ Erosion Flume (ISEF): determination of bed-shear stress and erosion of a kaolinite bed, J. Sea Res., Volume 39 (1998) no. 3, pp. 243-253 | DOI
[123] Flume measurements of sediment erodibility in boston harbor, J. Hydraul. Eng., Volume 125 (1999) no. 10, pp. 998-1005 | DOI
[124] A comparison and measurement standardisation of four in situ devices for determining the erosion shear stress of intertidal sediments, Cont. Shelf Res., Volume 20 (2000) no. 10, pp. 1397-1418 | DOI
[125] Effects of bed material properties on cohesive sediment erosion, Mar. Geol., Volume 207 (2004) no. 1, pp. 83-93 | DOI
[126] Chapter 11 Erodibility of natural sediments: experiments on sand/mud mixtures from laboratory and field erosion tests, Sediment and Ecohydraulics (T. Kusuda; H. Yamanishi; J. Spearman; J. Z. Gailani, eds.) (Proceedings in Marine Science), Volume 9, Elsevier, Amsterdam, 2008, pp. 137-153 | DOI
[127] Estimation and measurement of bed material shear stresses in erosion rate testing devices, J. Hydraul. Eng., Volume 138 (2012) no. 11, pp. 990-994 | DOI
[128] The Sediment Erosion Rate Flume (SERF): a new testing device for measuring soil erosion rate and shear stress, Geotech. Test. J., Volume 35 (2012) no. 4, pp. 649-659 | DOI
[129] Stability analysis of semicohesive streambanks with CONCEPTS: coupling field and laboratory investigations to quantify the onset of fluvial erosion and mass failure, J. Hydraul. Eng., Volume 140 (2014) no. 9, 04014041 | DOI
[130] Efficient automated laboratory testing of erosion resistance forfine-grained soils, Proceedings of the 10th International Conference on Scour and Erosion, Arlington, VA, USA, Volume 147, 2021
[131] Review of a semi-empirical modelling approach for cohesive sediment transport in river systems, Water, Volume 14 (2022) no. 2, 256 | DOI
[132] Erosion behavior of sand-silt mixtures: revisiting the erosion threshold, Water Resour. Res., Volume 58 (2022) no. 9, e2021WR031788 | DOI
[133] Pinhole test for identifying dispersive soils, J. Geotech. Eng. Div., Volume 102 (1976) no. 1, pp. 69-85 | DOI
[134] Cracking, leakage, and erosion of earth dam materials, J. Geotech. Eng. Div., Volume 106 (1980) no. 2, pp. 117-135 | DOI
[135] Erosivity of natural intact structured clay: evaluation, Can. Geotech. J., Volume 22 (1985) no. 4, pp. 508-517 | DOI
[136] Comparison of internal and surface erosion using flow pump tests on a sand-kaolinite mixture, Geotech. Test. J., Volume 23 (2000) no. 1, pp. 116-122 | DOI
[137] Investigation of rate of erosion of soils in embankment dams, J. Geotech. Geoenviron. Eng., Volume 130 (2004) no. 4, pp. 373-380 | DOI
[138] Laboratory tests on the rate of piping erosion of soils in embankment dams, Geotech. Test. J., Volume 27 (2004) no. 3, pp. 295-303 | DOI
[139] Investigating concentrated leak erosion behaviour of cohesive soils by performing hole erosion tests, Eur. J. Environ. Civil Eng., Volume 16 (2012) no. 1, pp. 43-58 | DOI
[140] The hole erosion test: a comparison of interpretation methods, Geotech. Test. J., Volume 40 (2017) no. 3, pp. 494-505 | DOI
[141] Measurement of pressure in viewable hole erosion test, Can. Geotech. J., Volume 55 (2018) no. 10, pp. 1502-1509 | DOI
[142] Experimental evaluation of internal erosion using groove erosion test, Int. J. Geomech., Volume 22 (2022) no. 6, 04022056 | DOI
[143] Experiments on the scour resistance of cohesive sediments, J. Geophys. Res. (1896–1977), Volume 67 (1962) no. 4, pp. 1437-1446 | DOI
[144] Erosion of loose beds by submerged circular impinging vertical turbulent jets, J. Hydraul. Res., Volume 34 (1996) no. 1, pp. 19-33 | DOI
[145] Erodibility of cohesive streambeds in the loess area of the midwestern USA, Hydrol. Process., Volume 15 (2001) no. 1, pp. 23-38 | DOI
[146] Borehole erosion test, J. Geotech. Geoenviron. Eng., Volume 143 (2017) no. 8, 04017037 | DOI
[147] Scouring by submerged steady water jet vertically impinging on a cohesive bed, Ocean Eng., Volume 196 (2020), 106781 | DOI
[148] Pore and eroding fluid influences on surface erosion on soil, J. Geotech. Eng. Div., Volume 101 (1975) no. 1, pp. 51-66 | DOI
[149] An improved rotating cylinder technique for quantitative measurements of the scour resistance of clays, Can. Geotech. J., Volume 23 (1986) no. 1, pp. 83-87 | DOI
[150] An improved rotating cylinder test design for laboratory measurement of erosion in clayey soils, Geotech. Test. J., Volume 32 (2009) no. 3, pp. 232-238 | DOI
[151] The rotating erosion testing apparatus (reta): a laboratory device for measuring erosion rates versus shear stresses of rock and cohesive materials, Geotech. Test. J., Volume 35 (2012) no. 4, pp. 641-648 | DOI
[152] Erosion threshold of saturated natural cohesive sediments: modeling and experiments, Water Resour. Res., Volume 44 (2008), W11434 | DOI
[153] Rotating circular flume, J. Hydraul. Eng., Volume 119 (1993) no. 6, pp. 758-767 | DOI
[154] Lessons learned using laboratory JET method to measure soil erodibility of compacted soils, Appl. Eng. Agric., Volume 23 (2007) no. 3, pp. 305-312 | DOI
[155] Deriving parameters of a fundamental detachment model for cohesive soils from flume and jet erosion tests, Trans. ASABE, Volume 56 (2013), pp. 489-504 | DOI
[156] et al. Perspectives on the Jet Erosion Test (JET): lessons learned, challenges and opportunities in quantifying cohesive soil erodibility, ASABE Papers, Volume 65 (2022) no. 2, pp. 197-207 | DOI
[157] Improvements in test procedure and data reduction for the Borehole Erosion Test, Geotech. Test. J., Volume 46 (2023) no. 2, pp. 295-315 | DOI
[158] Jet-induced cratering of a granular surface with application to lunar spaceports, J. Aerosp. Eng., Volume 22 (2009) no. 1, pp. 24-32 | DOI
[159] Two-fluid modeling of cratering in a particle bed by a subsonic turbulent jet, Powder Technol., Volume 318 (2017), pp. 68-82 | DOI
[160] Experimental investigations of crater formation on granular bed subjected to an air-jet impingement, Phys. Fluids, Volume 32 (2020), 053309 | DOI
[161] Impinging circular turbulent jets, J. Hudraul. Div.-ASCE, Volume 100 (1974) no. HY10, pp. 1313-1328 | DOI
[162] Relevance of free jet model for soil erosion by impinging jets, J. Hydraul. Eng., Volume 146 (2020) no. 1, 04019047 | DOI
[163] Manual of Soil Laboratory Testing, Third Edition: Volume Two: Permeability, Shear Strength and Compressibility Tests, Whittles Publishing, Caithness, 2011
[164] A device to examine the in situ response of intertidal cohesive sediment deposits to fluid shear, Cont. Shelf Res., Volume 15 (1995) no. 15, pp. 1945-1954 | DOI
[165] Measurements of sediment erosion and transport with the adjustable shear stress erosion and transport flume, J. Hydraul. Eng., Volume 129 (2003) no. 11, pp. 862-871 | DOI
[166] Measuring the in situ erosion shear stress of intertidal sediments with the Cohesive Strength Meter (CSM), Estuar. Coast. Shelf Sci., Volume 49 (1999) no. 2, pp. 281-294 | DOI
[167] Physical modeling of overtopping erosion and breach formation of cohesive embankments, Trans. ASAE, Volume 48 (2005) no. 5, pp. 1783-1794 | DOI
[168] Full-scale testing of levee resiliency during wave overtopping, Proceedings from Annual Innovative Dam and Levee Design and Construction for Sustainable Water Management, Elsevier, 2012, pp. 721-735
[169] Large-scale experiment and numerical modeling of a riverine levee breach, J. Hydraul. Eng., Volume 140 (2014) no. 9, 04014039 | DOI
[170] Overflowing tests at the Polish DredgDikes research dike – stability of the dike surface against erosion, Eng. Struct. Technol., Volume 6 (2014) no. 4, pp. 159-168 | DOI
[171] Full-scale flume experiments to analyze the surface erosion resistance of dike embankments made of dredged materials, J. Water. Port, Coast. Ocean Eng., Volume 143 (2017) no. 3, 04017001 | DOI
[172] Quantifying the erosion resistance of dikes with the overflowing simulator, Proceedings of the 3rd International Conference on Protection against Overtopping, 6–8 June 2018, Grange-over-Sands, United Kingdom, 2018
[173] et al. Field testing of the overflow erosion of rhone river levees, Proceedings of the 4th International Seminar on Dam Protections against Overtopping, 30 Nov 2 Dec 2012, Madrid, Spain, 2023 | DOI
[174] Some elements of comparison between two laboratory devices for soil erosion testing, Proceedings of the 6th International Conference on Scour and Erosion 27–31 August 2012, Paris, France, 2012
[175] Soil surface erosion: local law and influence of soil and flow properties on the erosion resistance of hydraulic structures, PhD Thesis, Aix Marseille University (2023)
[176] Erosion charts for selected geomaterials, J. Geotech. Geoenviron. Eng., Volume 143 (2017) no. 10, 04017072 | DOI
[177] Determining erosion indices of cohesive soils with the hole erosion test and jet erosion test, 2008 https://api.semanticscholar.org/CorpusID:52839129 (Report DSO-08-05)
[178] Impact of lime, cement, and clay treatments on the internal erosion of compacted soils, J. Mater. Civil Eng., Volume 28 (2016) no. 9, 04016071 | DOI
[179] A modified Hole Erosion test (HET-P) to study erosion characteristics of soil, PhD Thesis, University of British Columbia (2011)
[180] Experimental investigation of erosion in variably saturated clay soils, PhD Thesis, School of Civil and Environmental Engineering, The University of New South Wales (2006)
[181] A modified hole erosion test (HET-P) device, Geotech. Test. J., Volume 35 (2012), 104336 | DOI
[182] Erodibility parameters derived from jet and flume erosion tests on root-permeated soils, J. Contemp. Water Res. Educ., Volume 160 (2017), pp. 119-131 | DOI
[183] Variability of fluvial erodibility parameters for streambanks on a watershed scale, Geomorphology, Volume 231 (2015), pp. 281-291 | DOI
[184] Critical assessment of jet erosion test methodologies for cohesive soil and sediment, Geomorphology, Volume 295 (2017), pp. 529-536 | DOI
[185] Relationship Between Erodibility and Properties of Soils, The National Academies Press, Washington, DC, 2019
[186] State of the art on the likelihood of internal erosion of dams and levees by means of testing, Erosion in Geomechanics Applied to Dams and Levees (S. Bonelli, ed.) (Civil Engineering and Geomechanics Series), John Wiley & Sons, Ltd, 2013, pp. 1-99 | DOI
[187] Piping flow erosion in water retaining structures, Int. J. Hydrop. Dams, Volume 18 (2011) no. 3, pp. 94-99
[188] Microbially induced carbonate precipitation for seepage-induced internal erosion control in sand–clay mixtures, J. Geotech. Geoenviron. Eng., Volume 143 (2017) no. 3, 04016100 | DOI
[189] Modelling the erosion rate of chemically stabilized soil incorporating tensile force – deformation characteristics, Can. Geotech. J., Volume 46 (2009) no. 1, pp. 57-68 | DOI
[190] Predicting the erosion rate of chemically treated soil using a process simulation apparatus for internal crack erosion, J. Geotech. Geoenviron. Eng., Volume 134 (2008) no. 6, pp. 837-844 | DOI
[191] Internal erosion resistance of soils treated with lime: an evolutive benefit, Scour and Erosion: Proceedings of the 7th International Conference on Scour and Erosion, Perth, Australia, 2–4 December 2014 (L. Cheng; S. Draper; H. An, eds.), CRC Press, London, 2014, pp. 73-80 | DOI
[192] Lime treatment: evaluation for use in dike applications in the Netherlands, J. Mater. Civil Eng., Volume 35 (2023) no. 3, 04022465 | DOI
[193] Relationship between the erosion properties of soils and other parameters, Erosion in Geomechanics Applied to Dams and Levees (S. Bonelli, ed.) (Civil Engineering and Geomechanics Series), John Wiley & Sons, Ltd, 2013, pp. 343-381 | DOI
[194] Erosion resistance of cohesive soils, J. Hydraul. Res., Volume 46 (2008) no. 6, pp. 777-787 | DOI
[195] Investigation of interface erosion rate by Jet Erosion Test and statistical analysis, Eur. J. Environ. Civil Eng., Volume 15 (2011) no. 8, pp. 1167-1185 | DOI
[196] An experimental study on the effects of physical, mechanical, and electrochemical properties of natural cohesive soils on critical shear stress and erosion rate, Int. J. Sediment Res., Volume 31 (2016) no. 1, pp. 1-15 | DOI
[197] Relationship between soil erodibility and soil properties, J. Geotech. Geoenviron. Eng., Volume 149 (2023) no. 1, 04022121 | DOI
[198] Relationship Between Erodibility and Properties of Soils, The National Academies Press, Washington, DC, 2019
[199] Critical shear stress for erosion of sand-mud mixtures and pure mud, Front. Marine Sci., Volume 8 (2021), 713039 | DOI
[200] Unified formula for critical shear stress for erosion of sand, mud, and sand–mud mixtures, J. Hydraul. Eng., Volume 144 (2018) no. 8, 04018046 | DOI
[201] Generalized shields criterion for weakly cohesive granular materials, Phys. Rev. Fluids, Volume 5 (2020), 034308 | DOI
[202] Erosion of cohesive sediments as a rate process, Ocean Engng, Volume 7 (1980) no. 4, pp. 539-551 | DOI
[203] Water temperature, pH, and road salt impacts on the fluvial erosion of cohesive streambanks, Water, Volume 10 (2018) no. 3, 302 | DOI
[204] Fluvial erosion rate of cohesive streambanks is directly related to the difference in soil and water temperatures, J. Environ. Qual., Volume 48 (2019) no. 6, pp. 1741-1748 | DOI
[205] Effect of sediment pH on resuspension of Kaolinite sediments, J. Environ. Eng., Volume 127 (2001) no. 6, pp. 531-538 | DOI
[206] Granular Media: Between Fluid and Solid, Cambridge University Press, New York, 2013 | DOI
[207] An assessment of the erodibility of Holocene lithounits comprising streambanks in northeastern Kansas, USA, Geomorphology, Volume 213 (2014), pp. 116-127 | DOI
[208] Determining erodibility, critical shear stress, and allowable discharge estimates for cohesive channels: case study in the powder river basin of Wyoming, J. Hydraul. Eng., Volume 134 (2008) no. 12, pp. 1677-1687 | DOI
[209] Variability of erodibility parameters from laboratory mini jet erosion tests, J. Hydrol. Eng., Volume 21 (2016) no. 10, 04016030 | DOI
[210] Strength of undisturbed versus reconstituted silt and silty sand specimens, J. Geotech. Geoenviron. Eng., Volume 126 (2000) no. 7, pp. 606-617 | DOI
[211] Detachment of undisturbed soil by shallow flow, Soil Sci. Soc. Am. J., Volume 67 (2003) no. 3, pp. 713-719 | DOI
[212] A comparative analysis of interface erosion tests, Nat. Hazards, Volume 67 (2013) no. 2, pp. 937-950 | DOI
[213] Comparison of erodibility parameters for cohesive streambank soils between in situ jet test device and laboratory conduit flume, J. Hydraul. Eng., Volume 148 (2022) no. 1, 04021055 | DOI
[214] A systematic review of strategies for identifying and stabilizing dispersive clay soils for sustainable infrastructure, Soil Tillage Res., Volume 239 (2024), 106036 | DOI
[215] Comparison of computational fluid dynamic simulations with experimental jet erosion tests results, J. Hydraul. Eng., Volume 140 (2014) no. 5, 04014006 | DOI
[216] Numerical modelling of concentrated leak erosion during Hole Erosion Tests, Acta Geotech., Volume 10 (2015) no. 3, pp. 319-332 | DOI
[217] Physics of soil erosion at the microscale, EPJ Web Conf., Volume 140 (2017), 08014 | DOI
[218] Numerical modelling of backward front propagation in piping erosion by DEM-LBM coupling, Eur. J. Environ. Civil Eng., Volume 21 (2017) no. 7–8, pp. 960-987 | DOI
[219] A parallel GPU-based computational framework for the micromechanical analysis of geotechnical and erosion problems, Comput. Geotech., Volume 120 (2020), 103404 | DOI
[220] On the erosion of cohesive granular soils by a submerged jet: a numerical approach, Granul. Matt., Volume 25 (2023) no. 1, 8 | DOI
[221] Levee erosion by overtopping in new Orleans during the Katrina Hurricane, J. Geotech. Geoenviron. Eng., Volume 134 (2008) no. 5, pp. 618-632 | DOI
Cité par Sources :
Commentaires - Politique