[Rayonnement de Hawking par l’horizon analogue de spin dans un condensat de Bose–Einstein à deux composantes]
Nous étudions théoriquement l’émission de Hawking stimulée et spontanée à partir d’un horizon analogue pour les modes de spin dans un condensat de Bose–Einstein à deux composants, avec et sans couplage cohérent entre les deux composants. Nous mettons en évidence les avantages conceptuels et pratiques que ces systèmes offrent pour l’observation expérimentale du phénomène, à savoir la possibilité d’avoir des excitations élémentaires massives et l’accessibilité expérimentale des différentes quadratures des excitations de spin. Plus specifiquement, nous allons au-delà des régimes relativistes précédemment abordés dans la littérature, et identifions des observables qui montrent une signature du processus de Hawking, ainsi que des caractéristiques supplémentaires associées à la nature massive des modes, telles que des ondulations. Les calculs semi-analytiques des propriétés de reflexion et transmission de l’horizon et des fonctions de corrélation à deux points du rayonnement émis dans un cadre stationnaire idéal sont confirmés par des simulations numériques dépendantes du temps basées sur les théories de Gross–Pitaevskii et de Bogoliubov.
We theoretically study stimulated and spontaneous Hawking emission from an analog horizon for spin modes in a two-component Bose–Einstein condensate, both with and without a coherent coupling between the two components. We highlight the conceptual and practical advantages that these systems offer to the experimental observation of the phenomenon, namely the possibility of working with massive elementary excitations and the experimental accessibility of the different quadratures of the spin excitations. In particular, we go beyond the relativistic regimes previously addressed in the literature, and identify various observables that show a signature of the Hawking process, as well as additional features associated with the massive nature of the modes, such as undulations. Semi-analytical calculations of the scattering properties of the horizon and of two-point correlation functions of the emitted radiation in an ideal stationary setup are supported by time-dependent numerical simulations based on Gross–Pitaevskii and Bogoliubov theory.
Révisé le :
Accepté le :
Première publication :
Mots-clés : Rayonnement de Hawking, Gravité analogue, Condensats de Bose–Einstein à deux composantes
Anna Berti 1 ; Lennart Fernandes 2, 3 ; Salvatore Butera 4 ; Alessio Recati 1 ; Michiel Wouters 3 ; Iacopo Carusotto 1
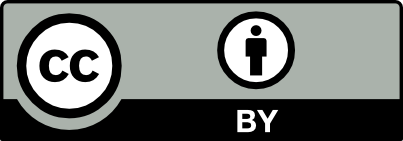
@article{CRPHYS_2024__25_S2_A12_0, author = {Anna Berti and Lennart Fernandes and Salvatore Butera and Alessio ~Recati and Michiel Wouters and Iacopo Carusotto}, title = {Analog {Hawking} radiation from a spin-sonic horizon in a two-component {Bose{\textendash}Einstein} condensate}, journal = {Comptes Rendus. Physique}, publisher = {Acad\'emie des sciences, Paris}, year = {2024}, doi = {10.5802/crphys.226}, language = {en}, note = {Online first}, }
TY - JOUR AU - Anna Berti AU - Lennart Fernandes AU - Salvatore Butera AU - Alessio Recati AU - Michiel Wouters AU - Iacopo Carusotto TI - Analog Hawking radiation from a spin-sonic horizon in a two-component Bose–Einstein condensate JO - Comptes Rendus. Physique PY - 2024 PB - Académie des sciences, Paris N1 - Online first DO - 10.5802/crphys.226 LA - en ID - CRPHYS_2024__25_S2_A12_0 ER -
%0 Journal Article %A Anna Berti %A Lennart Fernandes %A Salvatore Butera %A Alessio Recati %A Michiel Wouters %A Iacopo Carusotto %T Analog Hawking radiation from a spin-sonic horizon in a two-component Bose–Einstein condensate %J Comptes Rendus. Physique %D 2024 %I Académie des sciences, Paris %Z Online first %R 10.5802/crphys.226 %G en %F CRPHYS_2024__25_S2_A12_0
Anna Berti; Lennart Fernandes; Salvatore Butera; Alessio Recati; Michiel Wouters; Iacopo Carusotto. Analog Hawking radiation from a spin-sonic horizon in a two-component Bose–Einstein condensate. Comptes Rendus. Physique, Online first (2024), pp. 1-36. doi : 10.5802/crphys.226.
[1] Black hole explosions?, Nature, Volume 248 (1974) no. 5443, pp. 30-31 | DOI
[2] Particle creation by black holes, Commun. Math. Phys., Volume 43 (1975), pp. 199-220 | DOI
[3] The temperature of the cosmic microwave background, Astrophys. J., Volume 707 (2009), pp. 916-920 | DOI
[4] Black Hole Physics, Fundamental Theories of Physics, 96, Springer Netherlands, Dordrecht, 1998 | DOI
[5] Hawking radiation without black hole entropy, Phys. Rev. Lett., Volume 80 (1998) no. 16, pp. 3436-3439 | DOI
[6] Analogue gravity, Living Rev. Relativ., Volume 14 (2011) no. 1, 3 | DOI
[7] Observation of negative-frequency waves in a water tank: a classical analogue to the Hawking effect?, New J. Phys., Volume 10 (2008) no. 5, 053015 | DOI
[8] Horizon effects with surface waves on moving water, New J. Phys., Volume 12 (2010) no. 9, 095018 | DOI
[9] Rotational superradiant scattering in a vortex flow, Nat. Phys., Volume 13 (2017) no. 9, pp. 833-836 | DOI
[10] et al. Acoustic black hole in a stationary hydrodynamic flow of microcavity polaritons, Phys. Rev. Lett., Volume 114 (2015) no. 3, 036402 | DOI
[11] Analog Hawking radiation from an acoustic black hole in a flowing polariton superfluid, Phys. Rev. B, Volume 86 (2012) no. 14, 144505 | DOI
[12] Fiber-optical analog of the event horizon, Science, Volume 319 (2008) no. 5868, pp. 1367-1370 | DOI
[13] et al. Hawking radiation from ultrashort laser pulse filaments, Phys. Rev. Lett., Volume 105 (2010) no. 20, 203901 | DOI
[14] Realization of a sonic black hole analog in a Bose–Einstein condensate, Phys. Rev. Lett., Volume 105 (2010) no. 24, 240401 | DOI
[15] Black-hole radiation in Bose–Einstein condensates, Phys. Rev. A, Volume 80 (2009) no. 4, 043601 | DOI
[16] Bogoliubov theory of acoustic Hawking radiation in Bose–Einstein condensates, Phys. Rev. A, Volume 80 (2009) no. 4, 043603 | DOI
[17] Numerical observation of Hawking radiation from acoustic black holes in atomic Bose–Einstein condensates, New J. Phys., Volume 10 (2008) no. 10, 103001 | DOI
[18] Quantum fluctuations around black hole horizons in Bose–Einstein condensates, Phys. Rev. A, Volume 85 (2012) no. 1, 013621
[19] Observation of quantum Hawking radiation and its entanglement in an analogue black hole, Nat. Phys., Volume 12 (2016) no. 10, pp. 959-965 | DOI
[20] Observation of thermal Hawking radiation and its temperature in an analogue black hole, Nature, Volume 569 (2019) no. 7758, pp. 688-691 | DOI
[21] Observation of stationary spontaneous Hawking radiation and the time evolution of an analogue black hole, Nat. Phys., Volume 17 (2021) no. 3, pp. 362-367 | DOI
[22] A study of coherently coupled two-component Bose–Einstein condensates, Eur. Phys. J. D, Volume 67 (2013) no. 7, 148 | DOI
[23] Hawking radiation in a two-component Bose–Einstein condensate, Europhys. Lett., Volume 103 (2013) no. 6, 60001 | DOI
[24] Analogous Hawking radiation and quantum entanglement in two-component Bose–Einstein condensates: The gapped excitations, Phys. Rev. D, Volume 106 (2022) no. 4, 044016 | DOI
[25] Analogous Hawking radiation from gapped excitations in a transonic flow of binary Bose–Einstein condensates, Phys. Rev. D, Volume 107 (2023) no. 8, 084049 | DOI
[26] Quantum simulation of cosmic inflation in two-component Bose–Einstein condensates, Phys. Rev. A, Volume 70 (2004) no. 6, 063615 | DOI
[27] Observation of massless and massive collective excitations with Faraday patterns in a two-component superfluid, Phys. Rev. Lett., Volume 128 (2022) no. 21, 210401 | DOI
[28] Bose–Einstein Condensation and Superfluidity, International Series of Monographs on Physics, 164, Oxford University Press, Oxford, 2016 | DOI
[29] et al. Ferromagnetism in an extended coherently coupled atomic superfluid, Phys. Rev. X, Volume 13 (2023) no. 2, 021037 | DOI
[30] et al. Realization of Bose–Einstein condensates in lower dimensions, Phys. Rev. Lett., Volume 87 (2001) no. 13, 130402 | DOI
[31] Generation of dispersive shock waves by the flow of a Bose–Einstein condensate past a narrow obstacle, Phys. Rev. A, Volume 85 (2012) no. 3, 033603 | DOI
[32] Spatially varying interactions induced in ultra-cold atoms by optical Feshbach resonance, Phys. Lett. A, Volume 376 (2012) no. 5, pp. 729-732 | DOI
[33] Phonon background versus analog Hawking radiation in Bose–Einstein condensates, Phys. Rev. A, Volume 78 (2008) no. 2, 021601 | DOI
[34] Bragg scattering and the spin structure factor of two-component atomic gases, J. Phys. B: At. Mol. Opt. Phys., Volume 39 (2006) no. 10, p. S211-S219 | DOI
[35] Observation of magnetic solitons in two-component Bose–Einstein condensates, Phys. Rev. Lett., Volume 125 (2020) no. 3, 030401 | DOI
[36] Observation of two sound modes in a binary superfluid gas, Phys. Rev. A, Volume 101 (2020) no. 6, 061601 | DOI
[37] Hawking radiation of massive modes and undulations, Phys. Rev. D, Volume 86 (2012) no. 6, 064022 | DOI
[38] Departing from thermality of analogue Hawking radiation in a Bose–Einstein condensate, Phys. Rev. Lett., Volume 124 (2020) no. 6, 060401 | DOI
[39] Universality of low energy absorption cross sections for black holes, Phys. Rev. Lett., Volume 78 (1997) no. 3, pp. 417-419 | DOI
[40] Greybody factor for a scalar field coupling to Einstein’s tensor, Phys. Lett. B, Volume 691 (2010) no. 5, pp. 254-260 | DOI
[41] Greybody factors for nonminimally coupled scalar fields in Schwarzschild–de Sitter spacetime, Phys. Rev. D, Volume 87 (2013) no. 10, 104034 | DOI
[42] Gray-body factor and infrared divergences in 1D BEC acoustic black holes, Phys. Rev. D, Volume 90 (2014) no. 10, 104044 | DOI
[43] Acoustic white holes in flowing atomic Bose–Einstein condensates, New J. Phys., Volume 13 (2011) no. 2, 025007 | DOI
[44] Hawking radiation and the boomerang behavior of massive modes near a horizon, Phys. Rev. D, Volume 83 (2011) no. 10, 104028 | DOI
[45] Superradiance, 10, Springer Nature Switzerland AG, Cham, 2020 | DOI
[46] Understanding superradiant phenomena with synthetic vector potentials in atomic Bose–Einstein condensates, Phys. Rev. A, Volume 103 (2021) no. 4, 043309 | DOI
[47] Superradiant phenomena: Lessons from and for Bose–Einstein condensates, PhD thesis, University of Trento (2021)
[48] Analogue quantum simulation of the Hawking effect in a polariton superfluid, Eur. Phys. J. D, Volume 76 (2022) no. 8, 152 | DOI
[49] A time-splitting spectral method for computing dynamics of spinor F = 1 Bose–Einstein condensates, Int. J. Comp. Math., Volume 84 (2007) no. 6, pp. 925-944 | DOI
[50] Dynamical properties of Bose–Bose mixtures, PhD thesis, University of Trento (2016)
[51] Low-temperature Bose–Einstein condensates in time-dependent traps: Beyond the U(1) symmetry-breaking approach, Phys. Rev. A, Volume 57 (1998), pp. 3008-3021 | DOI
[52] Particle creation in the spin modes of a dynamically oscillating two-component Bose–Einstein condensate, Phys. Rev. D, Volume 104 (2021), 083503 | DOI
[53] Generation of finite difference formulas on arbitrarily spaced grids, Math. Comput., Volume 51 (1988) no. 184, pp. 699-706 | DOI
[54] Fluctuations in multicomponent quantum fluids, PhD thesis, University of Antwerp (2024)
Cité par Sources :
Commentaires - Politique