Nonlinear phononics is the phenomenon in which a coherent dynamics in a material along a set of phonons is launched after its infrared-active phonons are selectively excited using external light pulses. The microscopic mechanism underlying this phenomenon is the nonlinear coupling of the pumped infrared-active mode to other phonon modes present in a material. Nonlinear phonon couplings can cause finite time-averaged atomic displacements with or without broken crystal symmetries depending on the order, magnitude and sign of the nonlinearities. Such coherent lattice displacements along phonon coordinates can be used to control the physical properties of materials and even induce transient phases with lower symmetries. Light-control of materials via nonlinear phononics has become a practical reality due to the availability of intense mid-infrared lasers that can drive large-amplitude oscillations of the infrared-active phonons of materials. Mid-infrared pump induced insulator–metal transitions and spin and orbital order melting have been observed in pump–probe experiments. First principles based microscopic theory of nonlinear phononics has been developed, and it has been used to better understand how the lattice evolves after a mid-infrared pump excitation of infrared-active phonons. This theory has been used to predict light-induced switching of ferroelectric polarization as well as ferroelectricity in paraelectrics and ferromagnetism in antiferromagnets, which have been partially confirmed in recent experiments. This review summarizes the experimental and theoretical developments within this emerging field.
Publié le :
Alaska Subedi 1, 2
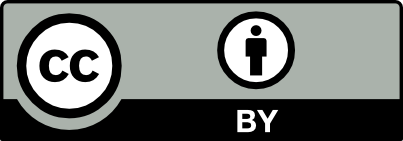
@article{CRPHYS_2021__22_S2_161_0, author = {Alaska Subedi}, title = {Light-control of materials via nonlinear phononics}, journal = {Comptes Rendus. Physique}, pages = {161--184}, publisher = {Acad\'emie des sciences, Paris}, volume = {22}, number = {S2}, year = {2021}, doi = {10.5802/crphys.44}, language = {en}, }
Alaska Subedi. Light-control of materials via nonlinear phononics. Comptes Rendus. Physique, Volume 22 (2021) no. S2, pp. 161-184. doi : 10.5802/crphys.44. https://comptes-rendus.academie-sciences.fr/physique/articles/10.5802/crphys.44/
[1] Laser selective chemistry — is it possible?, Phys. Today, Volume 33 (1980), pp. 27-33 | DOI
[2] Laser control of chemical reactions, Science, Volume 279 (1998) no. 5358, pp. 1875-1879 | DOI
[3] Coherent control of quantum dynamics: The dream is alive, Science, Volume 259 (1993), pp. 1581-1589 | DOI | MR | Zbl
[4] Energy redistribution in isolated molecules and the question of mode-selective laser chemistry revisited, J. Phys. Chem., Volume 88 (1984), pp. 5459-5465 | DOI
[5] Vibrational redistribution within excited electronic states of polyatomic molecules, Faraday Discuss. Chem. Soc., Volume 75 (1983), pp. 7-22 | DOI
[6] Vibrational energy flow in highly excited molecules: Role of intramolecular vibrational redistribution, J. Phys. Chem., Volume 100 (1996), pp. 12735-12756 | DOI
[7] Nonlinear two-dimensional vibrational spectroscopy of peptides, J. Phys. Condens. Matter, Volume 14 (2002) no. 39, R1035–R1062 | DOI
[8] Signatures of vibrational interactions in coherent two-dimensional infrared spectroscopy, Chem. Phys., Volume 266 (2001), pp. 213-230 | DOI
[9] Coherent 2D IR spectroscopy: Molecular structure and dynamics in solution, J. Phys. Chem., Volume 107 (2003), pp. 5258-5279 | DOI
[10] Vibrational multilevel quantum coherence due to anharmonic couplings in intermolecular hydrogen bonds, Phys. Rev. Lett., Volume 91 (2003), 197401 | DOI
[11] Ionic Raman effect. II. The first-order ionic Raman effect, Phys. Rev. B, Volume 3 (1971), pp. 2063-2075 | DOI
[12] Ionic Raman scattering and ionic frequency mixing, Phys. Status Solidi B, Volume 61 (1974) no. 2, pp. 493-502 | DOI
[13] Nonlinear phononics as an ultrafast route to lattice control, Nat. Phys., Volume 7 (2011), pp. 854-866 | DOI
[14] An effective magnetic field from optically driven phonons, Nat. Phys., Volume 13 (2016), pp. 132-136 | DOI
[15] Ultrafast strain engineering and coherent structural dynamics from resonantly driven optical phonons in LaAlO, NPJ Quant. Mater., Volume 5 (2020), 95 | DOI
[16] Ultrafast reversal of the ferroelectric polarization, Phys. Rev. Lett., Volume 118 (2017), 197601 | DOI
[17] Displacive lattice excitation through nonlinear phononics viewed by femtosecond X-ray diffraction, Solid State Commun., Volume 169 (2013), pp. 24-27 | DOI
[18] et al. Nonlinear lattice dynamics as a basis for enhanced superconductivity in YBaCuO, Nature (London), Volume 516 (2014), pp. 71-73 | DOI
[19] Proposal for ultrafast switching of ferroelectrics using midinfrared pulses, Phys. Rev. B, Volume 92 (2015), 214303 | DOI
[20] Control of the electronic phase of a manganite by mode-selective vibrational excitation, Nature (London), Volume 449 (2007), pp. 72-74 | DOI
[21] Nonlinear electron–phonon coupling in doped manganites, Phys. Rev. Lett., Volume 118 (2017), 247601 | DOI
[22] et al. Nonlinear electron–phonon coupling in doped manganites, Phys. Rev. Lett., Volume 108 (2012), 136801
[23] Ultrafast electronic phase transition in LaSrMnO by coherent vibrational excitation: Evidence for nonthermal melting of orbital order, Phys. Rev. Lett., Volume 101 (2008), 197404 | DOI
[24] et al. Driving magnetic order in a manganite by ultrafast lattice excitation, Phys. Rev. B, Volume 84 (2011), 241104(R) | DOI
[25] et al. Spatially resolved ultrafast magnetic dynamics initiated at a complex oxide heterointerface, Nat. Mater., Volume 14 (2015), pp. 883-888 | DOI
[26] Midinfrared-light-induced ferroelectricity in oxide paraelectrics via nonlinear phononics, Phys. Rev. B, Volume 95 (2017), 134113 | DOI
[27] Metastable ferroelectricity in optically strained SrTiO, Science, Volume 364 (2019), pp. 1075-1079 | DOI
[28] Terahertz field-induced ferroelectricity in quantum paraelectric SrTiO, Science, Volume 364 (2019), pp. 1079-1082 | DOI
[29] Light-induced superconductivity in a stripe-ordered cuprate, Science, Volume 331 (2011), pp. 189-191 | DOI
[30] Optically induced coherent transport far above Tc in underdoped YBaCuO, Phys. Rev. B, Volume 89 (2014), 184516 | DOI
[31] Optically enhanced coherent transport in YBaCuO by ultrafast redistribution of interlayer coupling, Nat. Mater., Volume 13 (2014), pp. 705-711 | DOI
[32] Possible light-induced superconductivity in KC at high temperature, Nature (London), Volume 530 (2016), pp. 461-464 | DOI
[33] Non-equilibrium control of complex solids by nonlinear phononics, Rep. Prog. Phys., Volume 79 (2016), 064503 | DOI
[34] Nonlinear light-matter interaction at terahertz frequencies, Adv. Opt. Photon. 8 (2016), pp. 401-464 | DOI
[35] Photo-induced superconductivity, Contemp. Phys., Volume 59 (2018), pp. 31-46 | DOI
[36] Collective coherent control: Synchronization of polarization in ferroelectric PbTiO by shaped THz fields, Phys. Rev. Lett., Volume 102 (2009), 247603
[37] Coherent phonon generation in time-dependent density functional theory, Phys. Rev. B, Volume 82 (2010), 155110 | DOI
[38] Theory of nonlinear phononics for coherent light control of solids, Phys. Rev. B, Volume 89 (2014), 220301(R) | DOI
[39] Ultrafast structure switching through nonlinear phononics, Phys. Rev. Lett., Volume 118 (2017), 054101 | DOI
[40] Breaking symmetry with light: Ultrafast ferroelectricity and magnetism from three-phonon coupling, Phys. Rev. B, Volume 97 (2018), 085145 | DOI
[41] Ultrafast control of magnetic interactions via light-driven phonons, Nat. Mater. (2021), pp. 1-5 | DOI
[42] Polarizing an antiferromagnet by optical engineering of the crystal field, Nat. Phys., Volume 16 (2020), pp. 937-941 | DOI
[43] Ultrafast band engineering and transient spin currents in antiferromagnetic oxides, Sci. Rep., Volume 6 (2016), 25121
[44] Phono-magnetic analogs to opto-magnetic effects, Phys. Rev. Res., Volume 2 (2020), 043035 | DOI
[45] Cavity control of nonlinear phononics (2019) (https://arxiv.org/abs/1912.00122)
[46] Parametric excitation of an optically silent Goldstone-like phonon mode, Phys. Rev. Lett., Volume 124 (2020), 117401 | DOI
[47] Mode-selective control of the crystal lattice, Acc. Chem. Res., Volume 48 (2015), pp. 380-387 | DOI
[48] Measuring non-equilibrium dynamics in complex solids with ultrashort X-ray pulses, Phil. Trans. R. Soc. A, Volume 377 (2019) no. 2145, 20170478 | DOI
[49] Matter manipulation with extreme terahertz light: Progress in the enabling THz technology, Phys. Rep., Volume 836-837 (2019), pp. 1-74 | DOI
[50] Optical study of PrCaMnO (x = 0.4) in a magnetic field: Variation of electronic structure with charge ordering and disordering phase transitions, Phys. Rev. B, Volume 59 (1999), pp. 7401-7408 | DOI
[51] Magnetic-field-induced metal-insulator phenomena in PrCaMnO with controlled charge-ordering instability, Phys. Rev. B, Volume 53 (1996), R1689–R1692 | DOI
[52] Photoinduced insulator-to-metal transition in a perovskite manganite, Phys. Rev. Lett., Volume 78 (1997), pp. 4257-4260 | DOI
[53] Current switching of resistive states in magnetoresistive manganites, Nature (London), Volume 388 (1997), pp. 50-52 | DOI
[54] Optical properties along the c-axis of YBaCuO, for x = 0.500.95 evolution of the pseudogap, Physica C, Volume 254 (1995) no. 3-4, pp. 265-280 | DOI
[55] Effects of intense optical phonon pumping on the structure and electronic properties of yttrium barium copper oxide, Phys. Rev. B, Volume 94 (2016), 134307 | DOI
[56] Infrared and electronic spectra of rare earth perovskites: Ortho-chromites, -manganites and -ferrites, Appl. Spectrosc., Volume 24 (1970), pp. 436-445
[57] Sum-frequency ionic Raman scattering, Phys. Rev. B, Volume 97 (2018), 174302 | DOI
[58] Terahertz-driven phonon upconversion in SrTiO, Nat. Phys., Volume 15 (2019), pp. 387-392 | DOI
[59] Dynamical multiferroicity, Phys. Rev. Mater., Volume 1 (2017), 014401
[60] Magnetophononics: Ultrafast spin control through the lattice, Phys. Rev. Mater., Volume 2 (2018), 064401
[61] Nonlinear phononic control and emergent magnetism in Mott insulating titanates, Phys. Rev. B, Volume 98 (2018), 024102
[62] Ultrafast optically induced ferromagnetic/anti-ferromagnetic phase transition in GdTiO from first principles, NPJ Quant. Mater., Volume 3 (2018), 15 | DOI
Cité par Sources :
Commentaires - Politique